Abstract
Mitochondria isolated from pea leaves (Pisum sativum L.) readily oxidized malate and glycine as substrates. The addition of glycine to mitochondria oxidizing malate in state 3 diminished the rate of malate oxidation. When glycine was added to mitochondria oxidizing malate in state 4, however, the rate of malate oxidation was either unaffected or stimulated. The reason both glycine and malate can be metabolized in state 4 appears to be that malate only used part of the electron transport capacity available in these mitochondria in this state. The remaining electron transport capacity was used by glycine, thus allowing both substrates to be oxidized simultaneously. This can be explained by differential use of two NADH dehydrogenases by glycine and malate and an increase in alternate oxidase activity upon glycine addition. These results help explain why photorespiratory glycine oxidation and its associated demand for NAD do not inhibit citric acid cycle function in leaves.
Full text
PDF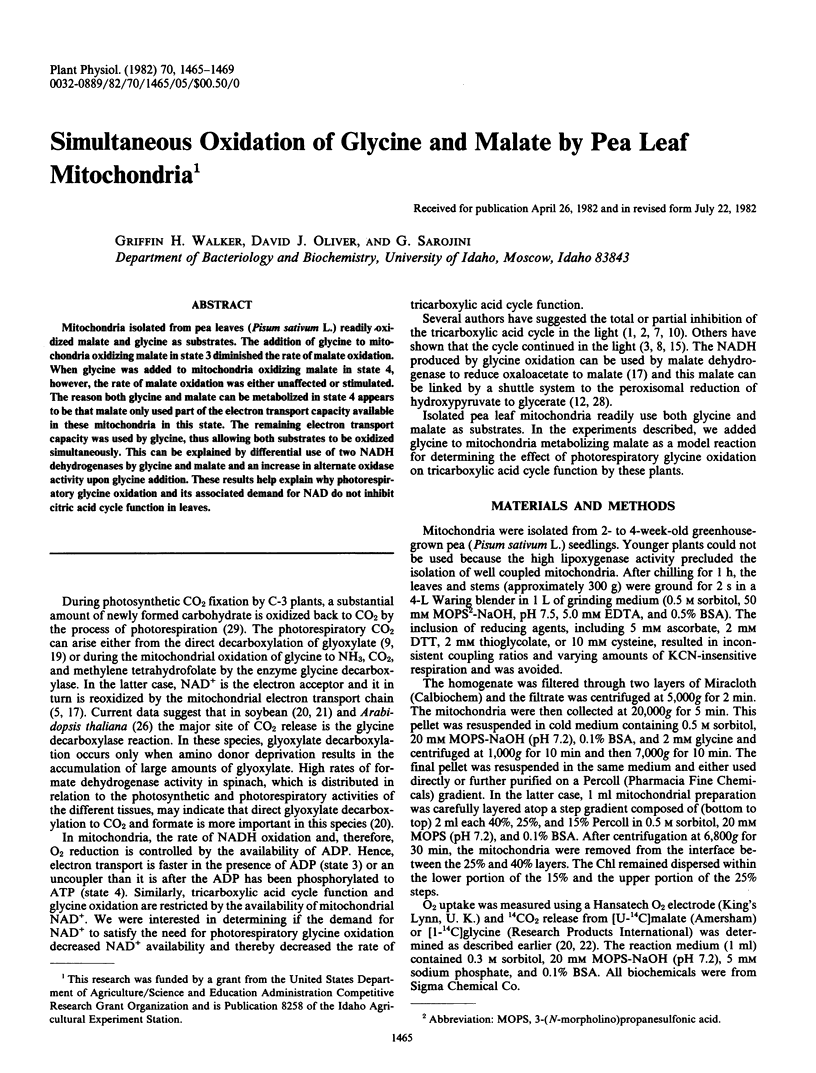
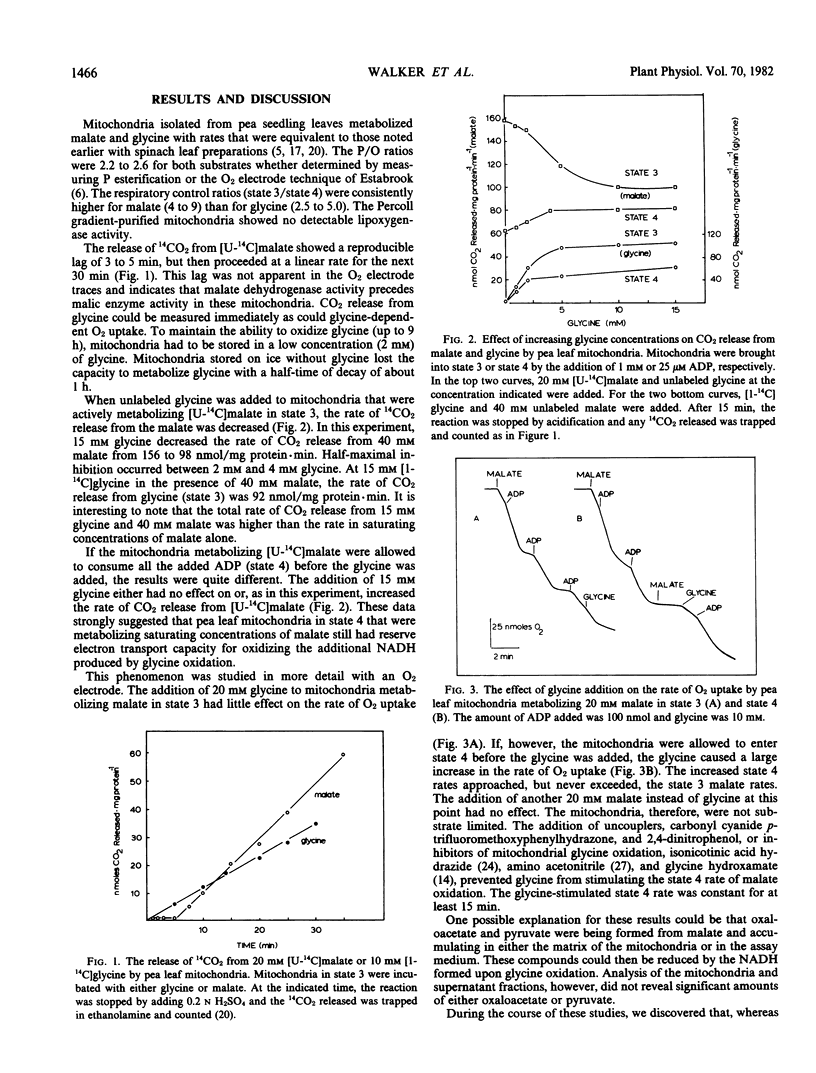
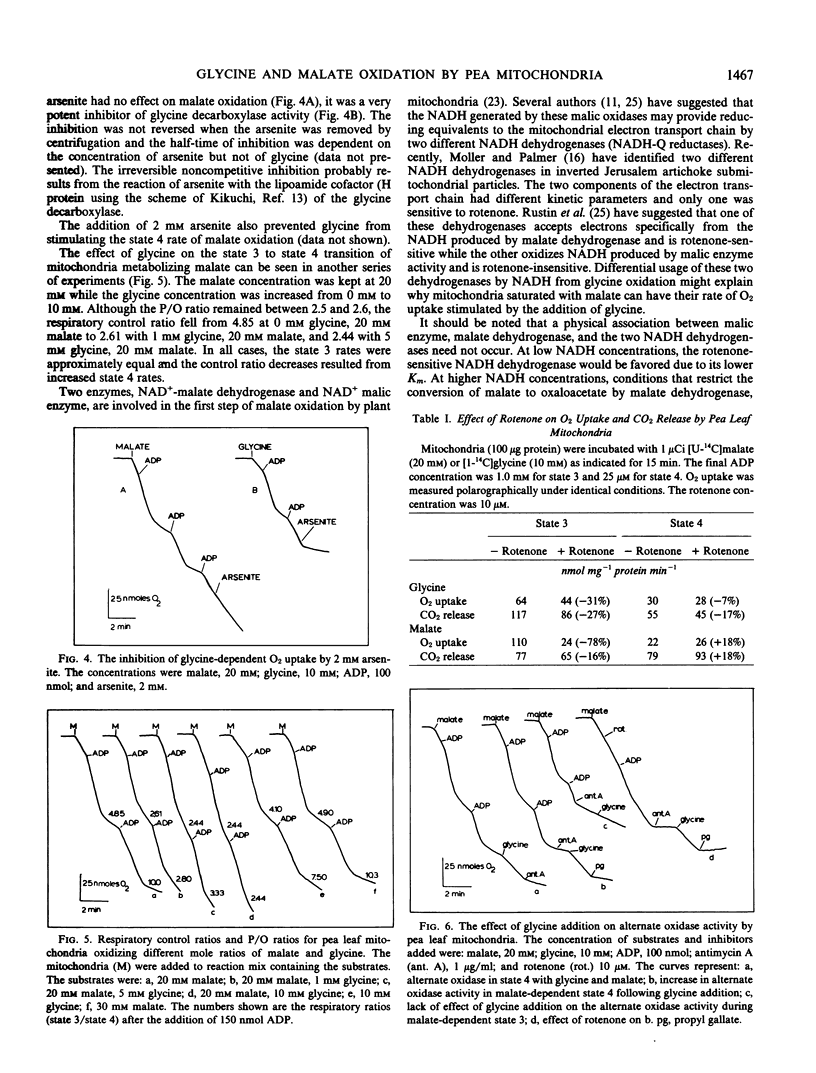
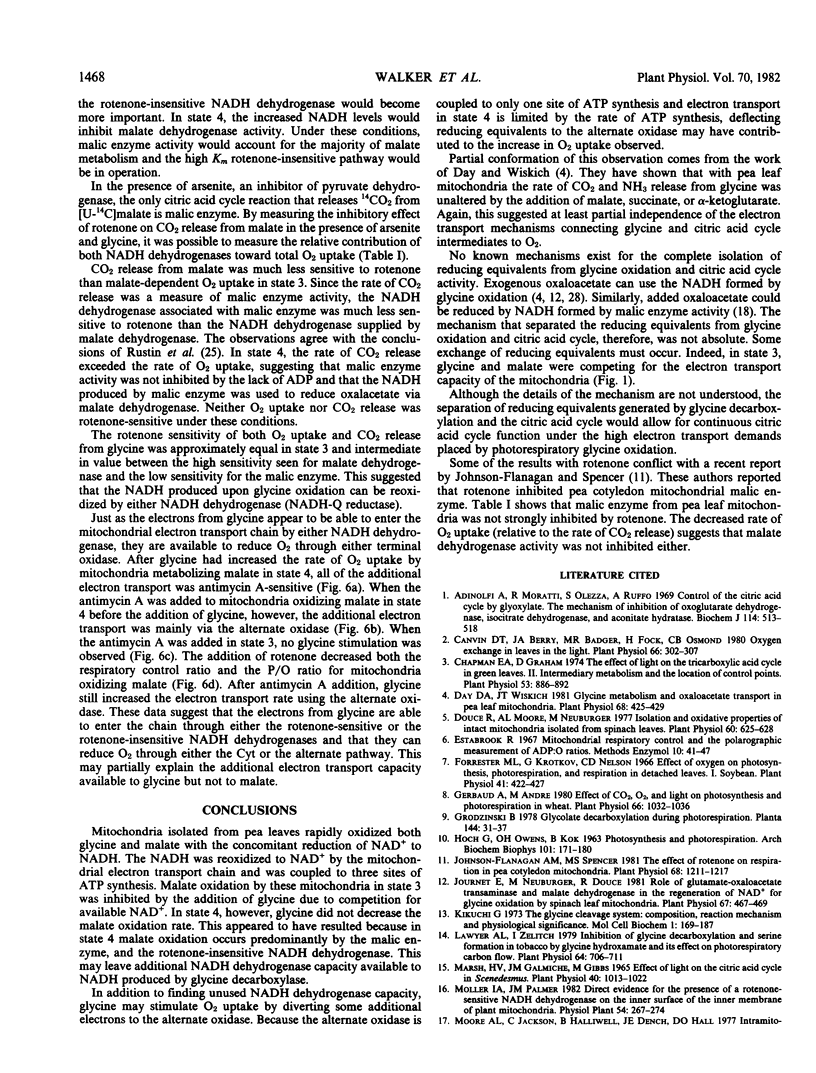
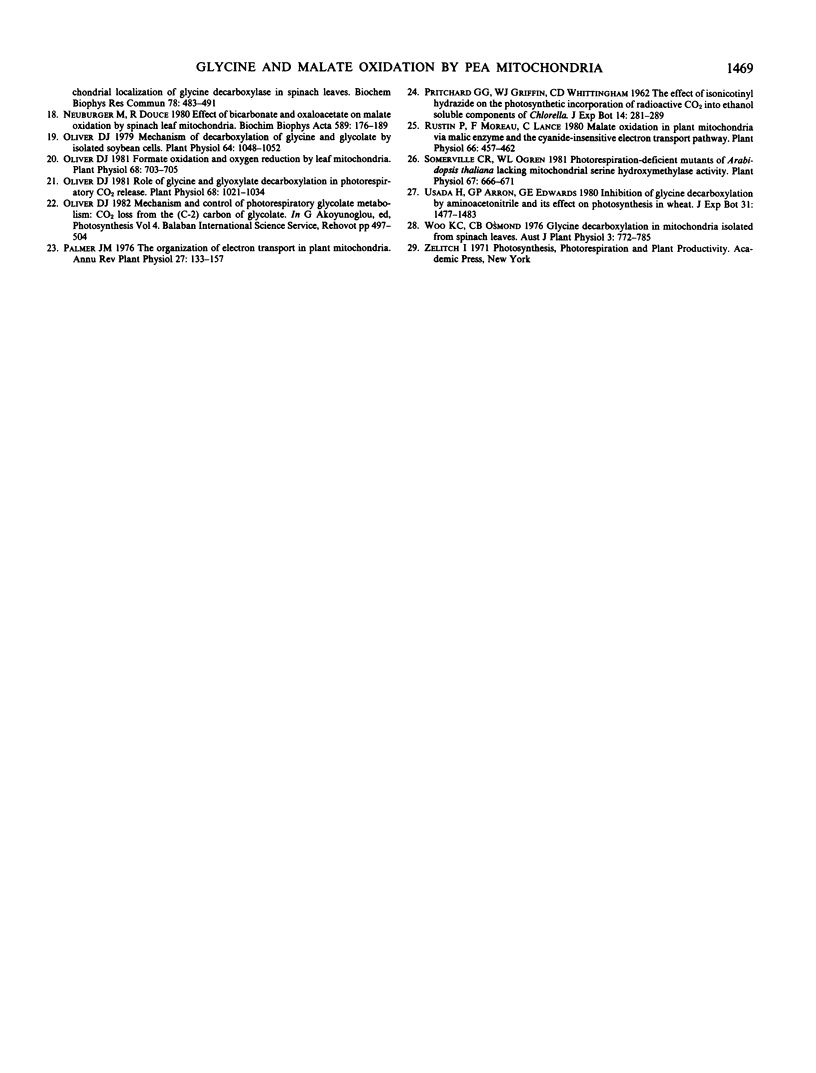
Selected References
These references are in PubMed. This may not be the complete list of references from this article.
- Adinolfi A., Moratti R., Olezza S., Ruffo A. Control of the citric acid cycle by glyoxylate. The mechanism of inhibition of oxoglutarate dehydrogenase, isocitrate dehydrogenase and aconitate hydratase. Biochem J. 1969 Sep;114(3):513–518. doi: 10.1042/bj1140513. [DOI] [PMC free article] [PubMed] [Google Scholar]
- Canvin D. T., Berry J. A., Badger M. R., Fock H., Osmond C. B. Oxygen exchange in leaves in the light. Plant Physiol. 1980 Aug;66(2):302–307. doi: 10.1104/pp.66.2.302. [DOI] [PMC free article] [PubMed] [Google Scholar]
- Chapman E. A., Graham D. The Effect of Light on the Tricarboxylic Acid Cycle in Green Leaves: II. Intermediary Metabolism and the Location of Control Points. Plant Physiol. 1974 Jun;53(6):886–892. doi: 10.1104/pp.53.6.886. [DOI] [PMC free article] [PubMed] [Google Scholar]
- Day D. A., Wiskich J. T. Glycine metabolism and oxalacetate transport by pea leaf mitochondria. Plant Physiol. 1981 Aug;68(2):425–429. doi: 10.1104/pp.68.2.425. [DOI] [PMC free article] [PubMed] [Google Scholar]
- Douce R., Moore A. L., Neuburger M. Isolation and oxidative properties of intact mitochondria isolated from spinach leaves. Plant Physiol. 1977 Oct;60(4):625–628. doi: 10.1104/pp.60.4.625. [DOI] [PMC free article] [PubMed] [Google Scholar]
- Forrester M. L., Krotkov G., Nelson C. D. Effect of oxygen on photosynthesis, photorespiration and respiration in detached leaves. I. Soybean. Plant Physiol. 1966 Mar;41(3):422–427. doi: 10.1104/pp.41.3.422. [DOI] [PMC free article] [PubMed] [Google Scholar]
- Gerbaud A., André M. Effect of CO(2), O(2), and Light on Photosynthesis and Photorespiration in Wheat. Plant Physiol. 1980 Dec;66(6):1032–1036. doi: 10.1104/pp.66.6.1032. [DOI] [PMC free article] [PubMed] [Google Scholar]
- HOCH G., OWENS O. V., KOK B. Photosynthesis and respiration. Arch Biochem Biophys. 1963 Apr;101:171–180. doi: 10.1016/0003-9861(63)90547-2. [DOI] [PubMed] [Google Scholar]
- Johnson-Flanagan A. M., Spencer M. S. The effect of rotenone on respiration in pea cotyledon mitochondria. Plant Physiol. 1981 Dec;68(6):1211–1217. doi: 10.1104/pp.68.6.1211. [DOI] [PMC free article] [PubMed] [Google Scholar]
- Journet E. P., Neuburger M., Douce R. Role of Glutamate-oxaloacetate Transaminase and Malate Dehydrogenase in the Regeneration of NAD for Glycine Oxidation by Spinach leaf Mitochondria. Plant Physiol. 1981 Mar;67(3):467–469. doi: 10.1104/pp.67.3.467. [DOI] [PMC free article] [PubMed] [Google Scholar]
- Kikuchi G. The glycine cleavage system: composition, reaction mechanism, and physiological significance. Mol Cell Biochem. 1973 Jun 27;1(2):169–187. doi: 10.1007/BF01659328. [DOI] [PubMed] [Google Scholar]
- Lawyer A. L., Zelitch I. Inhibition of glycine decarboxylation and serine formation in tobacco by glycine hydroxamate and its effect on photorespiratory carbon flow. Plant Physiol. 1979 Nov;64(5):706–711. doi: 10.1104/pp.64.5.706. [DOI] [PMC free article] [PubMed] [Google Scholar]
- Marsh H. V., Galmiche J. M., Gibbs M. Effect of Light on the Tricarboxylic Acid Cycle in Scenedesmus. Plant Physiol. 1965 Nov;40(6):1013–1022. doi: 10.1104/pp.40.6.1013. [DOI] [PMC free article] [PubMed] [Google Scholar]
- Oliver D. J. Mechanism of decarboxylation of glycine and glycolate by isolated soybean cells. Plant Physiol. 1979 Dec;64(6):1048–1052. doi: 10.1104/pp.64.6.1048. [DOI] [PMC free article] [PubMed] [Google Scholar]
- Oliver D. J. Role of Glycine and Glyoxylate Decarboxylation in Photorespiratory CO(2) Release. Plant Physiol. 1981 Nov;68(5):1031–1034. doi: 10.1104/pp.68.5.1031. [DOI] [PMC free article] [PubMed] [Google Scholar]
- Somerville C. R., Ogren W. L. Photorespiration-deficient Mutants of Arabidopsis thaliana Lacking Mitochondrial Serine Transhydroxymethylase Activity. Plant Physiol. 1981 Apr;67(4):666–671. doi: 10.1104/pp.67.4.666. [DOI] [PMC free article] [PubMed] [Google Scholar]