Abstract
A simple model based on HCO3− transport has been developed to relate photosynthesis and inorganic carbon fluxes for the marine cyanobacterium, Synechococcus sp. Nägeli (strain RRIMP N1). Predicted relationships between inorganic carbon transport, CO2 fixation, internal carbonic anhydrase activity, and leakage of CO2 out of the cell, allow comparisons to be made with experimentally obtained data. Measurements of inorganic carbon fluxes and internal inorganic carbon pool sizes in these cells were made by monitoring time-courses of CO2 changes (using a mass spectrometer) during light/dark transients. At just saturating CO2 conditions, total inorganic carbon transport did not exceed net CO2 fixation by more than 30%. This indicates CO2 leakage similar to that estimated for C4 plants.
For this leakage rate, the model predicts the cell would need a conductance to CO2 of around 10−5 centimeters per second. This is similar to estimates made for the same cells using inorganic carbon pool sizes and CO2 efflux measurements. The model predicts that carbonic anhydrase is necessary internally to allow a sufficiently fast rate of CO2 production to prevent a large accumulation of HCO3−. Intact cells show light stimulated carbonic anhydrase activity when assayed using 18O-labeled CO2 techniques. This is also supported by low but detectable levels of carbonic anhydrase activity in cell extracts, sufficient to meet the requirements of the model.
Full text
PDF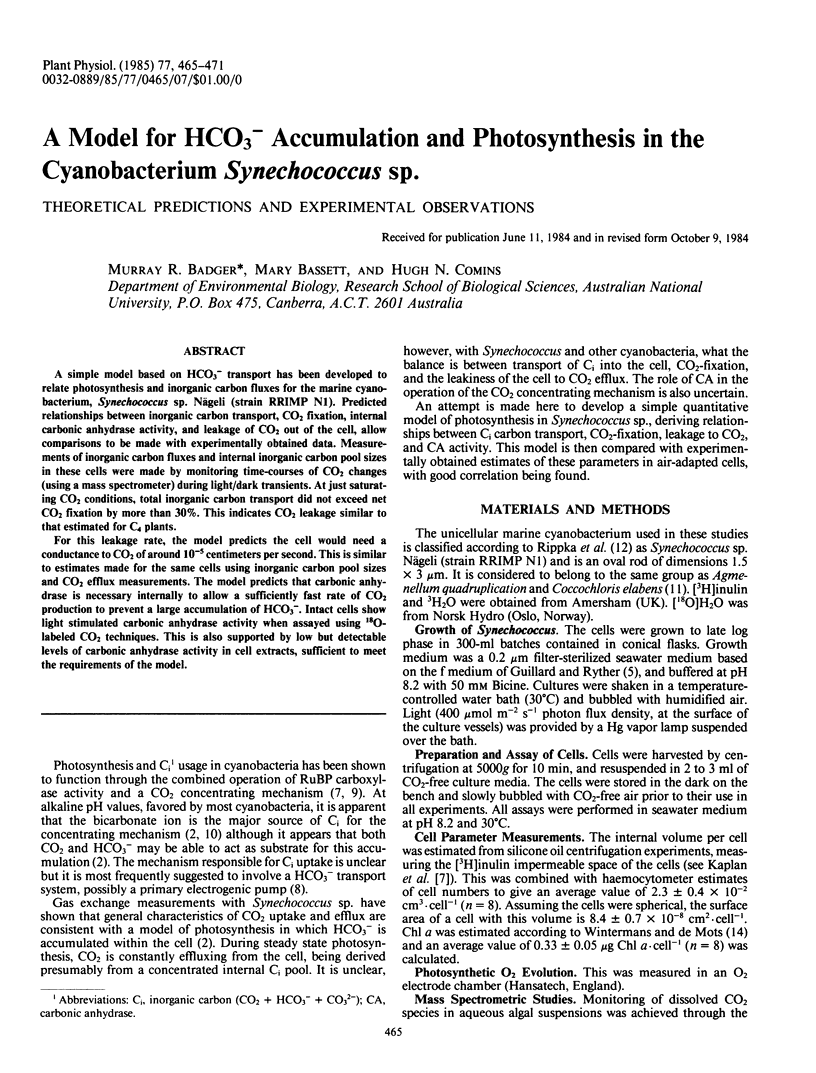
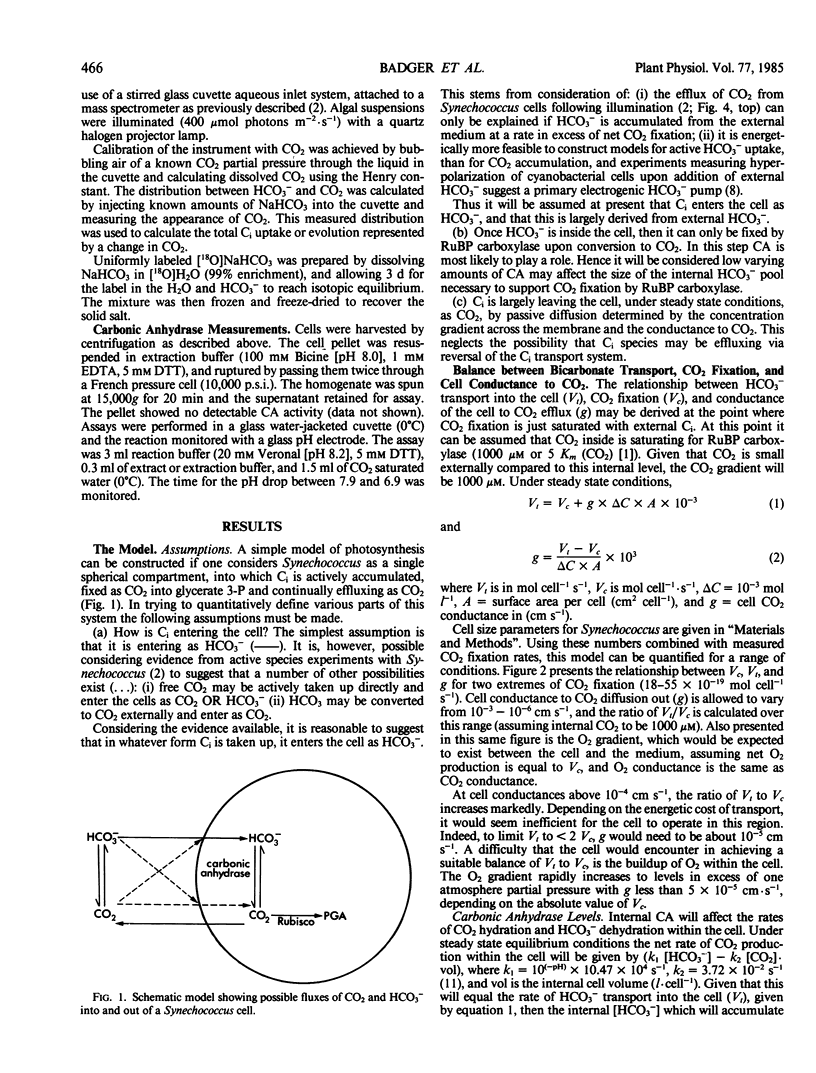
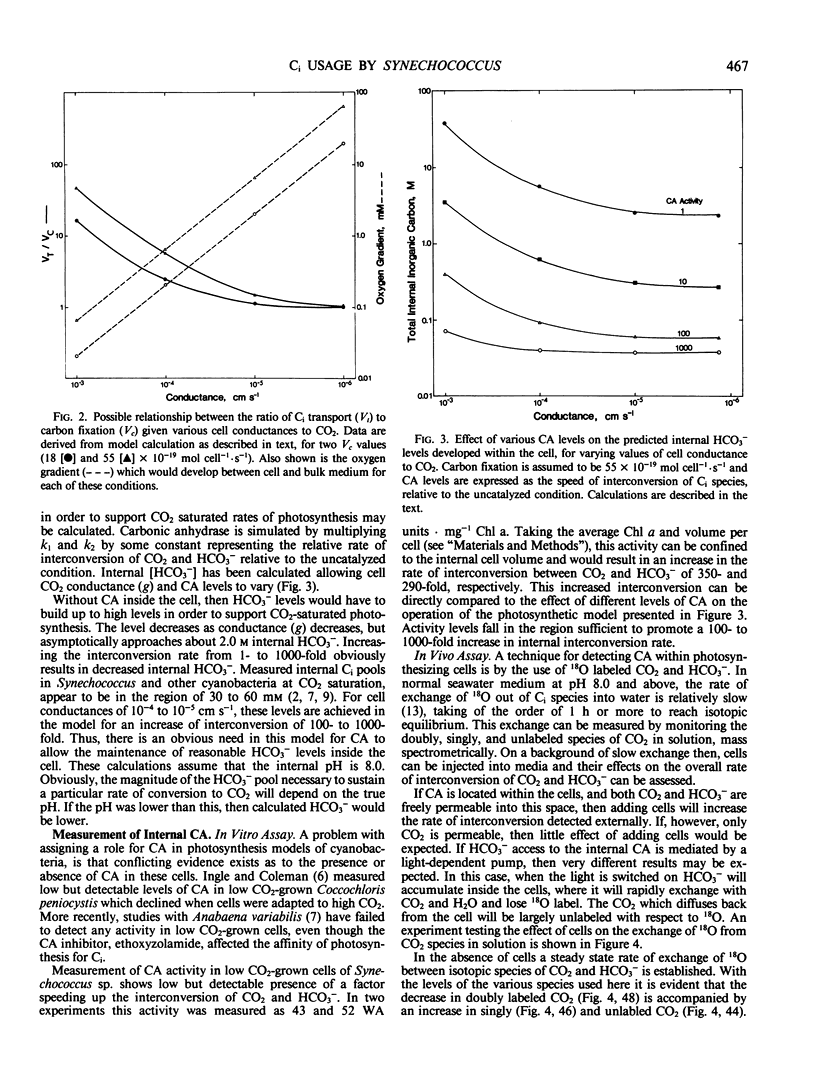
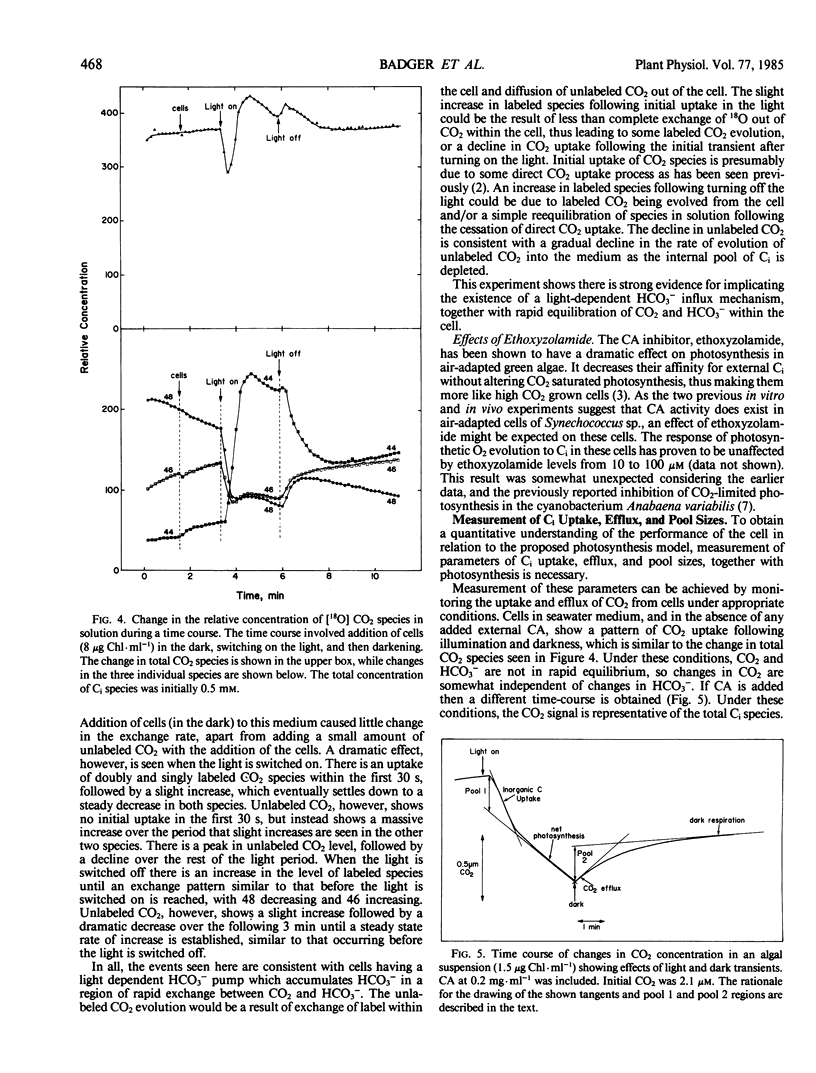
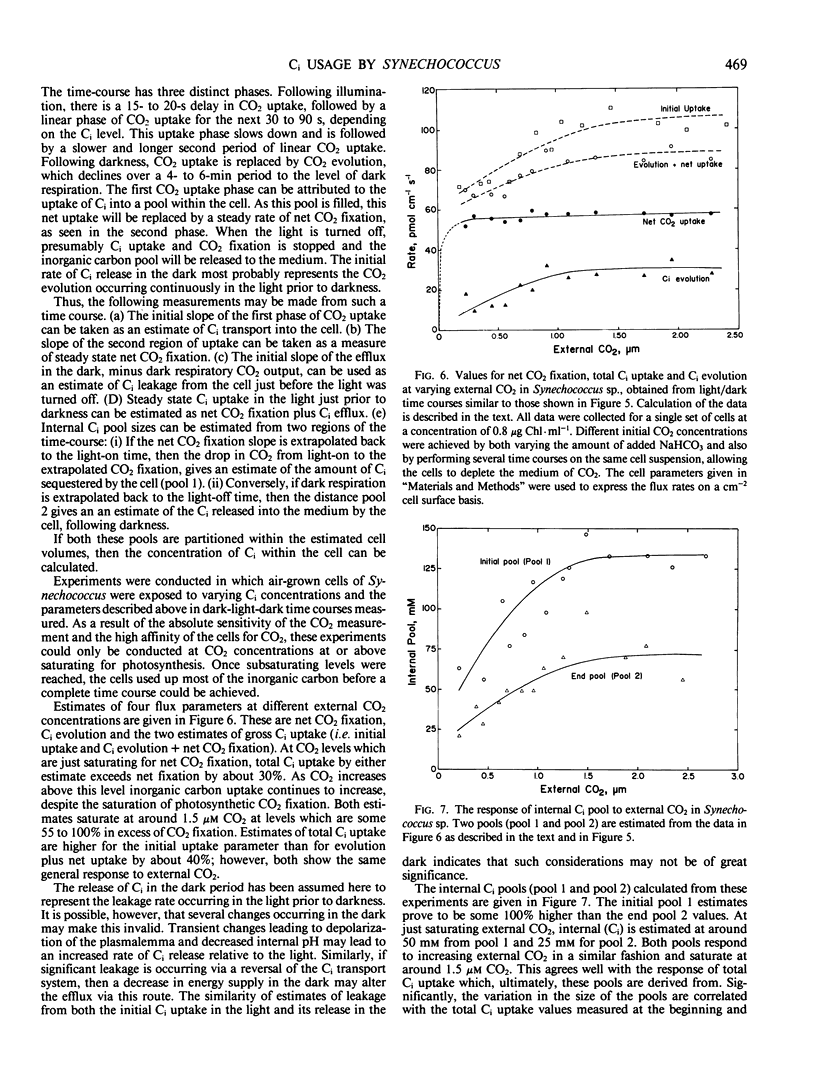
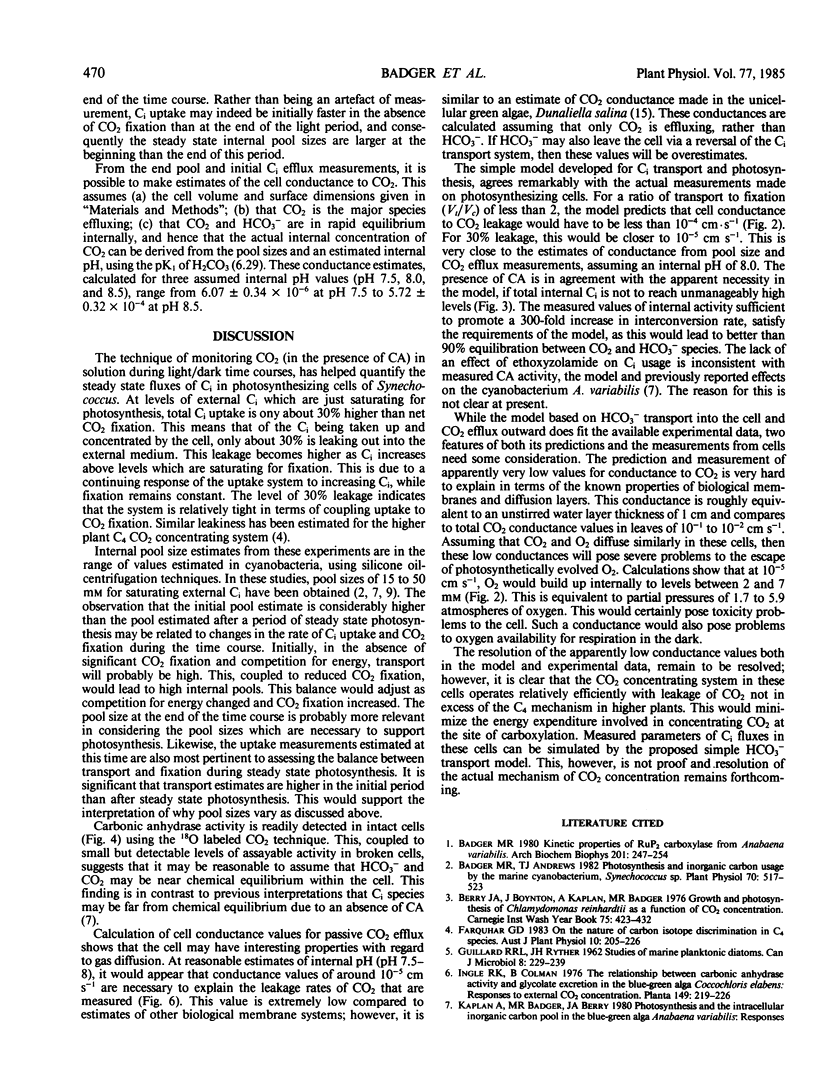
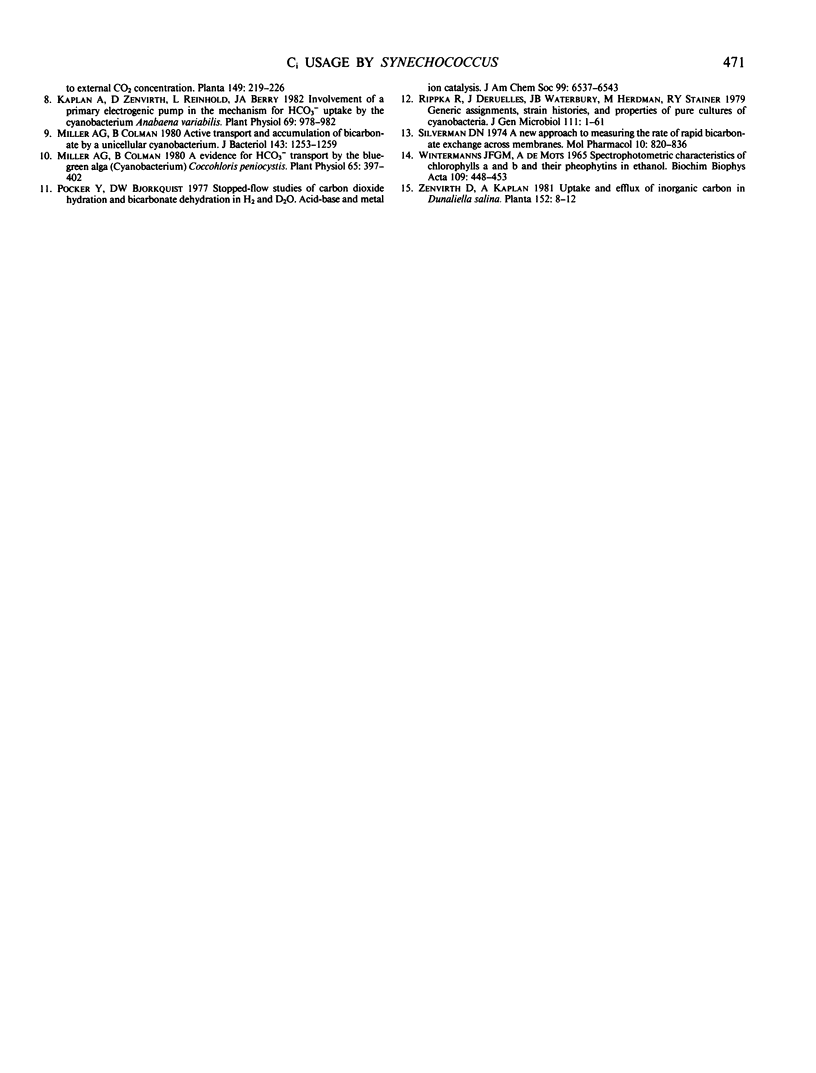
Selected References
These references are in PubMed. This may not be the complete list of references from this article.
- Badger M. R., Andrews T. J. Photosynthesis and Inorganic Carbon Usage by the Marine Cyanobacterium, Synechococcus sp. Plant Physiol. 1982 Aug;70(2):517–523. doi: 10.1104/pp.70.2.517. [DOI] [PMC free article] [PubMed] [Google Scholar]
- Badger M. R. Kinetic properties of ribulose 1,5-bisphosphate carboxylase/oxygenase from Anabaena variabilis. Arch Biochem Biophys. 1980 Apr 15;201(1):247–254. doi: 10.1016/0003-9861(80)90509-3. [DOI] [PubMed] [Google Scholar]
- GUILLARD R. R., RYTHER J. H. Studies of marine planktonic diatoms. I. Cyclotella nana Hustedt, and Detonula confervacea (cleve) Gran. Can J Microbiol. 1962 Apr;8:229–239. doi: 10.1139/m62-029. [DOI] [PubMed] [Google Scholar]
- Kaplan A., Zenvirth D., Reinhold L., Berry J. A. Involvement of a Primary Electrogenic Pump in the Mechanism for HCO(3) Uptake by the Cyanobacterium Anabaena variabilis. Plant Physiol. 1982 Apr;69(4):978–982. doi: 10.1104/pp.69.4.978. [DOI] [PMC free article] [PubMed] [Google Scholar]
- Kaplan H. S. Essentials of staging and management of the malignant lymphomas. Semin Roentgenol. 1980 Jul;15(3):219–226. doi: 10.1016/0037-198x(80)90048-6. [DOI] [PubMed] [Google Scholar]
- Miller A. G., Colman B. Active transport and accumulation of bicarbonate by a unicellular cyanobacterium. J Bacteriol. 1980 Sep;143(3):1253–1259. doi: 10.1128/jb.143.3.1253-1259.1980. [DOI] [PMC free article] [PubMed] [Google Scholar]
- Miller A. G., Colman B. Evidence for HCO(3) Transport by the Blue-Green Alga (Cyanobacterium) Coccochloris peniocystis. Plant Physiol. 1980 Feb;65(2):397–402. doi: 10.1104/pp.65.2.397. [DOI] [PMC free article] [PubMed] [Google Scholar]
- Wintermans J. F., de Mots A. Spectrophotometric characteristics of chlorophylls a and b and their pheophytins in ethanol. Biochim Biophys Acta. 1965 Nov 29;109(2):448–453. doi: 10.1016/0926-6585(65)90170-6. [DOI] [PubMed] [Google Scholar]