Abstract
The relationship between photochemical quantum yield (φs) and fluorescence yield have been investigated in leaf tissue from Nicotiana tabacum using CO2 exchange and a modulated fluorescence measuring system. The quantum yield of CO2 fixation at 1.6% (v/v) O2 and limiting irradiance was reduced 20% by increasing the mean H2O vapor pressure deficit (VPD) from 9.2 to 18.6 mbars. As [CO2] and irradiance were varied, the intrinsic quantum yield of open photosystem II units (φs/qQ where qQ is the photochemical fluorescence quenching coefficient) declined linearly with the degree of nonphotochemical fluorescence quenching. The slope and y-intercept values for this function were significantly reduced when the mean VPD was 18.4 millibars relative to 8.9 millibars. Susceptibility of the leaf tissue to photoinhibition was unaffected by VPD. Elevated O2 concentrations (20.5% v/v) reduced the intrinsic quantum yield of net CO2 uptake due to the occurrence of O2-reducing processes. However, the relative effect of high VPD compared to low VPD on intrinsic quantum yield was not dependent on the O2 level. This suggests that the Mehler reaction does not mediate the response of quantum yield to elevated VPD. The results are discussed with regard to the possible role of transpiration stress in regulating dissipation of excitation by electron transport pathways other than noncyclic electron flow supporting reduction of CO2 and/or O2.
Full text
PDF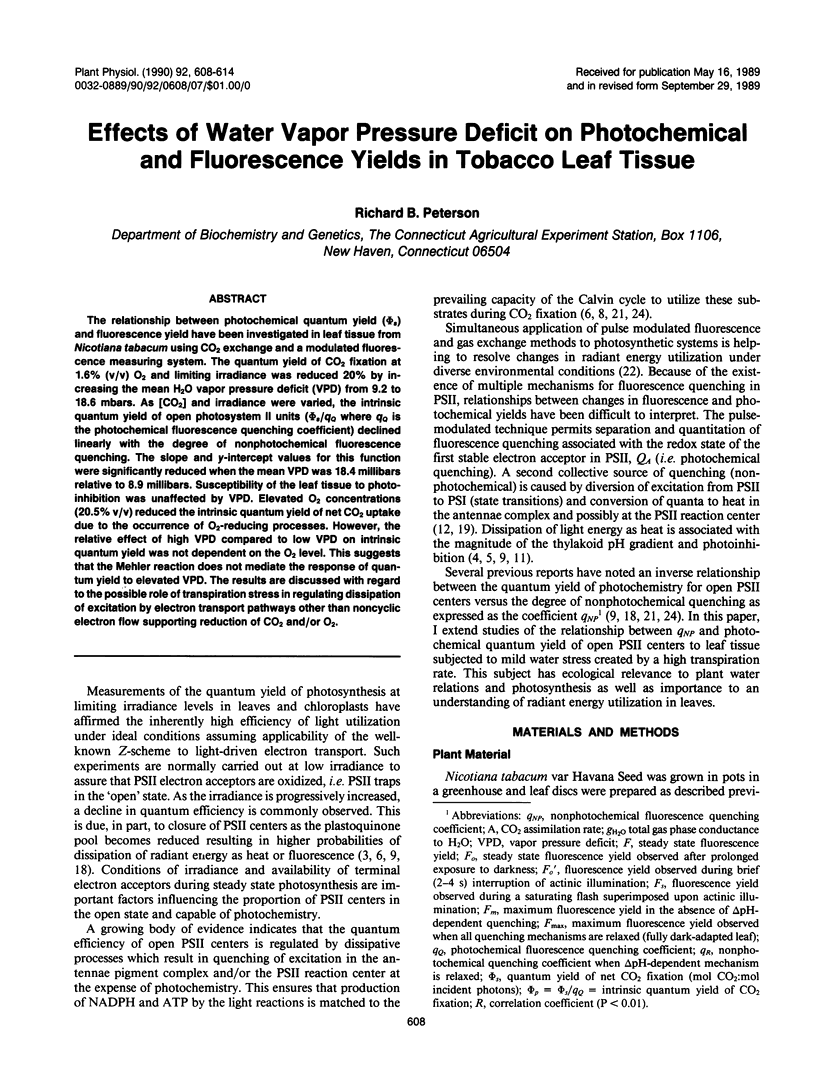
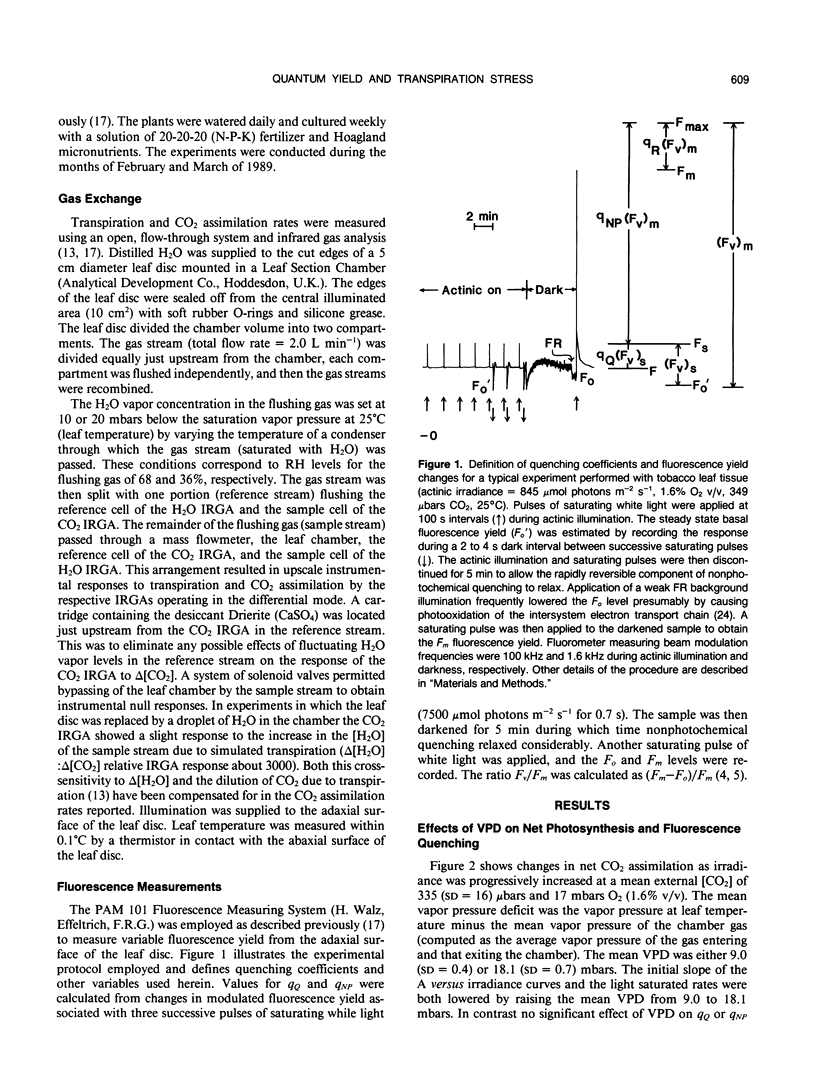
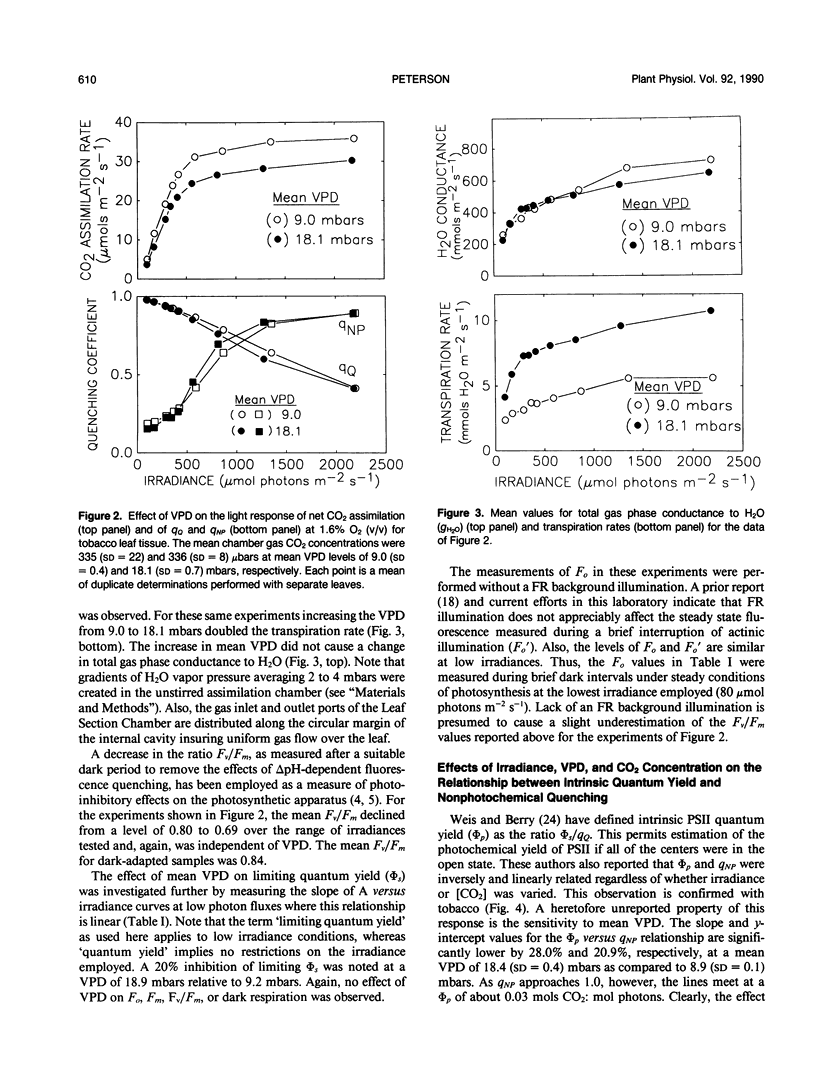
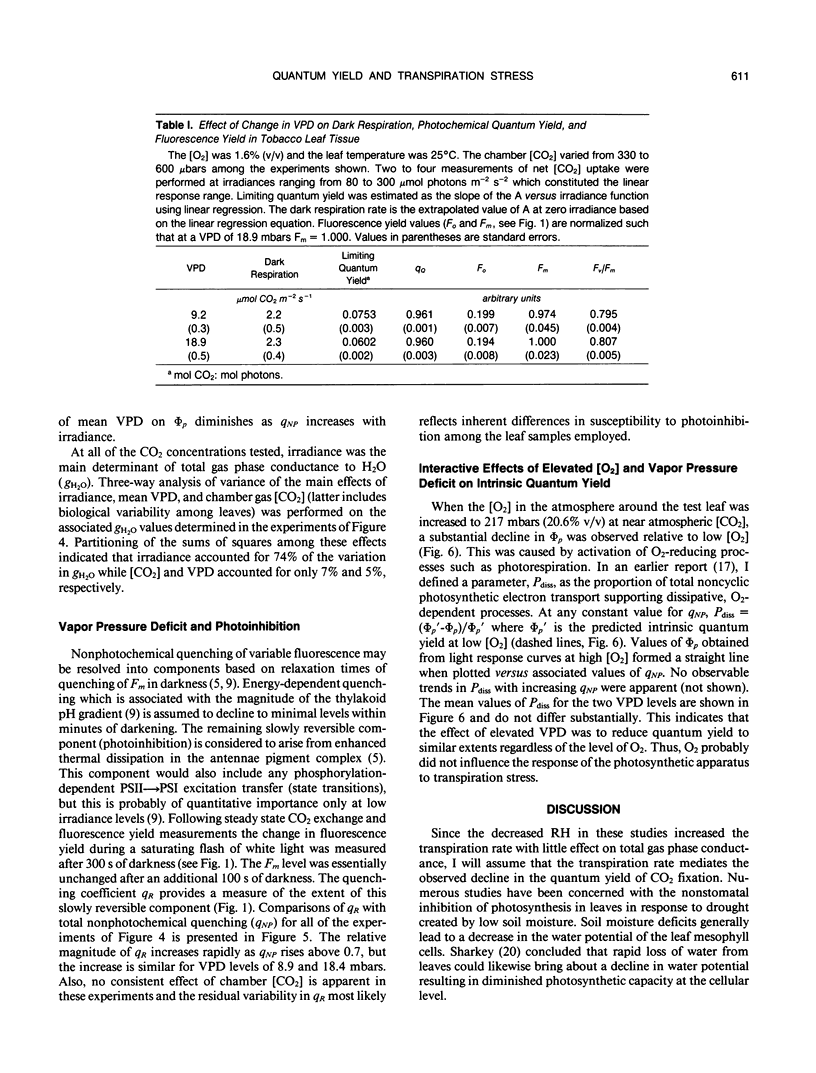
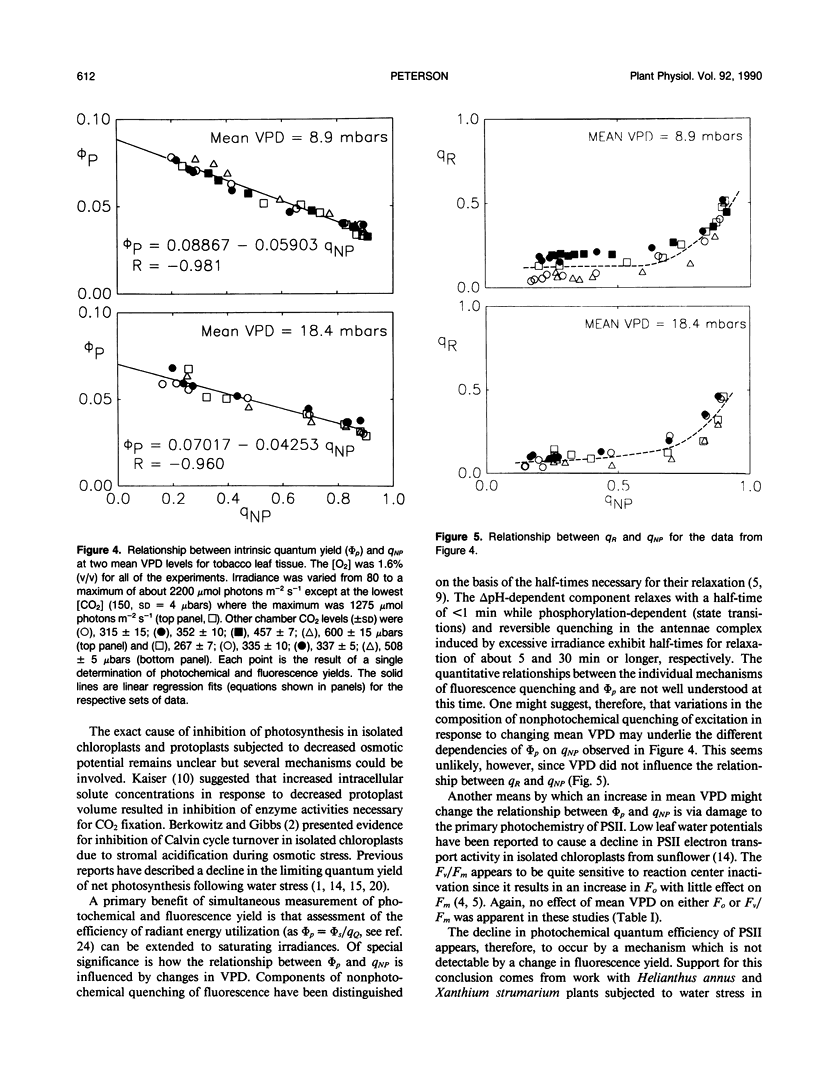
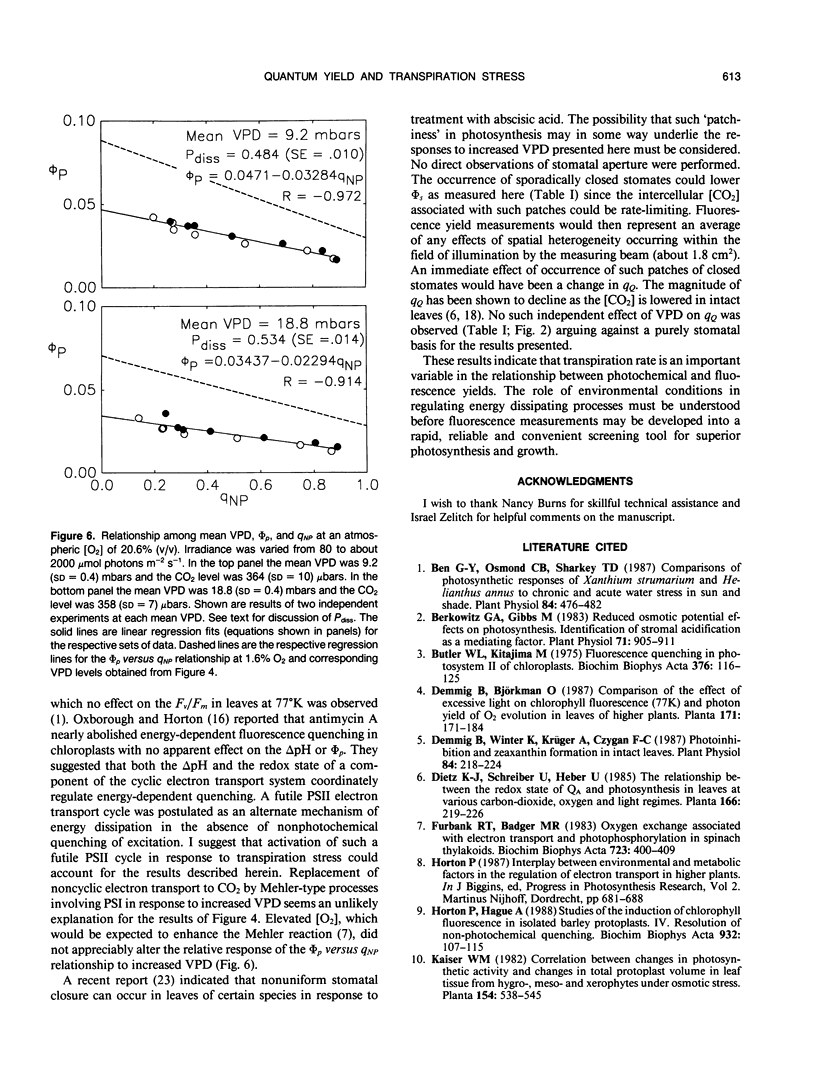
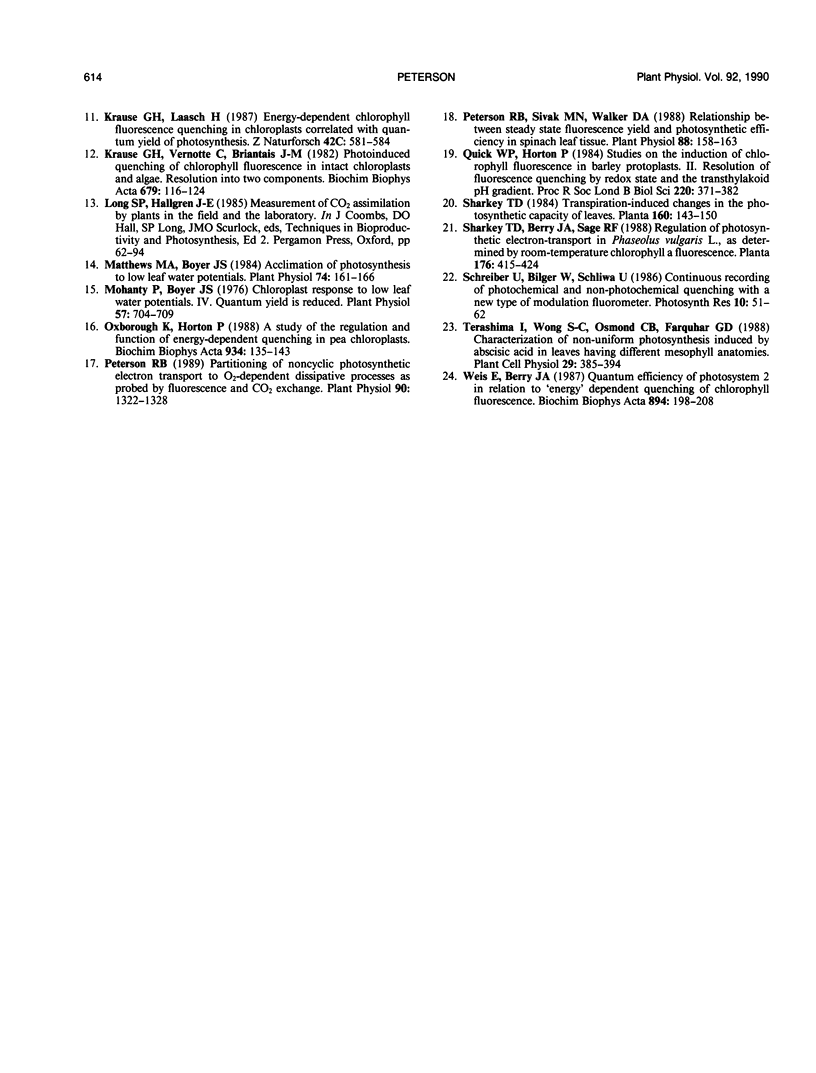
Selected References
These references are in PubMed. This may not be the complete list of references from this article.
- Ben G. Y., Osmond C. B., Sharkey T. D. Comparisons of Photosynthetic Responses of Xanthium strumarium and Helianthus annuus to Chronic and Acute Water Stress in Sun and Shade. Plant Physiol. 1987 Jun;84(2):476–482. doi: 10.1104/pp.84.2.476. [DOI] [PMC free article] [PubMed] [Google Scholar]
- Berkowitz G. A., Gibbs M. Reduced osmotic potential effects on photosynthesis : identification of stromal acidification as a mediating factor. Plant Physiol. 1983 Apr;71(4):905–911. doi: 10.1104/pp.71.4.905. [DOI] [PMC free article] [PubMed] [Google Scholar]
- Butler W. L., Kitajima M. Fluorescence quenching in photosystem II of chloroplasts. Biochim Biophys Acta. 1975 Jan 31;376(1):116–125. doi: 10.1016/0005-2728(75)90210-8. [DOI] [PubMed] [Google Scholar]
- Demmig B., Winter K., Krüger A., Czygan F. C. Photoinhibition and zeaxanthin formation in intact leaves : a possible role of the xanthophyll cycle in the dissipation of excess light energy. Plant Physiol. 1987 Jun;84(2):218–224. doi: 10.1104/pp.84.2.218. [DOI] [PMC free article] [PubMed] [Google Scholar]
- Matthews M. A., Boyer J. S. Acclimation of photosynthesis to low leaf water potentials. Plant Physiol. 1984 Jan;74(1):161–166. doi: 10.1104/pp.74.1.161. [DOI] [PMC free article] [PubMed] [Google Scholar]
- Mohanty P., Boyer J. S. Chloroplast Response to Low Leaf Water Potentials: IV. Quantum Yield Is Reduced. Plant Physiol. 1976 May;57(5):704–709. doi: 10.1104/pp.57.5.704. [DOI] [PMC free article] [PubMed] [Google Scholar]
- Peterson R. B. Partitioning of Noncyclic Photosynthetic Electron Transport to O(2)-Dependent Dissipative Processes as Probed by Fluorescence and CO(2) Exchange. Plant Physiol. 1989 Aug;90(4):1322–1328. doi: 10.1104/pp.90.4.1322. [DOI] [PMC free article] [PubMed] [Google Scholar]
- Peterson R. B., Sivak M. N., Walker D. A. Relationship between Steady-State Fluorescence Yield and Photosynthetic Efficiency in Spinach Leaf Tissue. Plant Physiol. 1988 Sep;88(1):158–163. doi: 10.1104/pp.88.1.158. [DOI] [PMC free article] [PubMed] [Google Scholar]