Abstract
Time courses of cytoplasmic and vacuolar pH changes under salt stress were monitored by in vivo31P-nuclear magnetic resonance spectroscopy in intact cells of Nitellopsis obtusa. When cells were treated with 100 millimolar NaCl for 2 hours, the cytoplasmic pH deceased from 7.2 to 7.0, while the vacuolar pH increased from 4.9 to 5.2. This salt-induced breakdown of the pH gradient between the cytoplasm and the vacuole was also confirmed through direct measurements of change in vacuolar pH with a micro-pH electrode. We speculate that the intracellular pH changes induced by the salt stress mainly results from the inhibition of the H+-translocating pyrophosphatase in the vacuolar membrane, since this H+-translocating system is sensitive to salt-induced increase in the cytoplasmic [Na+] and a simultaneous decrease in the cytoplasmic [K+]. Since disturbance of the cytoplasmic pH value should have serious consequences on the homeostasis of living cells, we propose that the salt-induced intracellular pH changes are one of initial and important steps that lead to cell death.
Full text
PDF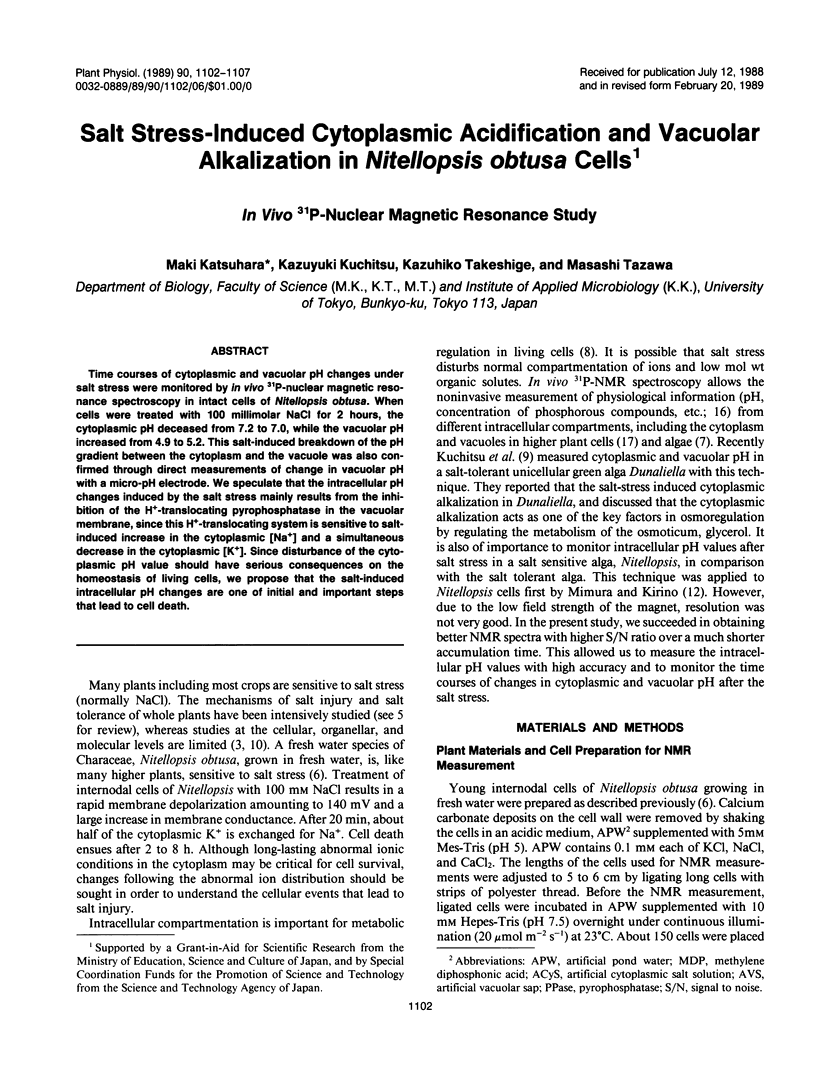
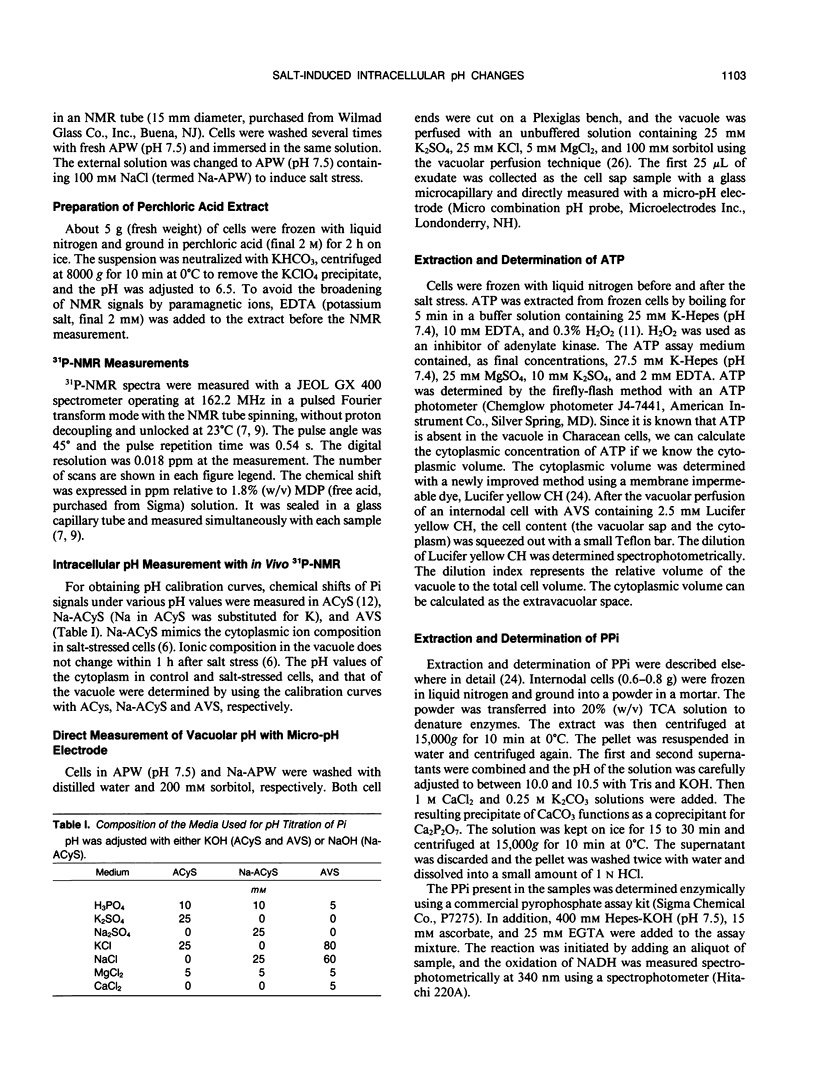
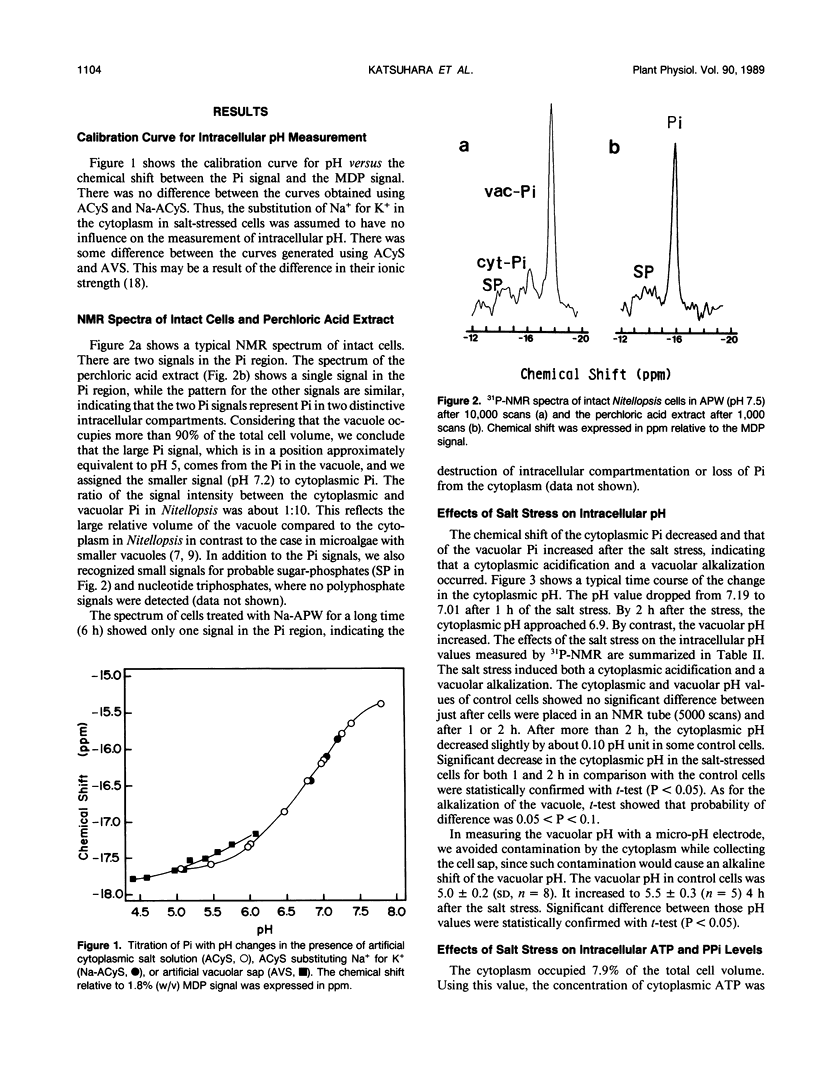
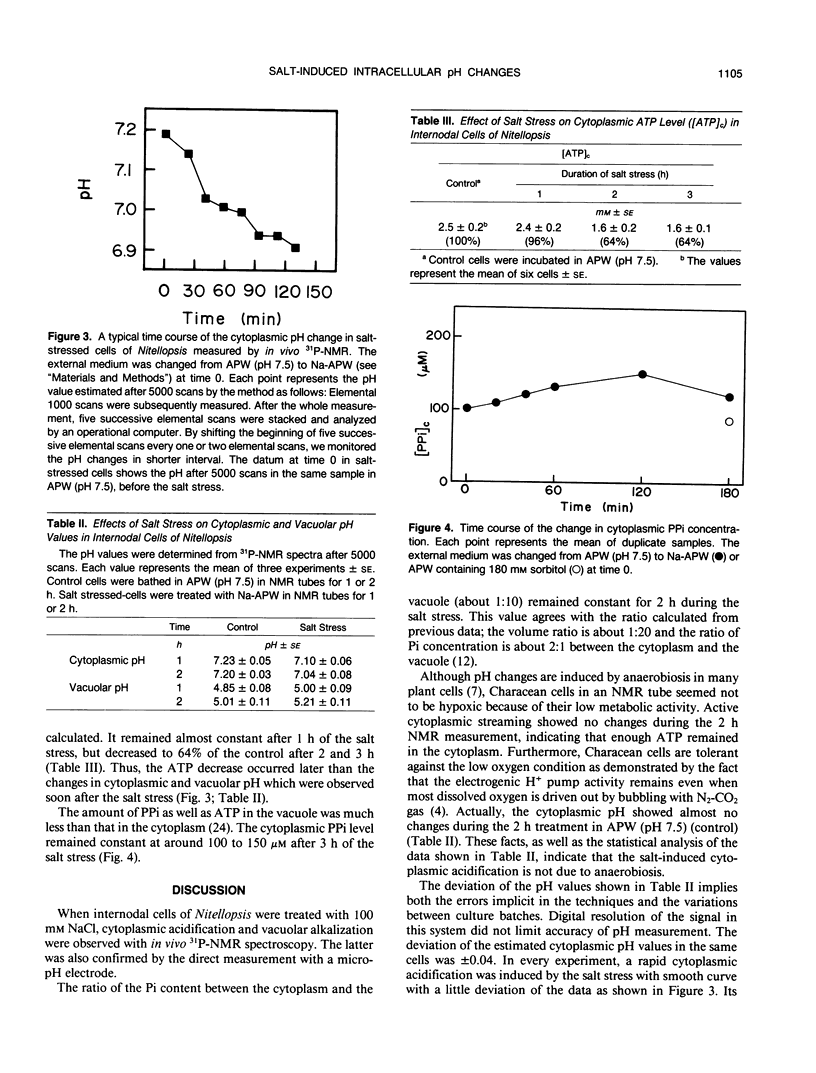
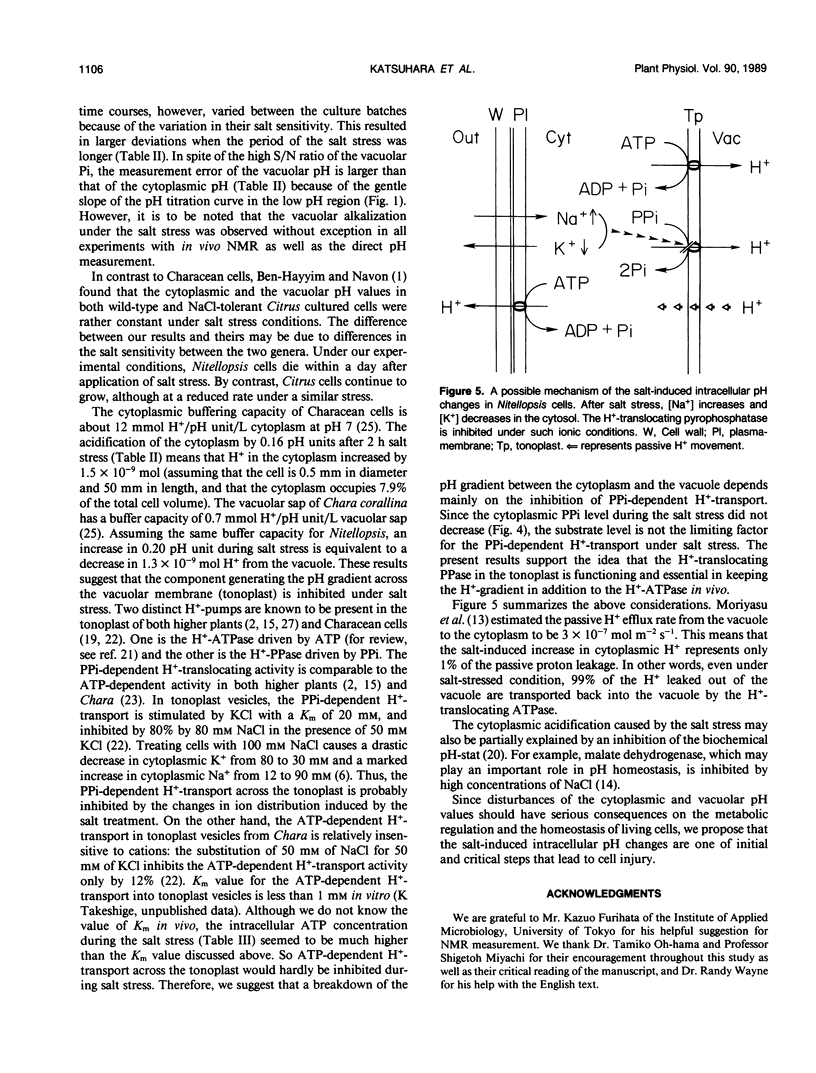
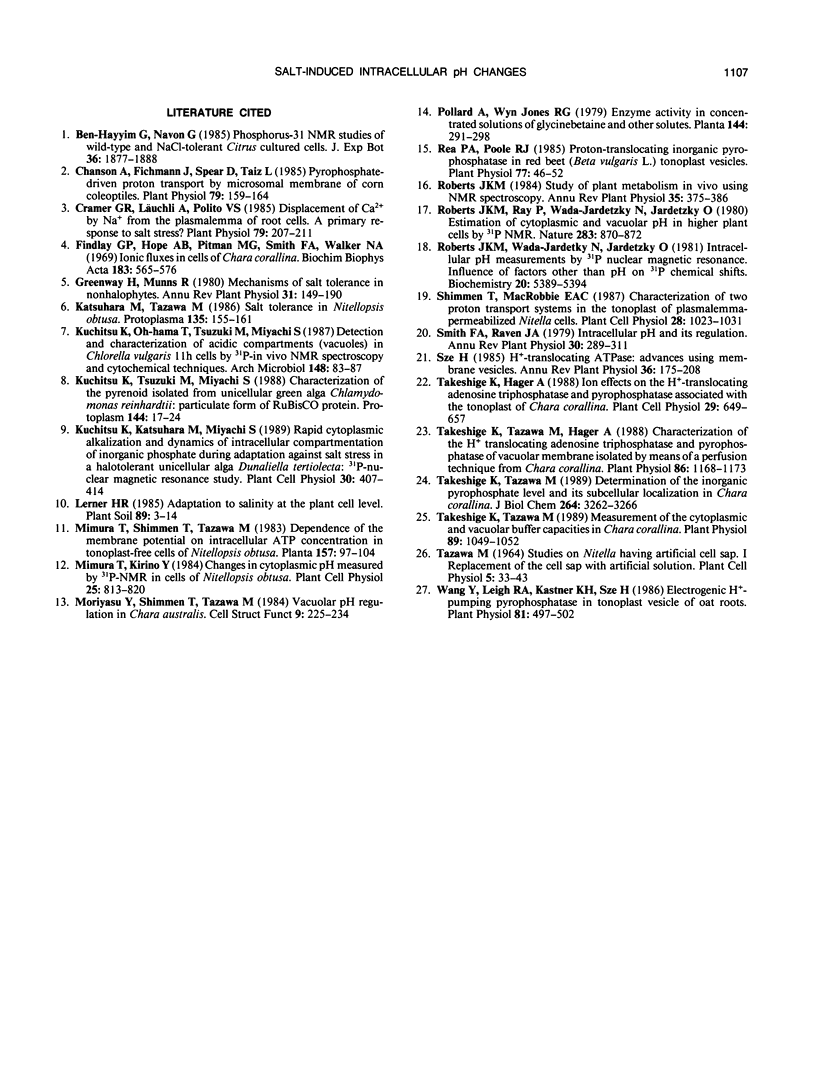
Selected References
These references are in PubMed. This may not be the complete list of references from this article.
- Chanson A., Fichmann J., Spear D., Taiz L. Pyrophosphate-driven proton transport by microsomal membranes of corn coleoptiles. Plant Physiol. 1985 Sep;79(1):159–164. doi: 10.1104/pp.79.1.159. [DOI] [PMC free article] [PubMed] [Google Scholar]
- Cramer G. R., Läuchli A., Polito V. S. Displacement of ca by na from the plasmalemma of root cells : a primary response to salt stress? Plant Physiol. 1985 Sep;79(1):207–211. doi: 10.1104/pp.79.1.207. [DOI] [PMC free article] [PubMed] [Google Scholar]
- Findlay G. P., Hope A. B., Pitman M. G., Smith F. A., Walker N. A. Ionic fluxes in cells of Chara corallina. Biochim Biophys Acta. 1969;183(3):565–576. doi: 10.1016/0005-2736(69)90170-9. [DOI] [PubMed] [Google Scholar]
- Rea P. A., Poole R. J. Proton-Translocating Inorganic Pyrophosphatase in Red Beet (Beta vulgaris L.) Tonoplast Vesicles. Plant Physiol. 1985 Jan;77(1):46–52. doi: 10.1104/pp.77.1.46. [DOI] [PMC free article] [PubMed] [Google Scholar]
- Roberts J. K., Wade-Jardetzky N., Jardetzky O. Intracellular pH measurements by 31P nuclear magnetic resonance. Influence of factors other than pH on 31P chemical shifts. Biochemistry. 1981 Sep 15;20(19):5389–5394. doi: 10.1021/bi00522a006. [DOI] [PubMed] [Google Scholar]
- Takeshige K., Tazawa M. Determination of the inorganic pyrophosphate level and its subcellular localization in Chara corallina. J Biol Chem. 1989 Feb 25;264(6):3262–3266. [PubMed] [Google Scholar]
- Takeshige K., Tazawa M., Hager A. Characterization of the H Translocating Adenosine Triphosphatase and Pyrophosphatase of Vacuolar Membranes Isolated by Means of a Perfusion Technique from Chara corallina. Plant Physiol. 1988 Apr;86(4):1168–1173. doi: 10.1104/pp.86.4.1168. [DOI] [PMC free article] [PubMed] [Google Scholar]
- Takeshige K., Tazawa M. Measurement of the Cytoplasmic and Vacuolar Buffer Capacities in Chara corallina. Plant Physiol. 1989 Apr;89(4):1049–1052. doi: 10.1104/pp.89.4.1049. [DOI] [PMC free article] [PubMed] [Google Scholar]
- Wang Y., Leigh R. A., Kaestner K. H., Sze H. Electrogenic h-pumping pyrophosphatase in tonoplast vesicles of oat roots. Plant Physiol. 1986 Jun;81(2):497–502. doi: 10.1104/pp.81.2.497. [DOI] [PMC free article] [PubMed] [Google Scholar]