Abstract
Since environmental pollution by potentially acidic gases such as SO2 causes proton release inside leaf tissues, homogenates of needles of spruce (Picea abies) and fir (Abies alba) and of leaves of spinach (Spinacia oleracea) and barley (Hordeum vulgare) were titrated and buffer capacities were determined as a function of pH. Titration curves of barley leaves were compared with titration curves of barley mesophyll protoplasts. From the protoplasts, chloroplasts and vacuoles were isolated and subjected to titration experiments. From the titration curves, the intracellular distribution of buffering capacities could be deduced. Buffering was strongly pH-dependent. It was high at the extremes of pH but still significant close to neutrality. Owing to its large size, the vacuole was mainly responsible for cellular buffering. However, on a unit volume basis, the cytoplasm was much more strongly buffered than the vacuole. Potentially acidic gases are trapped in the anionic form. They release protons when trapped. The magnitude of diffusion gradients from the atmosphere into the cells, which determines flux, depends on intracellular pH. In the light, the chloroplast stroma, as the most alkaline leaf compartment, has the highest trapping potential. Acidification of the chloroplast stroma inhibits photosynthesis. The trapping potential of the chloroplast is followed by that of the cytosol. Compared with the cytoplasm, the vacuole possesses little trapping potential in spite of its large size. It is particularly small in the acidic vacuoles of conifer needles. In the physiological pH range (slightly above neutrality), chloroplast buffering was about 1 microequivalents H+ per milligram chlorophyll per pH unit or 35 microequivalents H+ per milliliter per pH unit in barley or spinach chloroplasts. This compares with SO2-generated H+ production of somewhat more than 1 microequivalent H+ per milligram chlorophyll per hour, which results from observed SO2 uptake of leaves when stomata were open and the atmospheric SO2 concentration was 0.4 microliters per liter (GE Taylor Jr, DT Tingey 1983 Plant Physiol 72: 237-244). At lower SO2 concentrations, similar H+ generation inside the cells requires correspondingly longer exposure times.
Full text
PDF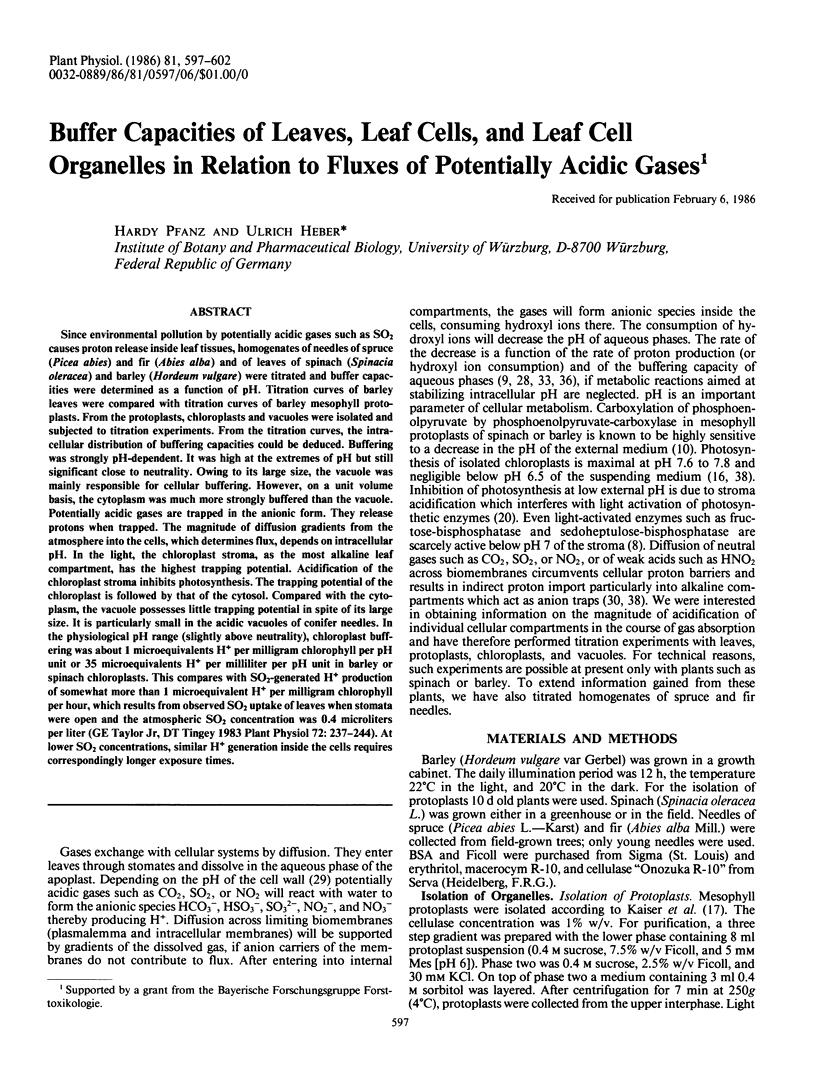
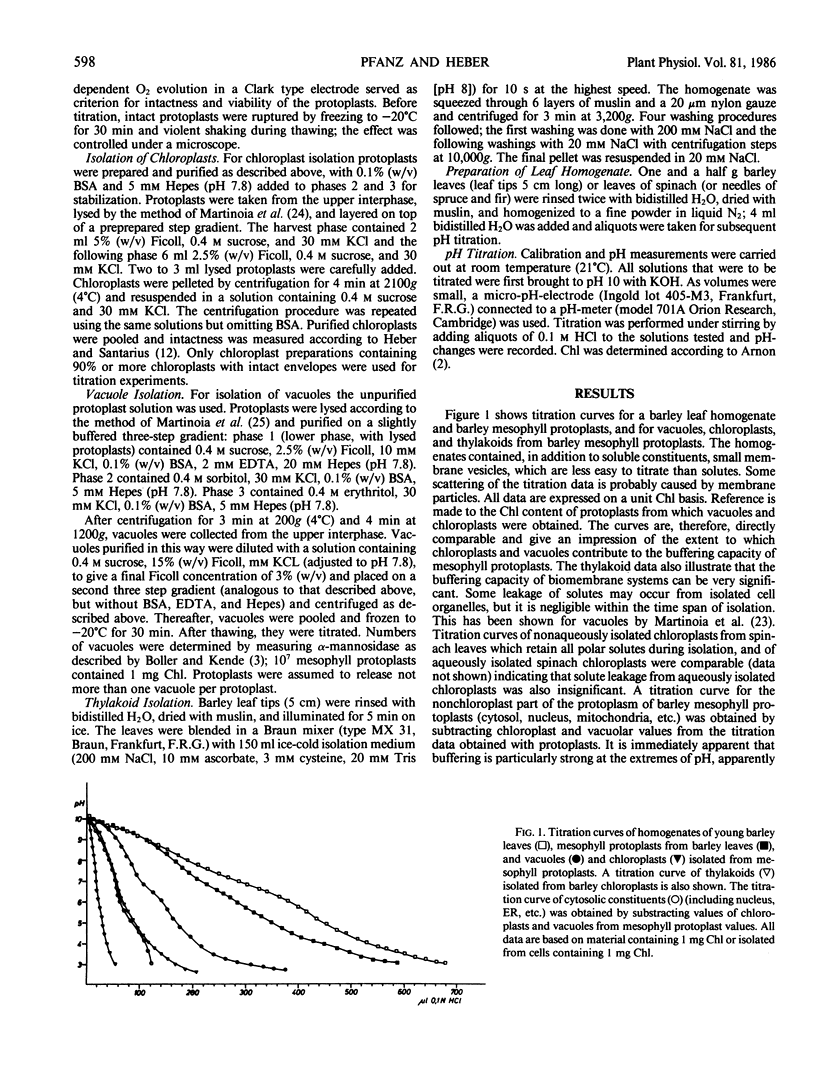
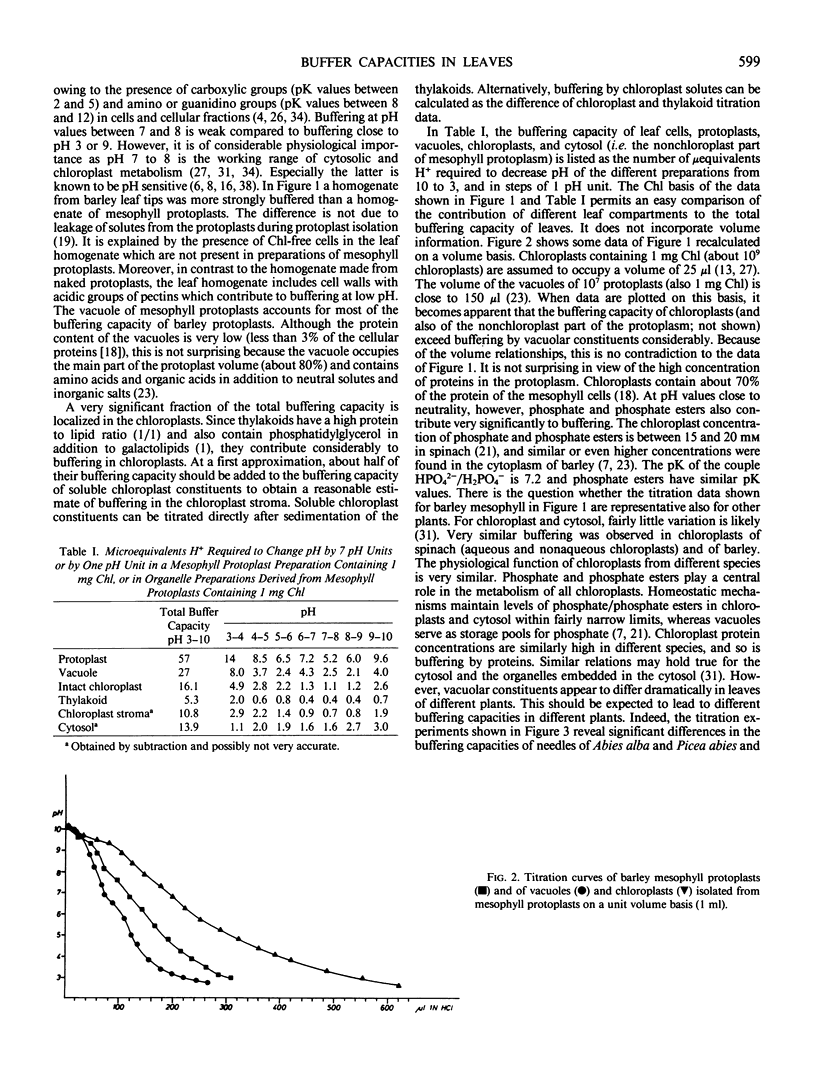
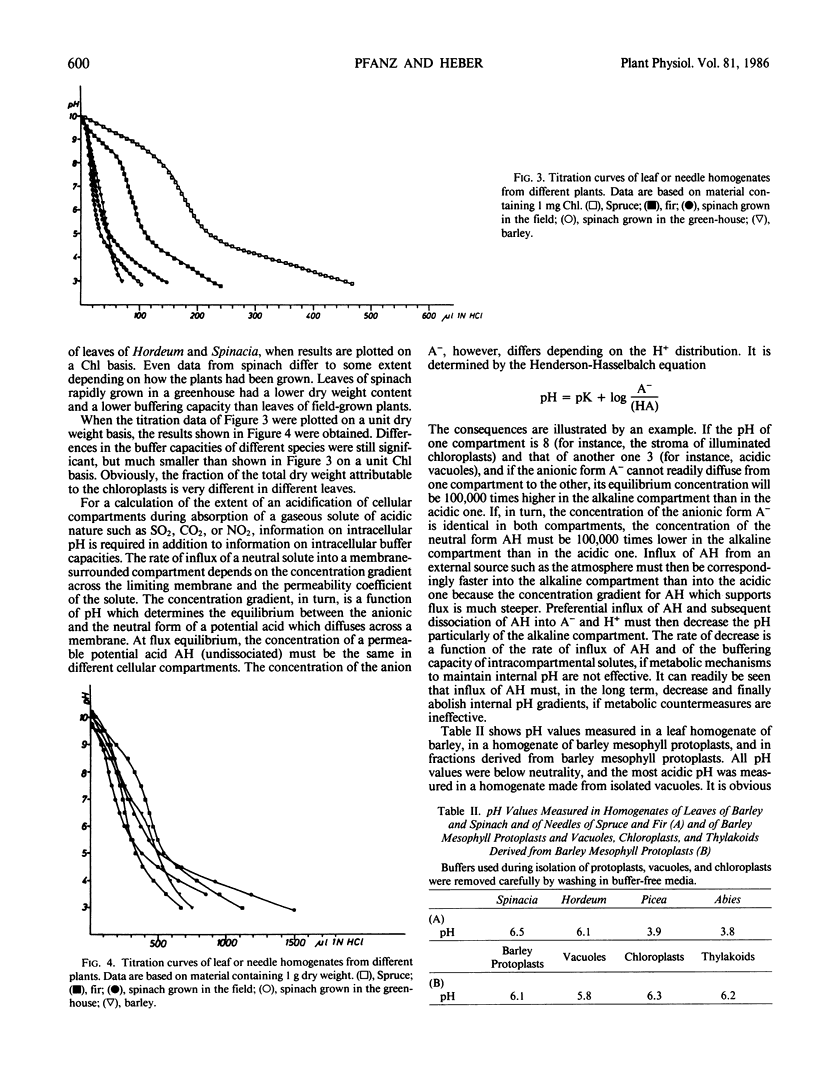
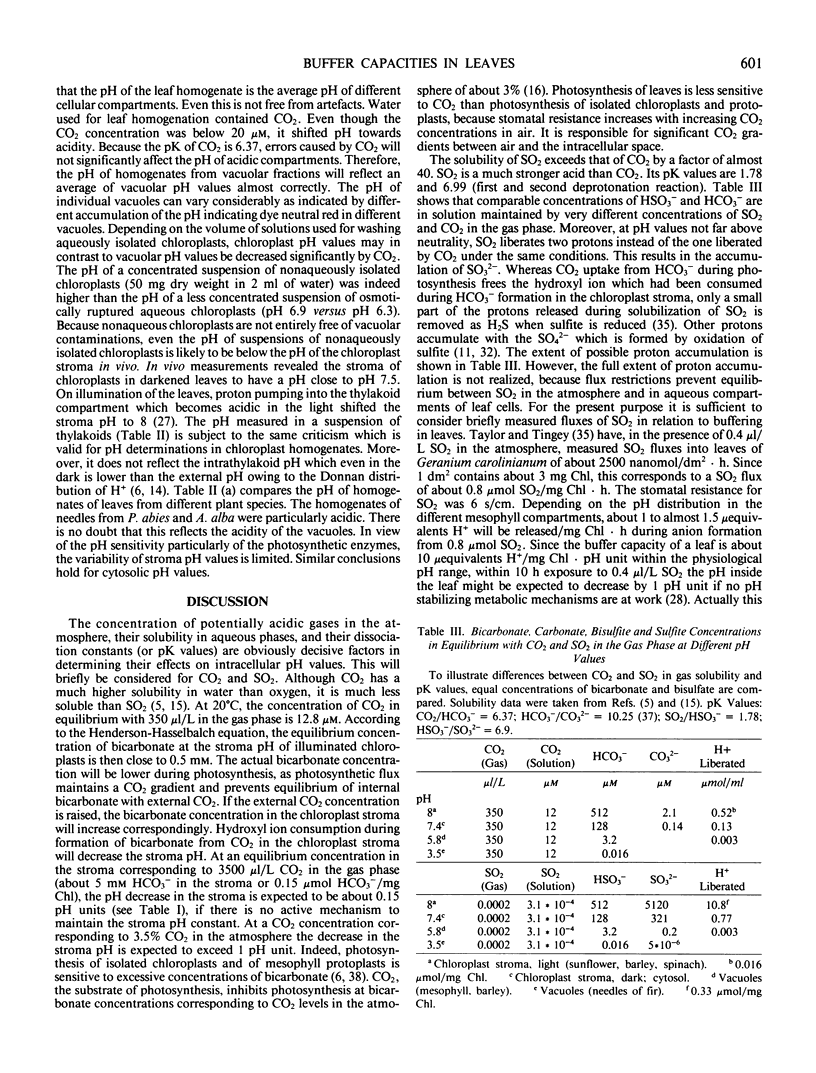
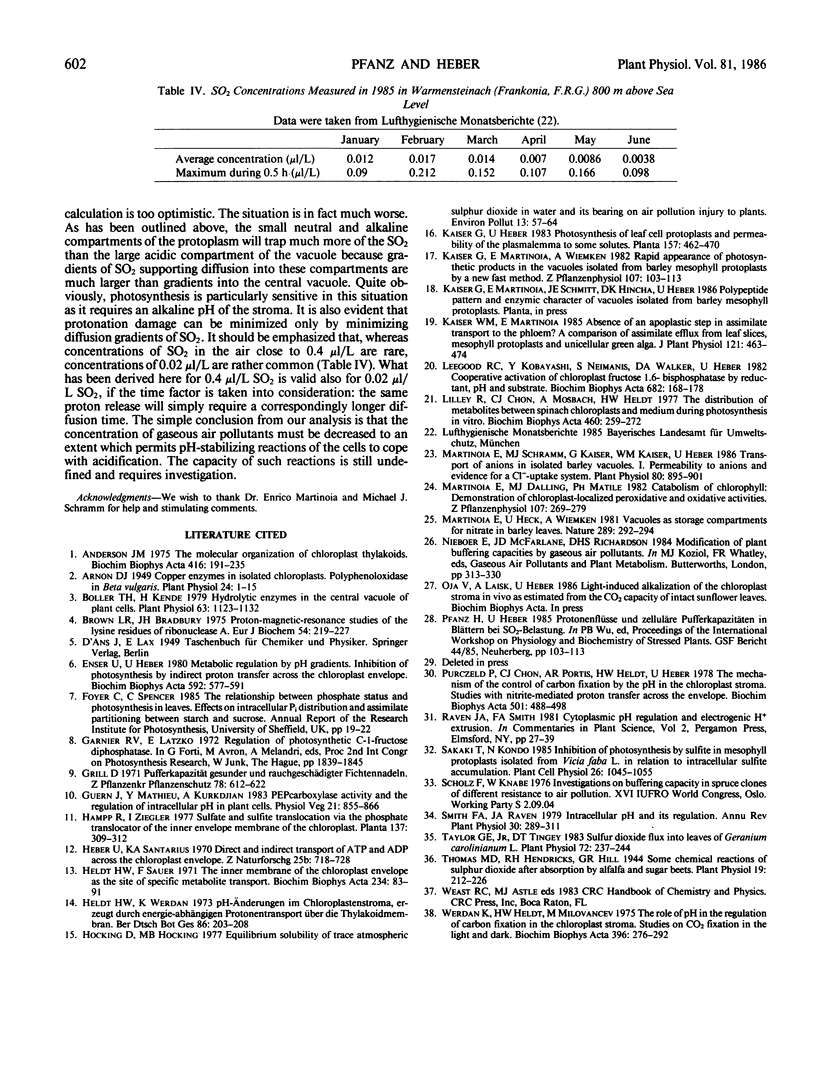
Selected References
These references are in PubMed. This may not be the complete list of references from this article.
- Anderson J. M. The molecular organization of chloroplast thylakoids. Biochim Biophys Acta. 1975 Aug 15;416(2):191–235. doi: 10.1016/0304-4173(75)90007-5. [DOI] [PubMed] [Google Scholar]
- Arnon D. I. COPPER ENZYMES IN ISOLATED CHLOROPLASTS. POLYPHENOLOXIDASE IN BETA VULGARIS. Plant Physiol. 1949 Jan;24(1):1–15. doi: 10.1104/pp.24.1.1. [DOI] [PMC free article] [PubMed] [Google Scholar]
- Boller T., Kende H. Hydrolytic enzymes in the central vacuole of plant cells. Plant Physiol. 1979 Jun;63(6):1123–1132. doi: 10.1104/pp.63.6.1123. [DOI] [PMC free article] [PubMed] [Google Scholar]
- Brown L. R., Bradbury J. H. Proton-magnetic-resonance studies of the lysine residues of ribonuclease A. Eur J Biochem. 1975 May;54(1):219–227. doi: 10.1111/j.1432-1033.1975.tb04131.x. [DOI] [PubMed] [Google Scholar]
- Enser U., Heber U. Metabolic regulation by pH gradients. Inhibition of photosynthesis by indirect proton transfer across the chloroplast envelope. Biochim Biophys Acta. 1980 Oct 3;592(3):577–591. doi: 10.1016/0005-2728(80)90102-4. [DOI] [PubMed] [Google Scholar]
- Heber U., Santarius K. A. Direct and indirect transfer of ATP and ADP across the chloroplast envelope. Z Naturforsch B. 1970 Jul;25(7):718–728. doi: 10.1515/znb-1970-0714. [DOI] [PubMed] [Google Scholar]
- Heldt H. W., Sauer F. The inner membrane of the chloroplast envelope as the site of specific metabolite transport. Biochim Biophys Acta. 1971 Apr 6;234(1):83–91. doi: 10.1016/0005-2728(71)90133-2. [DOI] [PubMed] [Google Scholar]
- Lilley R. M., Chon C. J., Mosbach A., Heldt H. W. The distribution of metabolites between spinach chloroplasts and medium during photosynthesis in vitro. Biochim Biophys Acta. 1977 May 11;460(2):259–272. doi: 10.1016/0005-2728(77)90212-2. [DOI] [PubMed] [Google Scholar]
- Martinoia E., Schramm M. J., Kaiser G., Kaiser W. M., Heber U. Transport of anions in isolated barley vacuoles : I. Permeability to anions and evidence for a cl-uptake system. Plant Physiol. 1986 Apr;80(4):895–901. doi: 10.1104/pp.80.4.895. [DOI] [PMC free article] [PubMed] [Google Scholar]
- Purczeld P., Chon C. J., Portis A. R., Jr, Heldt H. W., Heber U. The mechanism of the control of carbon fixation by the pH in the chloroplast stroma. Studies with nitrite-mediated proton transfer across the envelope. Biochim Biophys Acta. 1978 Mar 13;501(3):488–498. doi: 10.1016/0005-2728(78)90116-0. [DOI] [PubMed] [Google Scholar]
- Taylor G. E., Tingey D. T. Sulfur Dioxide Flux into Leaves of Geranium carolinianum L. : Evidence for a Nonstomatal or Residual Resistance. Plant Physiol. 1983 May;72(1):237–244. doi: 10.1104/pp.72.1.237. [DOI] [PMC free article] [PubMed] [Google Scholar]
- Thomas M. D., Hendricks R. H., Hill G. R. SOME CHEMICAL REACTIONS OF SULPHUR DIOXIDE AFTER ABSORPTION BY ALFALFA AND SUGAR BEETS. Plant Physiol. 1944 Apr;19(2):212–226. doi: 10.1104/pp.19.2.212. [DOI] [PMC free article] [PubMed] [Google Scholar]
- Werdan K., Heldt H. W., Milovancev M. The role of pH in the regulation of carbon fixation in the chloroplast stroma. Studies on CO2 fixation in the light and dark. Biochim Biophys Acta. 1975 Aug 11;396(2):276–292. doi: 10.1016/0005-2728(75)90041-9. [DOI] [PubMed] [Google Scholar]