Abstract
Magnetic resonance (MR) imaging is the most complex imaging technology available to clinicians. Whereas most imaging technologies depict differences in one, or occasionally two, tissue characteristics, MR imaging has five tissue variables—spin density, T1 and T2 relaxation times and flow and spectral shifts—from which to construct its images. These variables can be combined in various ways by selecting pulse sequences and pulse times to emphasize any desired combination of tissue characteristics in the image. This selection is determined by the user of the MR system before imaging data are collected. If the selection is not optimal, the imaging process must be repeated at a cost of time and resources.
The optimal selection of MR imaging procedures and the proper interpretation of the resultant images require a thorough understanding of the basic principles of MR imaging. Included in this understanding should be at least the rudiments of how an MR imaging signal is produced and why it decays with time; the significance of relaxation constants; the principles of scanning methods such as saturation recovery, inversion recovery and spin echo; how data obtained by these methods are used to form an image, and how the imaging data are complied by multi-slice and volumetric processes. In selecting an MR imaging unit, information about different magnet designs (resistive, superconductive and permanent) is useful. Although no bioeffects are thought to be associated with an MR imaging examination, some knowledge of the attempts to identify bioeffects is helpful in alleviating concern in patients.
Full text
PDF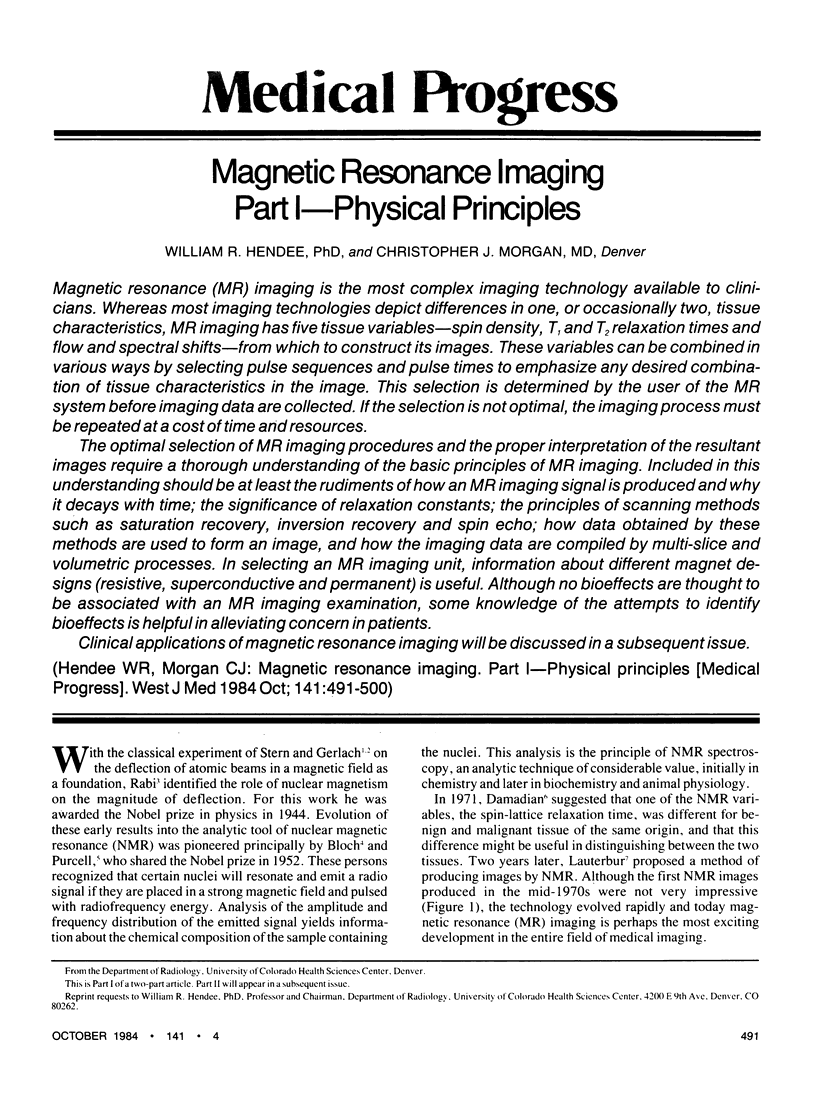
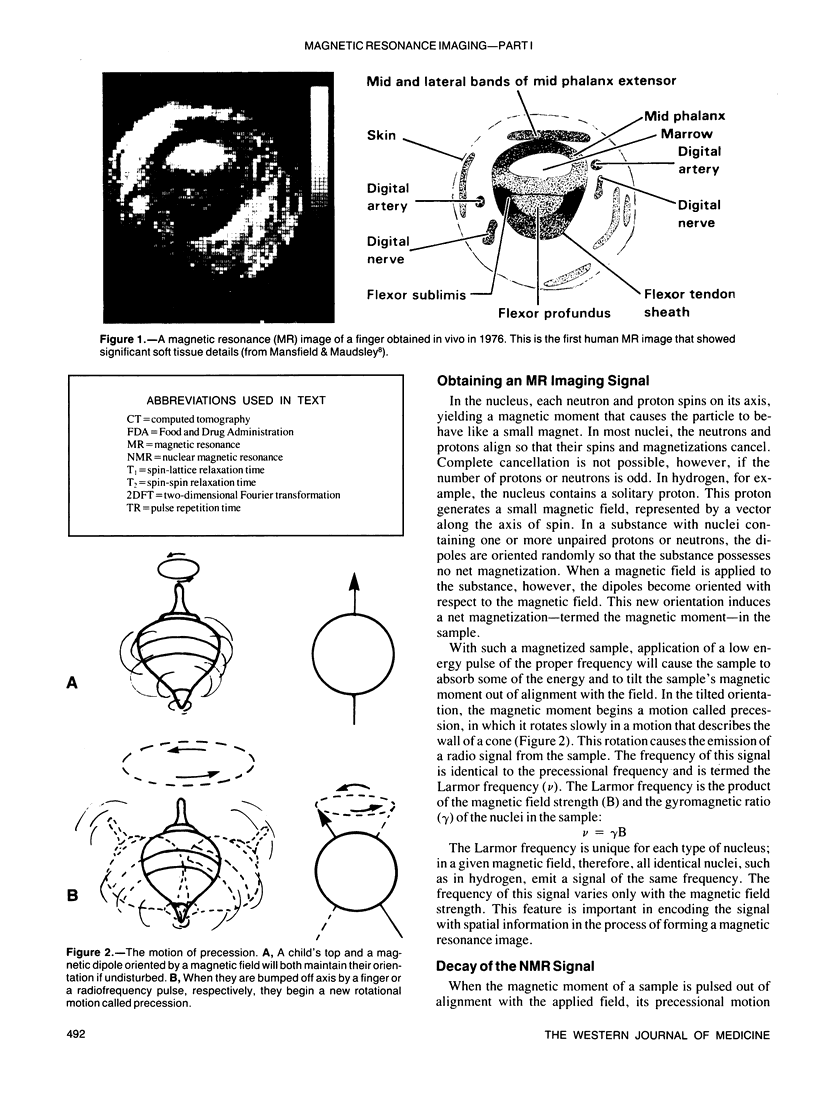
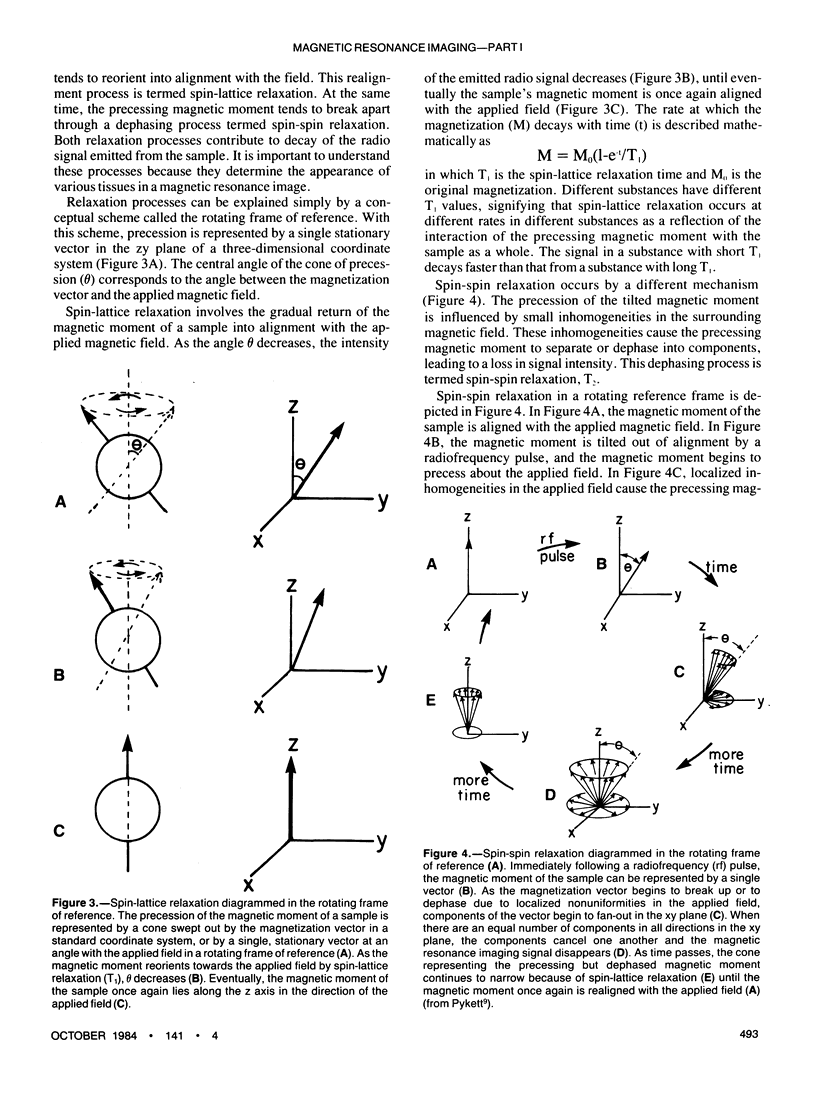
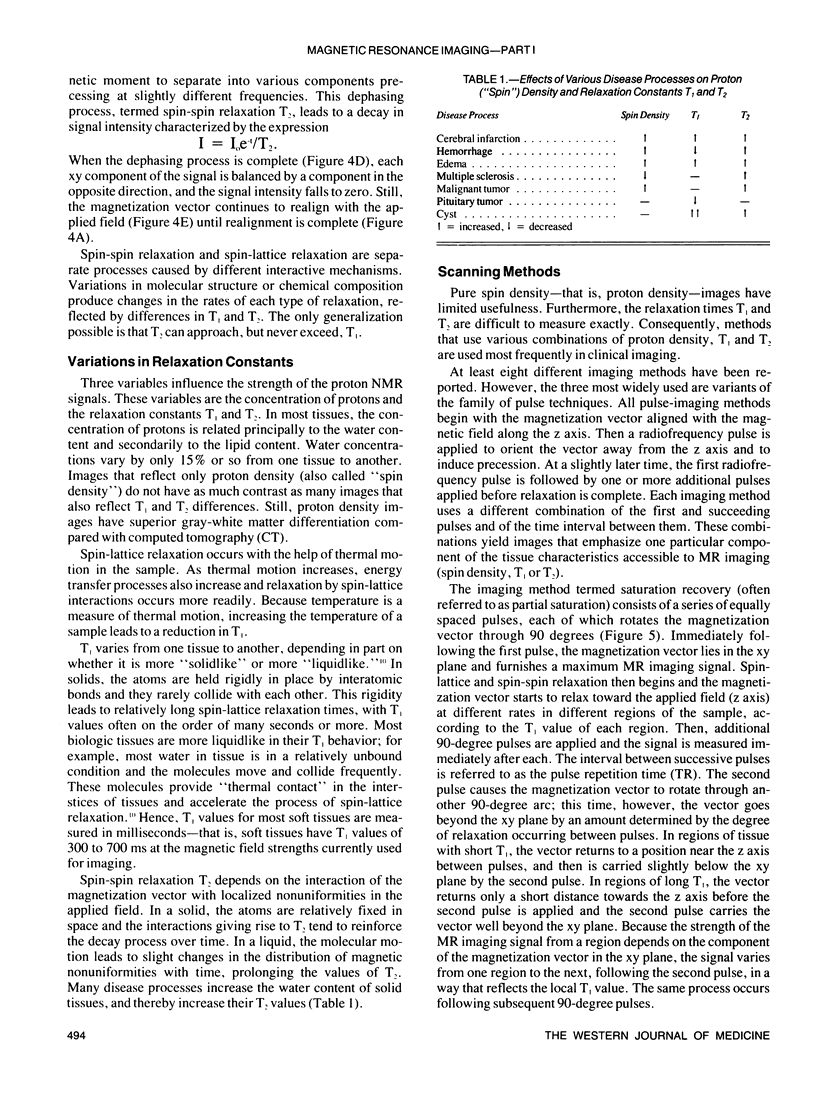
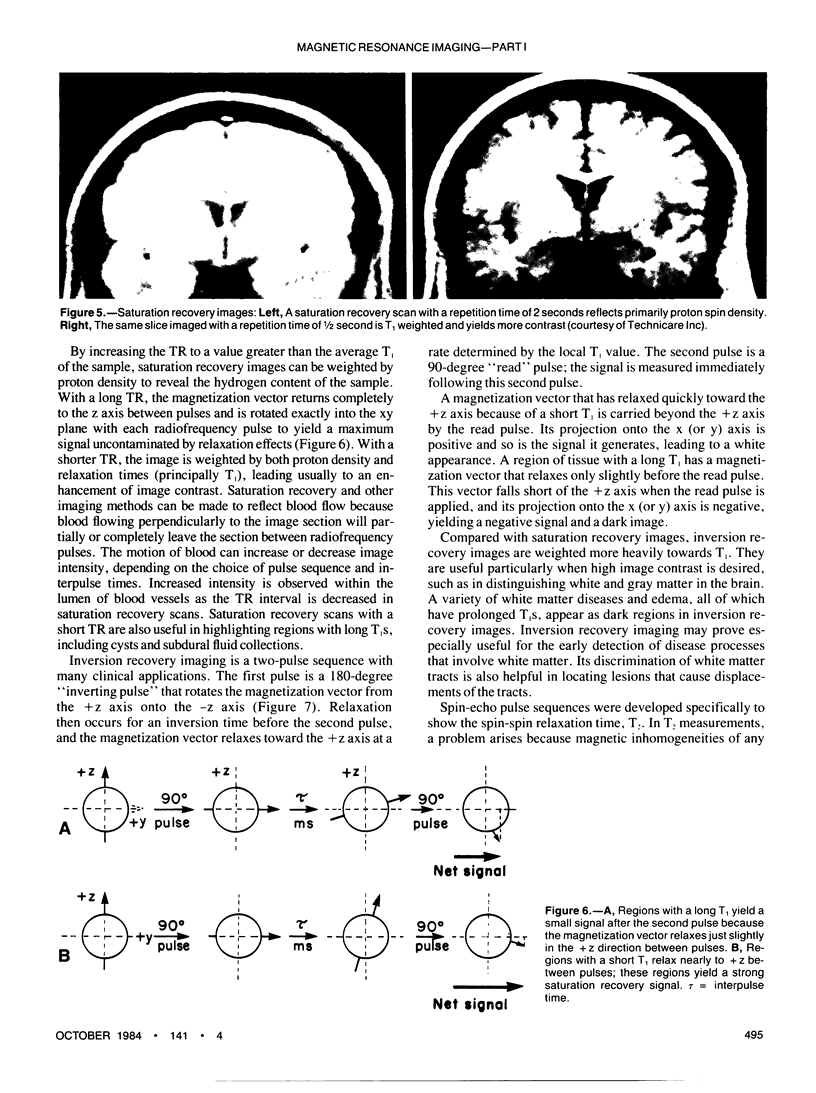
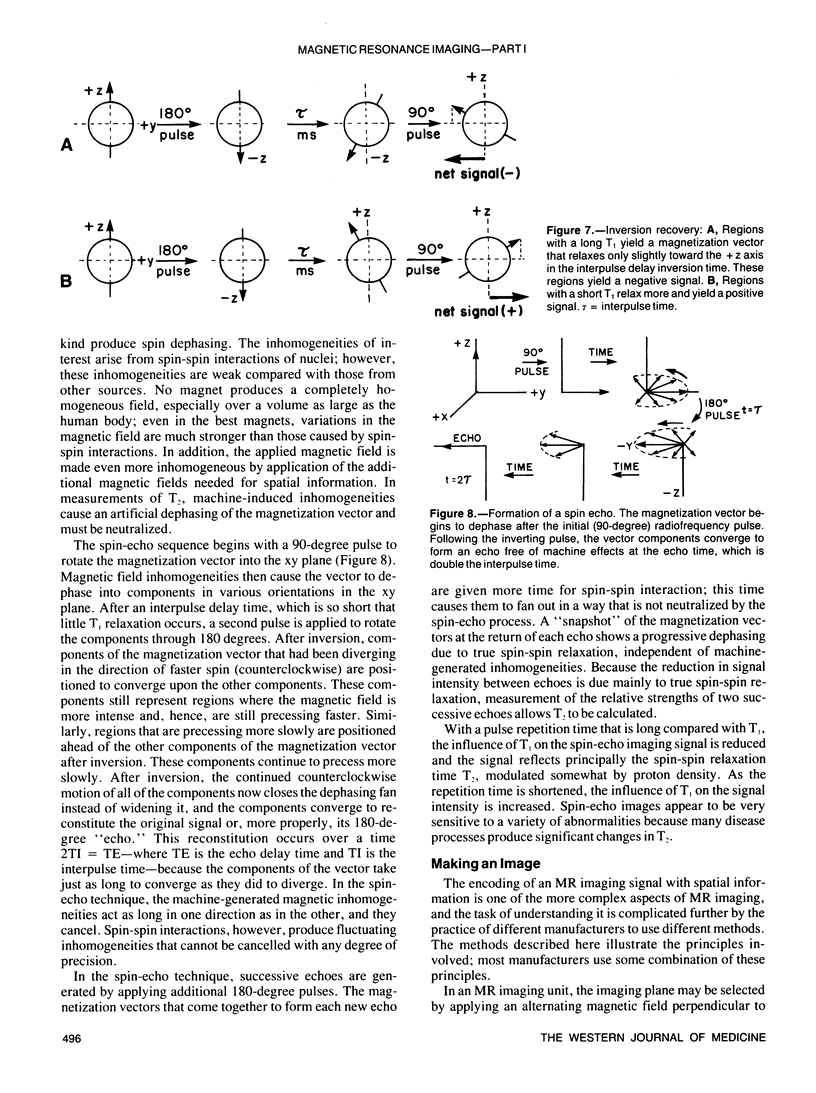
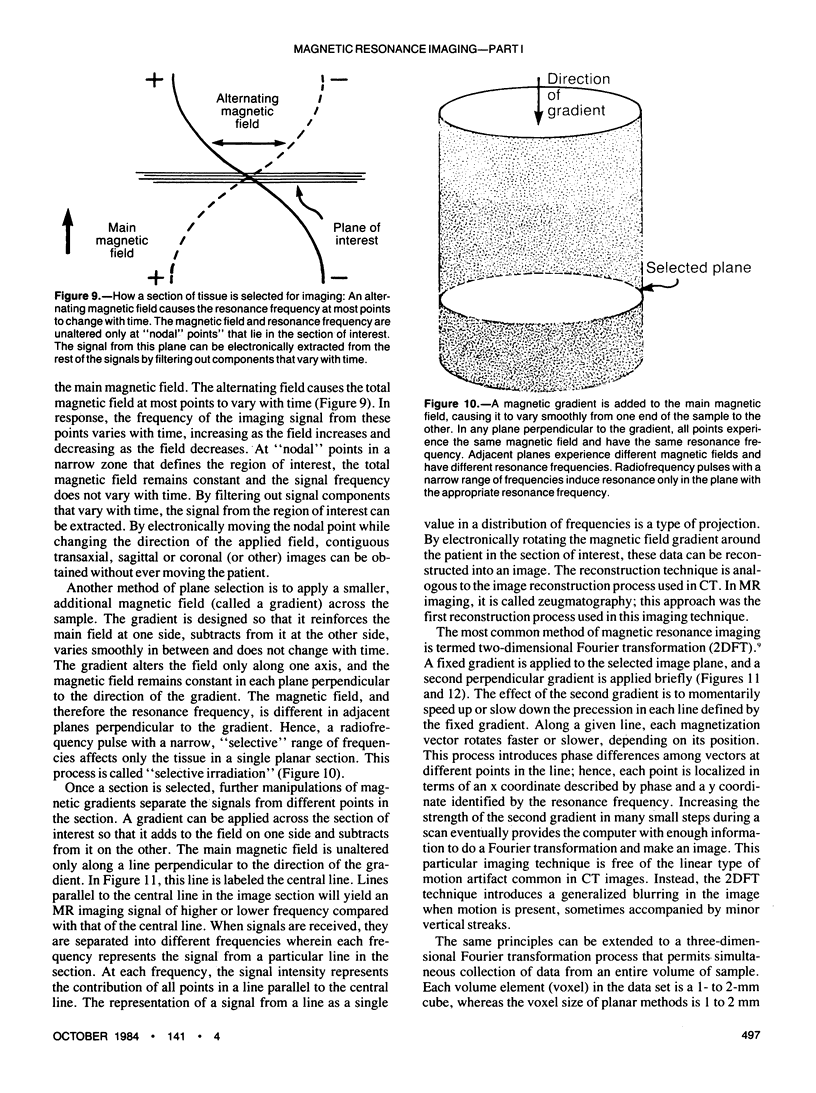
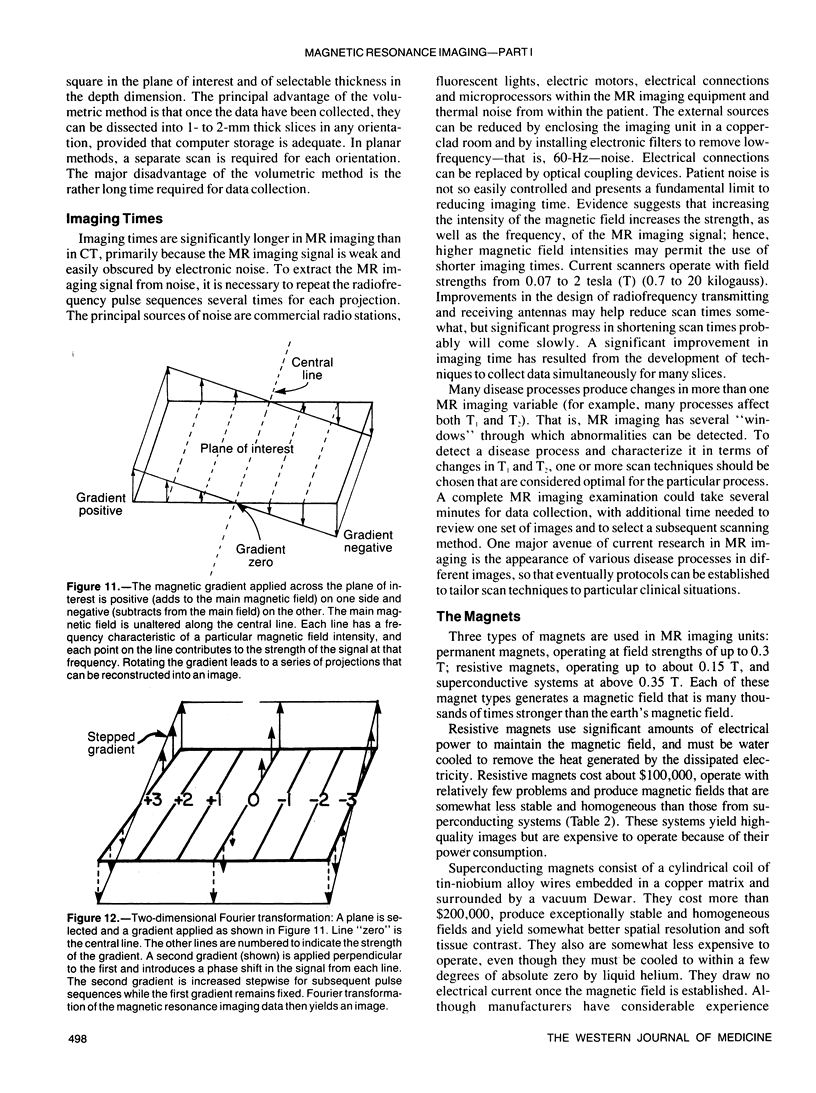
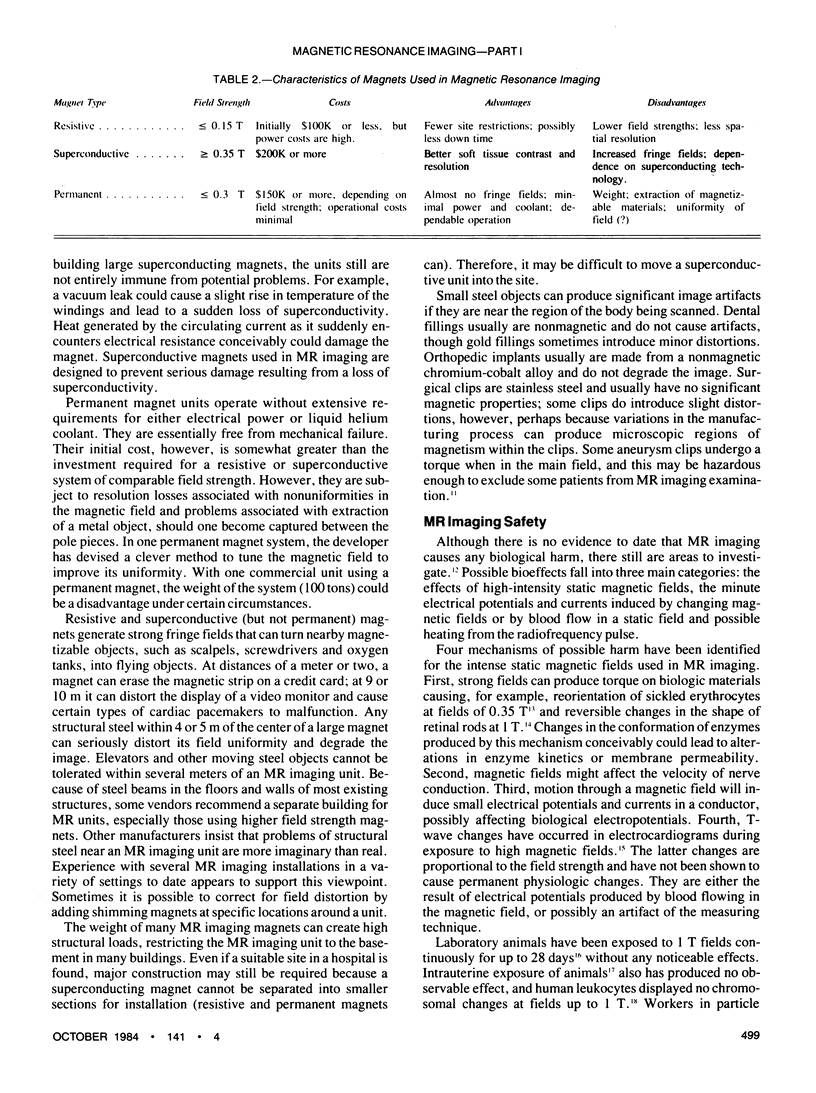
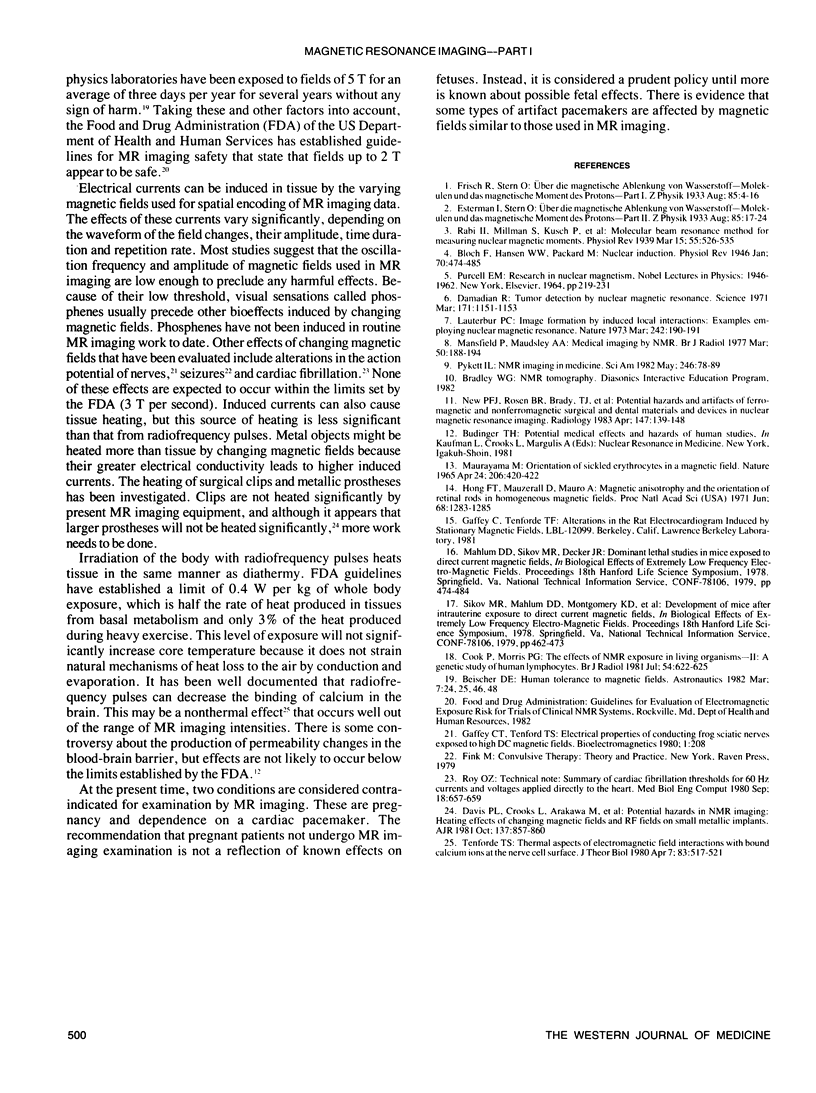
Images in this article
Selected References
These references are in PubMed. This may not be the complete list of references from this article.
- Cooke P., Morris P. G. The effects of NMR exposure on living organisms. II. A genetic study of human lymphocytes. Br J Radiol. 1981 Jul;54(643):622–625. doi: 10.1259/0007-1285-54-643-622. [DOI] [PubMed] [Google Scholar]
- Davis P. L., Crooks L., Arakawa M., McRee R., Kaufman L., Margulis A. R. Potential hazards in NMR imaging: heating effects of changing magnetic fields and RF fields on small metallic implants. AJR Am J Roentgenol. 1981 Oct;137(4):857–860. doi: 10.2214/ajr.137.4.857. [DOI] [PubMed] [Google Scholar]
- Hong F. T., Mauzerall D., Mauro A. Magnetic anisotropy and the orientation of retinal rods in a homogeneous magnetic field. Proc Natl Acad Sci U S A. 1971 Jun;68(6):1283–1285. doi: 10.1073/pnas.68.6.1283. [DOI] [PMC free article] [PubMed] [Google Scholar]
- Mansfield P., Maudsley A. A. Medical imaging by NMR. Br J Radiol. 1977 Mar;50(591):188–194. doi: 10.1259/0007-1285-50-591-188. [DOI] [PubMed] [Google Scholar]
- New P. F., Rosen B. R., Brady T. J., Buonanno F. S., Kistler J. P., Burt C. T., Hinshaw W. S., Newhouse J. H., Pohost G. M., Taveras J. M. Potential hazards and artifacts of ferromagnetic and nonferromagnetic surgical and dental materials and devices in nuclear magnetic resonance imaging. Radiology. 1983 Apr;147(1):139–148. doi: 10.1148/radiology.147.1.6828719. [DOI] [PubMed] [Google Scholar]
- Roy O. Z. Summary of cardiac fibrillation thresholds for 60 Hz currents and voltages applied directly to the heart. Med Biol Eng Comput. 1980 Sep;18(5):657–659. doi: 10.1007/BF02443140. [DOI] [PubMed] [Google Scholar]
- Tenforde T. S. Thermal aspects of electromagnetic field interactions with bound calcium ions at the nerve cell surface. J Theor Biol. 1980 Apr 7;83(3):517–521. doi: 10.1016/0022-5193(80)90057-0. [DOI] [PubMed] [Google Scholar]