Abstract
Needles from phosphorus deficient seedlings of Pinus radiata D. Don grown for 8 weeks at either 330 or 660 microliters CO2 per liter displayed chlorophyll a fluorescence induction kinetics characteristic of structural changes within the thylakoid chloroplast membrane, i.e. constant yield fluorescence (FO) was increased and induced fluorescence ([FP-FI]/FO) was reduced. The effect was greatest in the undroughted plants grown at 660 μl CO2 L−1. By week 22 at 330 μl CO2 L−1 acclimation to P deficiency had occurred as shown by the similarity in the fluorescence characteristics and maximum rates of photosynthesis of the needles from the two P treatments. However, acclimation did not occur in the plants grown at 660 μl CO2 L−1. The light saturated rate of photosynthesis of needles with adequate P was higher at 660 μl CO2 L−1 than at 330 μl CO2 L−1, whereas photosynthesis of P deficient plants showed no increase when grown at the higher CO2 concentration. The average growth increase due to CO2 enrichment was 14% in P deficient plants and 32% when P was adequate. In drought stressed plants grown at 330 μl CO2 L−1, there was a reduction in the maximal rate of quenching of fluorescence (RQ) after the major peak. Constant yield fluorescence was unaffected but induced fluorescence was lower. These results indicate that electron flow subsequent to photosystem II was affected by drought stress. At 660 μl CO2 L−1 this response was eliminated showing that CO2 enrichment improved the ability of the seedlings to acclimate to drought stress. The average growth increase with CO2 enrichment was 37% in drought stressed plants and 19% in unstressed plants.
Full text
PDF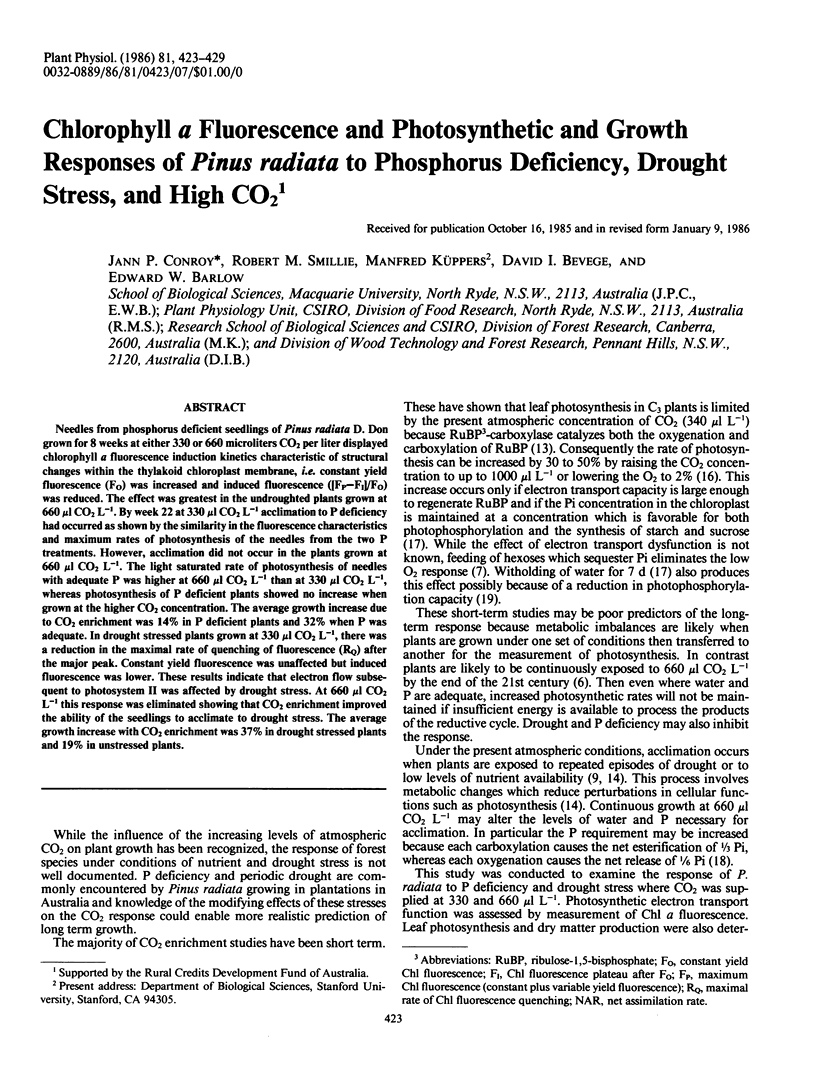
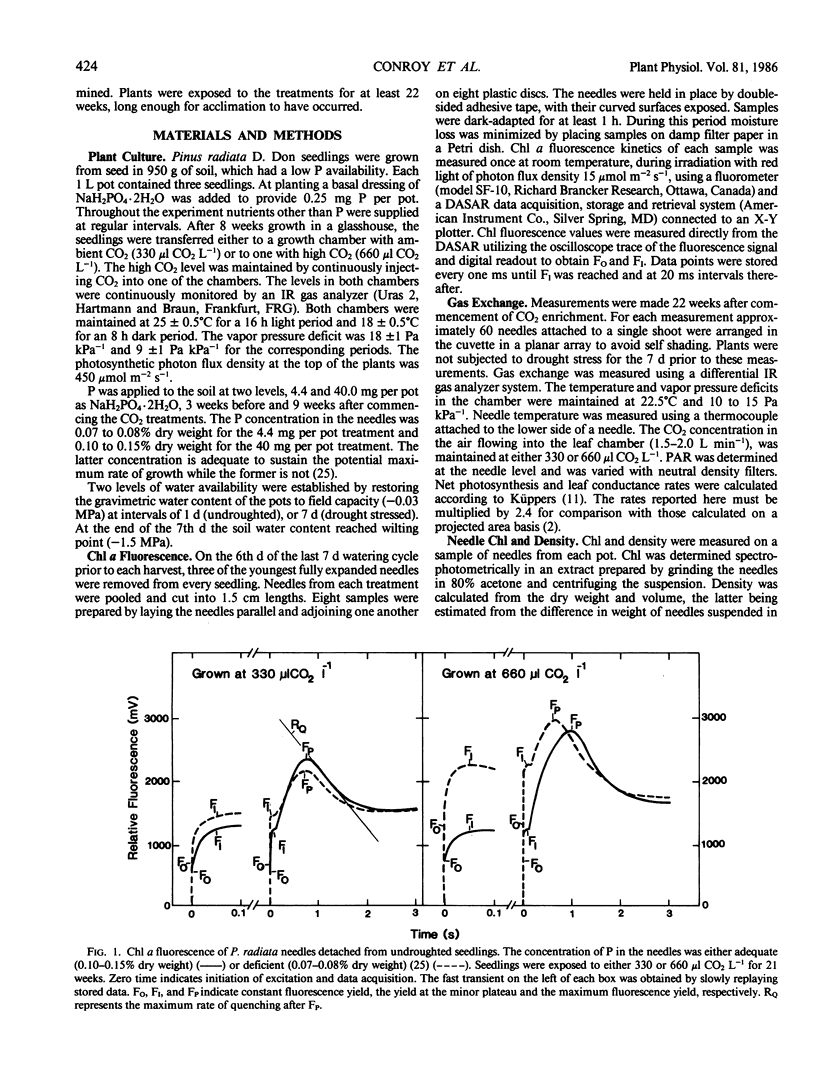
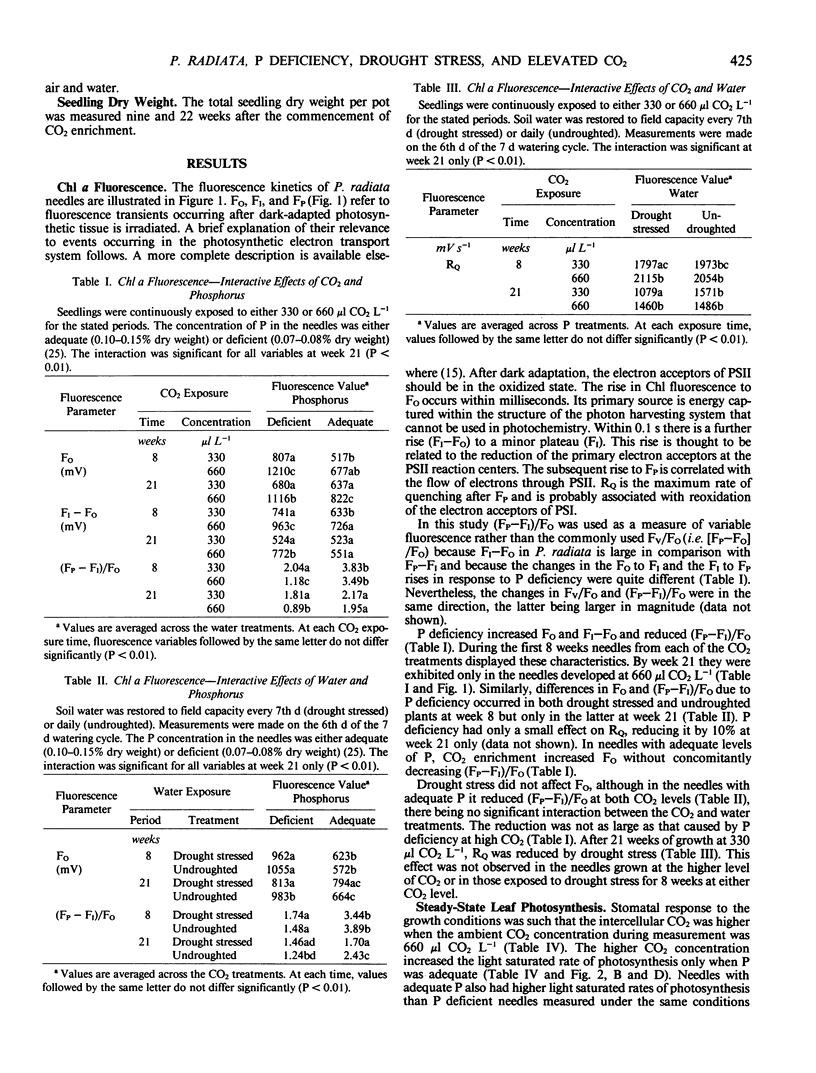
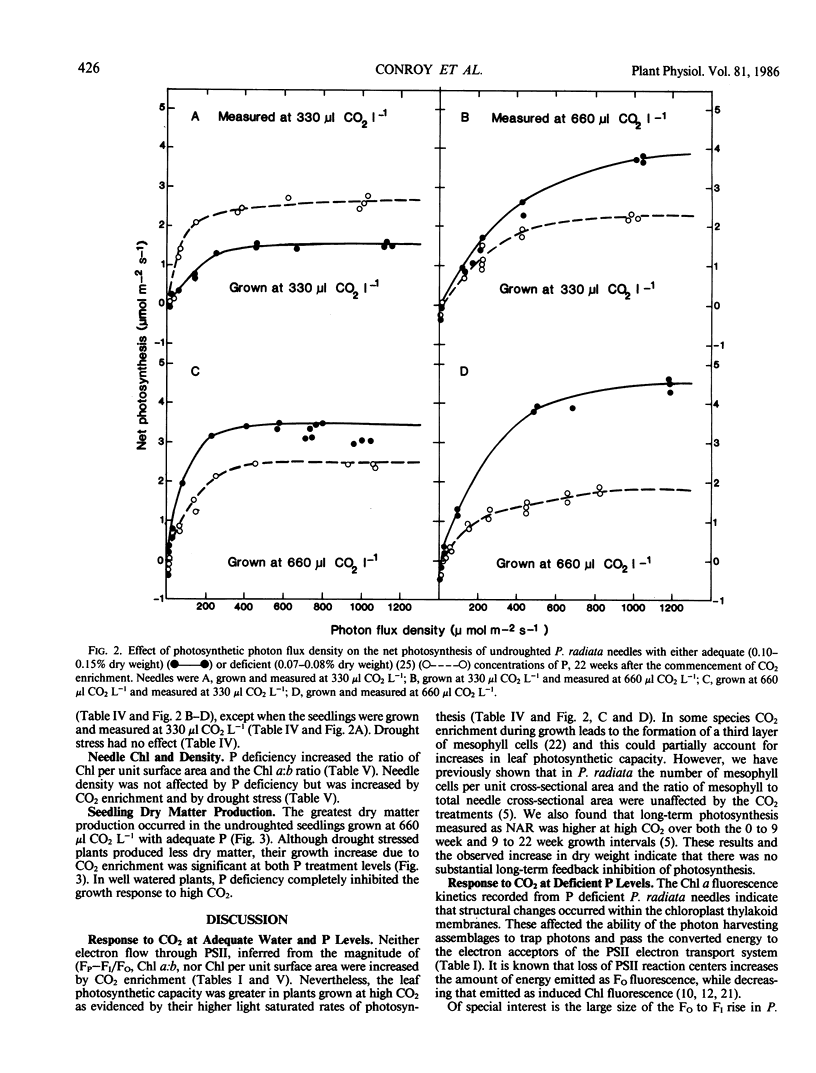
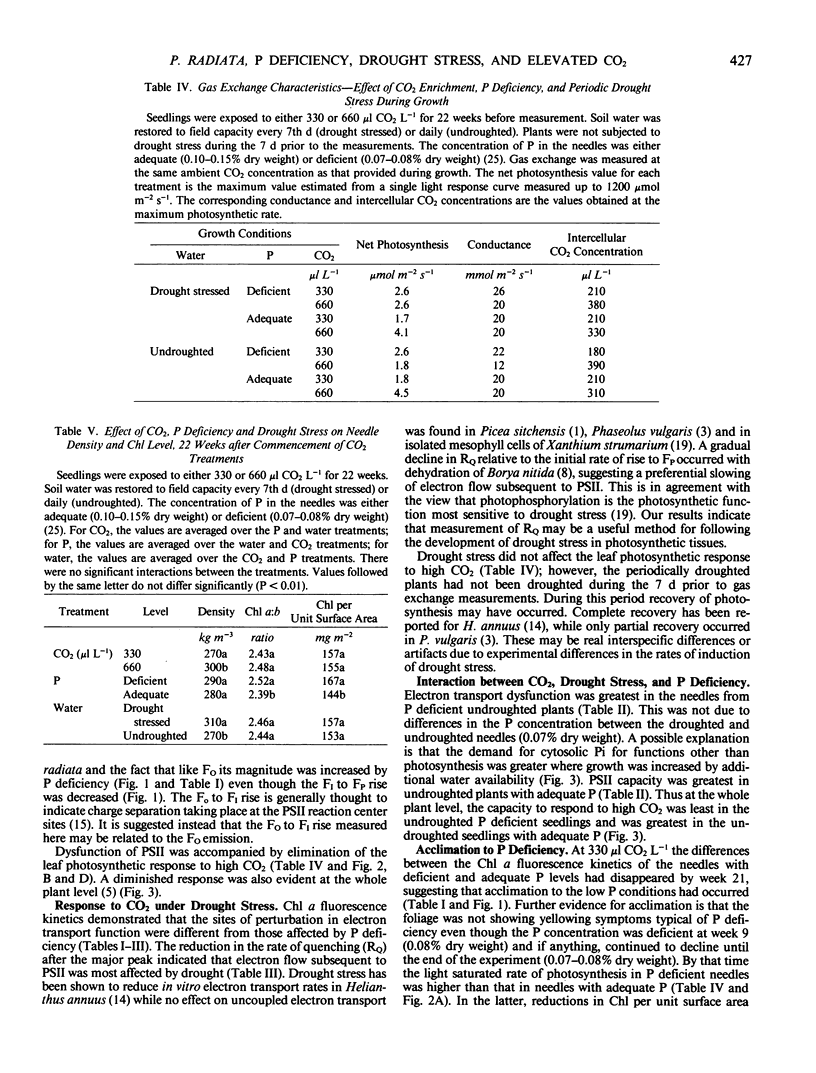
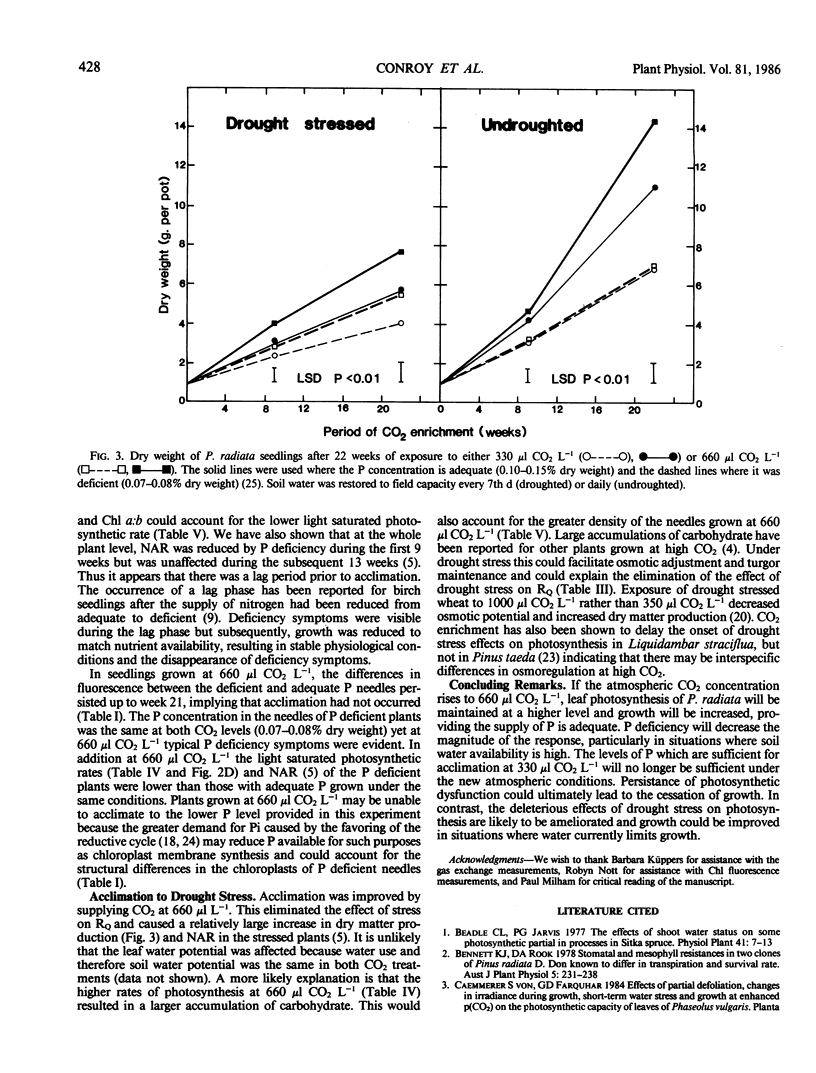
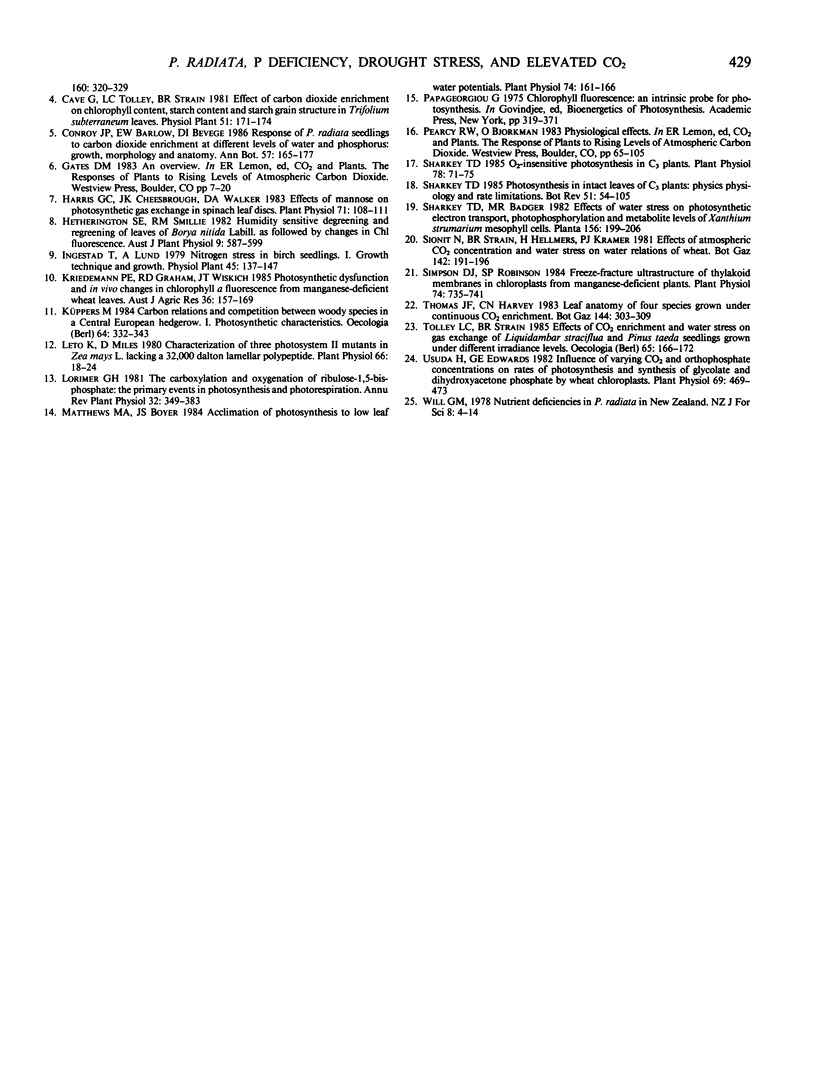
Selected References
These references are in PubMed. This may not be the complete list of references from this article.
- Harris G. C., Cheesbrough J. K., Walker D. A. Effects of mannose on photosynthetic gas exchange in spinach leaf discs. Plant Physiol. 1983 Jan;71(1):108–111. doi: 10.1104/pp.71.1.108. [DOI] [PMC free article] [PubMed] [Google Scholar]
- Leto K. J., Miles D. Characterization of Three Photosystem II Mutants in Zea mays L. Lacking a 32,000 Dalton Lamellar Polypeptide. Plant Physiol. 1980 Jul;66(1):18–24. doi: 10.1104/pp.66.1.18. [DOI] [PMC free article] [PubMed] [Google Scholar]
- Matthews M. A., Boyer J. S. Acclimation of photosynthesis to low leaf water potentials. Plant Physiol. 1984 Jan;74(1):161–166. doi: 10.1104/pp.74.1.161. [DOI] [PMC free article] [PubMed] [Google Scholar]
- Sharkey T. D. O(2)-insensitive photosynthesis in c(3) plants : its occurrence and a possible explanation. Plant Physiol. 1985 May;78(1):71–75. doi: 10.1104/pp.78.1.71. [DOI] [PMC free article] [PubMed] [Google Scholar]
- Simpson D. J., Robinson S. P. Freeze-fracture ultrastructure of thylakoid membranes in chloroplasts from manganese-deficient plants. Plant Physiol. 1984 Mar;74(3):735–741. doi: 10.1104/pp.74.3.735. [DOI] [PMC free article] [PubMed] [Google Scholar]
- Usuda H., Edwards G. E. Influence of Varying CO(2) and Orthophosphate Concentrations on Rates of Photosynthesis, and Synthesis of Glycolate and Dihydroxyacetone Phosphate by Wheat Chloroplasts. Plant Physiol. 1982 Feb;69(2):469–473. doi: 10.1104/pp.69.2.469. [DOI] [PMC free article] [PubMed] [Google Scholar]