Abstract
Development of a legume root nodule is a complex process culminating in a plant/bacterial symbiosis possessing the capacity for biological dinitrogen fixation. Formation of root nodules is initiated by the binding and stabilization of rhizobia to plant root hairs, mediated in part by a receptor/ligand recognition system composed of lectins on the plant root surface and lectin-binding sites on the rhizobial cell surface. The dinitrogen fixation activity of these root nodules may be an important feature of enclosed, space-based life support systems, and may provide an ecological method to recycle nitrogen for amino acid production. However, the effects on nodule development of varied gravitational fields, or of root nutrient delivery hardware, remain unknown. We have investigated the effects of microgravity on root nodule formation, with preliminary experiments focused upon the receptor/ligand component. Microgravity, obtained during parabolic flight aboard NASA 930, has no apparent effect on the binding of purified lectin to rhizobia, a result that will facilitate forthcoming experiments using intact root tissues.
Full text
PDF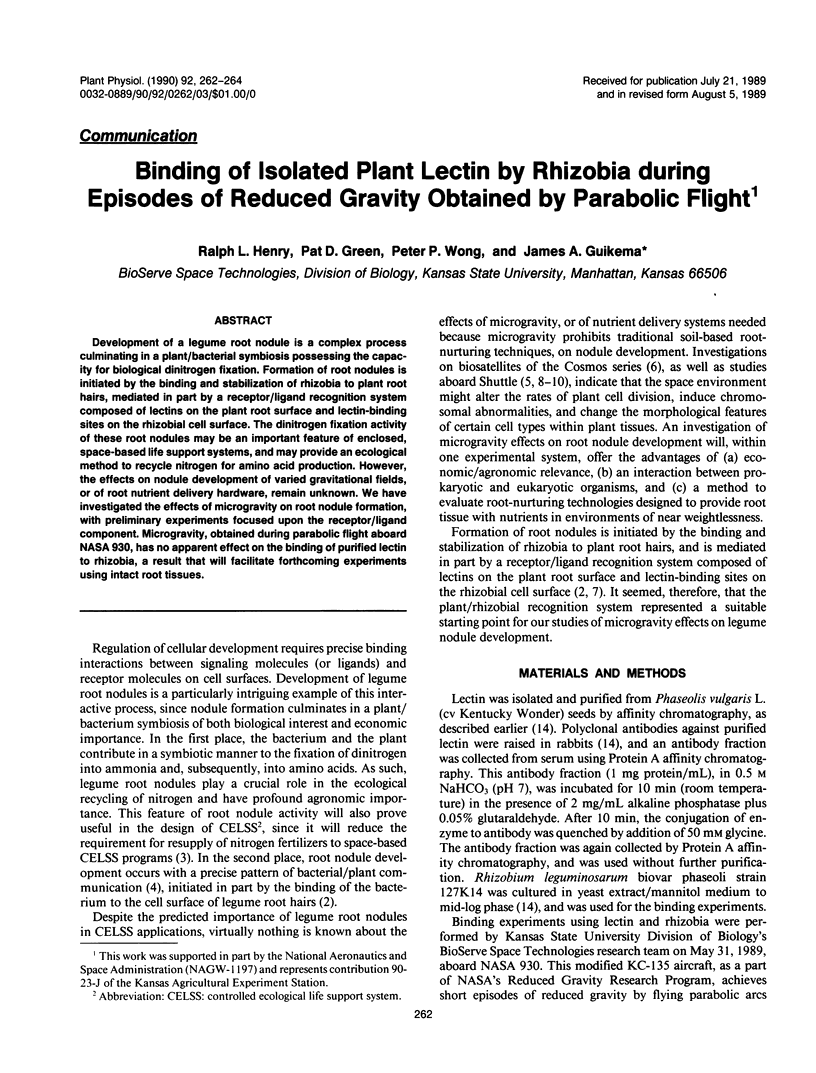
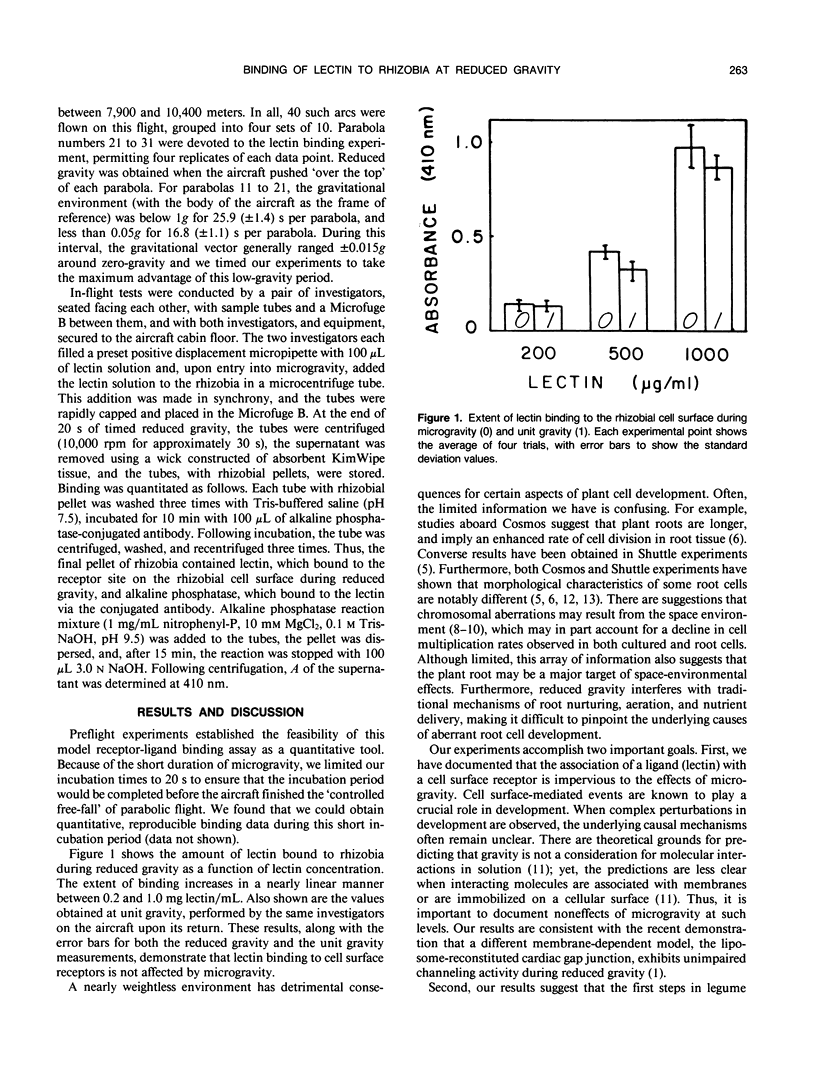
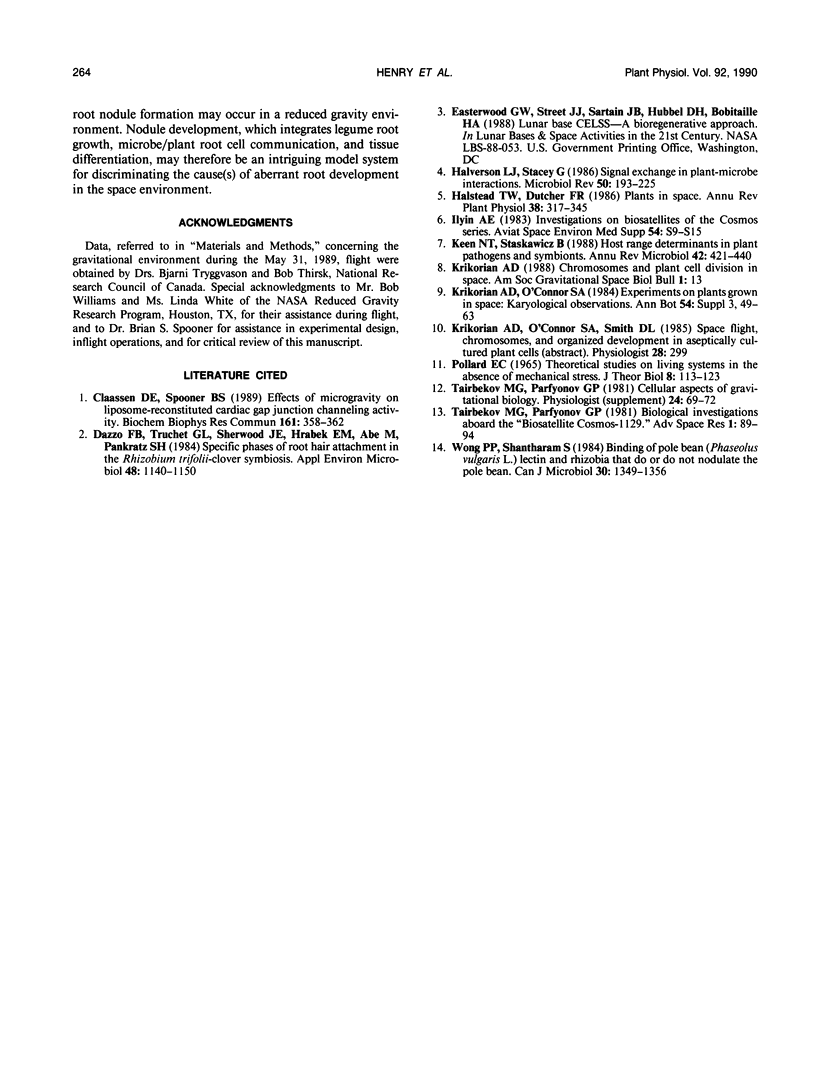
Selected References
These references are in PubMed. This may not be the complete list of references from this article.
- Claassen D. E., Spooner B. S. Effects of microgravity on liposome-reconstituted cardiac gap junction channeling activity. Biochem Biophys Res Commun. 1989 May 30;161(1):358–362. doi: 10.1016/0006-291x(89)91605-7. [DOI] [PubMed] [Google Scholar]
- Dazzo F. B., Truchet G. L., Sherwood J. E., Hrabak E. M., Abe M., Pankratz S. H. Specific phases of root hair attachment in the Rhizobium trifolii-clover symbiosis. Appl Environ Microbiol. 1984 Dec;48(6):1140–1150. doi: 10.1128/aem.48.6.1140-1150.1984. [DOI] [PMC free article] [PubMed] [Google Scholar]
- Halstead T. W., Dutcher F. R. Plants in space. Annu Rev Plant Physiol. 1987;38:317–345. doi: 10.1146/annurev.pp.38.060187.001533. [DOI] [PubMed] [Google Scholar]
- Halverson L. J., Stacey G. Signal exchange in plant-microbe interactions. Microbiol Rev. 1986 Jun;50(2):193–225. doi: 10.1128/mr.50.2.193-225.1986. [DOI] [PMC free article] [PubMed] [Google Scholar]
- Ilyin E. A. Investigations on biosatellites of the Cosmos series. Aviat Space Environ Med. 1983 Dec;54(12 Pt 2):S9–15. [PubMed] [Google Scholar]
- Krikorian A. D., O'Connor S. A. Karyological observations. Ann Bot. 1984 Nov;54(Suppl 3):49–63. doi: 10.1093/oxfordjournals.aob.a086866. [DOI] [PubMed] [Google Scholar]
- Pollard E. C. Theoretical studies on living systems in the absence of mechanical stress. J Theor Biol. 1965 Jan;8(1):113–123. doi: 10.1016/0022-5193(65)90097-4. [DOI] [PubMed] [Google Scholar]
- Tairbekov M. G., Parfyonov G. P., Platonova R. W., Abramova V. M., Golov V. K., Rostopshina A. V., Lyubchenko VYu, Chuchkin V. G. Biological investigations aboard the biosatellite Cosmos-1129. Adv Space Res. 1981;1(14):89–94. doi: 10.1016/0273-1177(81)90248-9. [DOI] [PubMed] [Google Scholar]