Abstract
Pulsed, time resolved photoacoustics has sufficient sensitivity to determine oxygen emission and uptake by single turnover flashes to leaves. The advantage over previous methodologies is that when combined with single turnover flashes the kinetics of the thermal and the gas signals can be resolved to 0.1 millisecond and separated. The S-state oscillations of oxygen formation are readily observed. The gas signal from common spongy leaves such as spinach (Spinacia sp.), Japanese andromeda (Pieris japonica), mock orange (Philadelphus coronarius) and viburnum (Viburnum tomentosum), after correction for instrumental rise time, show a lag of only 1 millisecond and a rise time of 5 milliseconds in the formation of oxygen. Thus a recent proposal that the formation of oxygen requires over 100 milliseconds cannot be true for choroplasts in vivo. The rapid emission is correlated with structure of the leaf. At low light flash energies a rapid gas uptake is observed. The uptake has slightly slower kinetics than oxygen evolution, and its magnitude increases with damage to the leaf. The pulse methodology shows that the uptake begins with the very first flash after dark adaption, and allows the detection of a positive signal (oxygen) on the third flash. These observations, the long wavelength of excitation (695 nanometers) and the magnitude of the signal support the contention that the gas uptake is oxygen reduction by electrons from photosystem I. These results show that important physiological aspects of a leaf can be studied by pulsed, time resolved photoacoustics.
Full text
PDF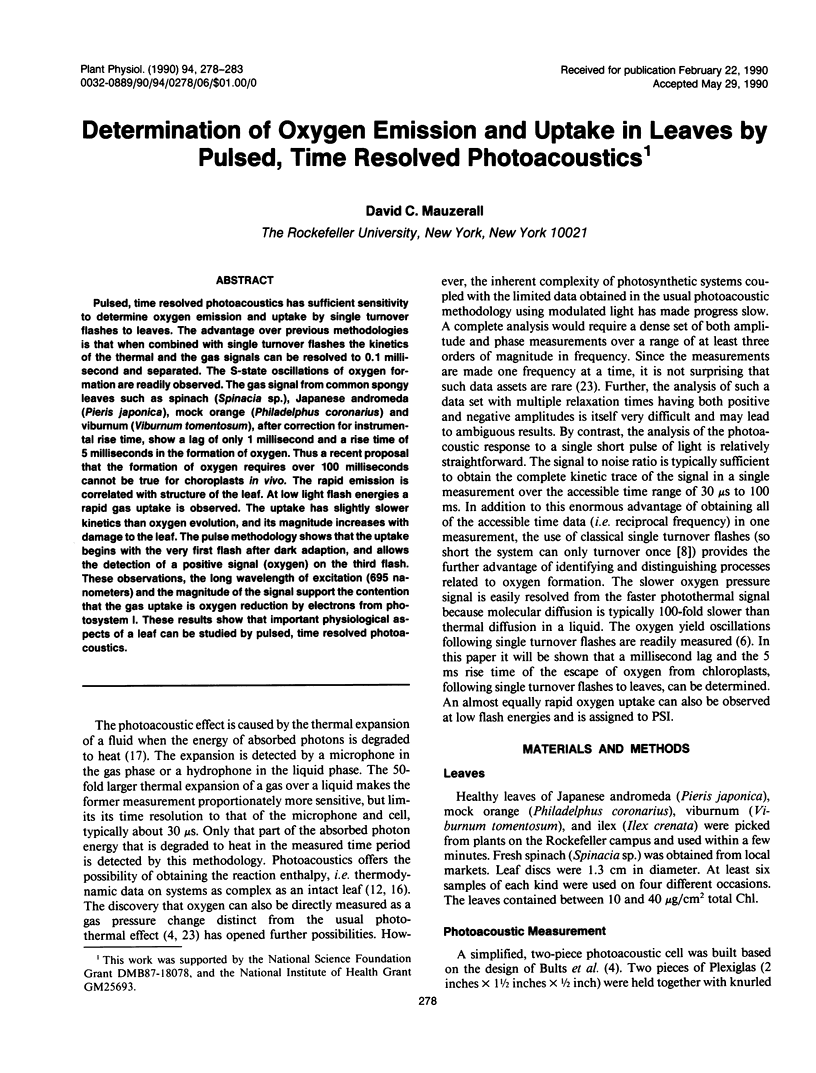
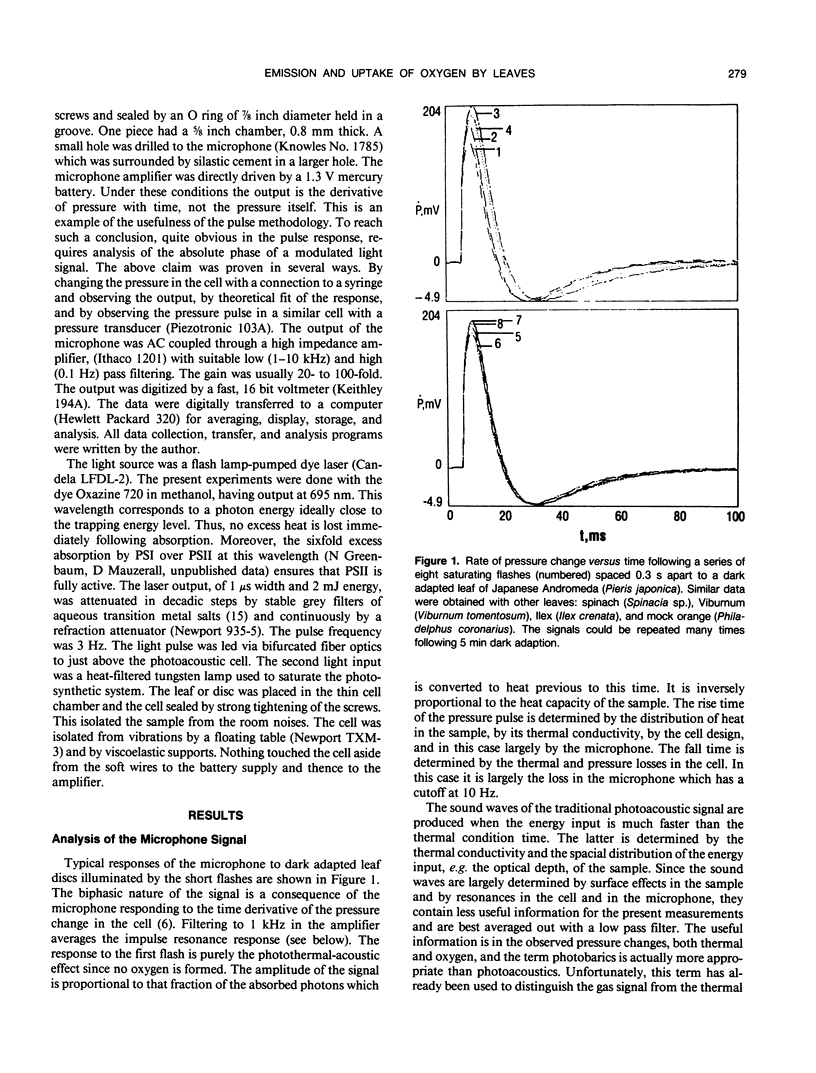
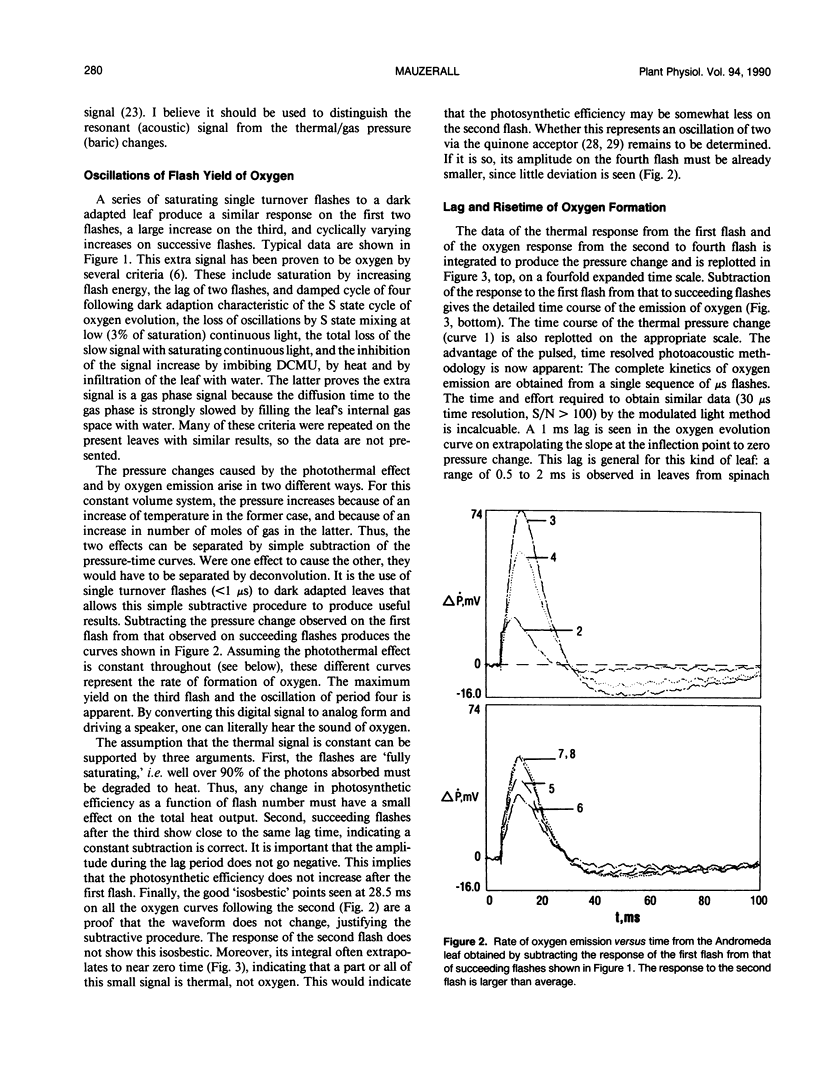
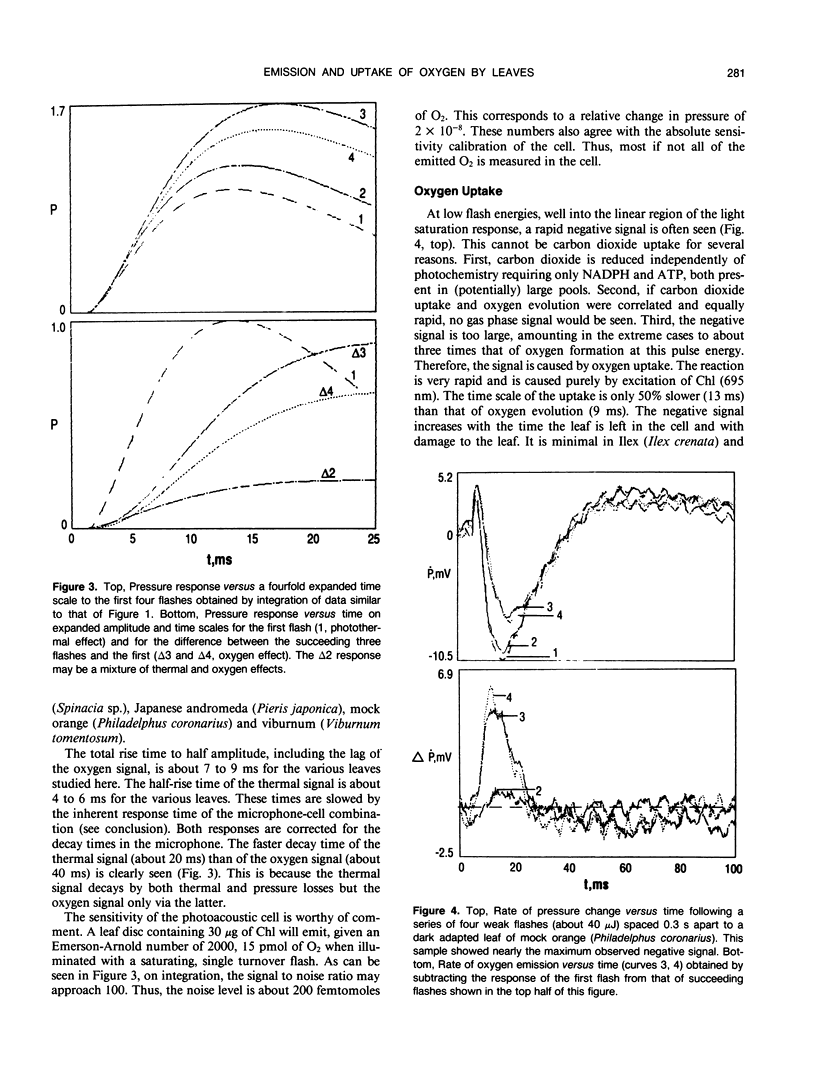
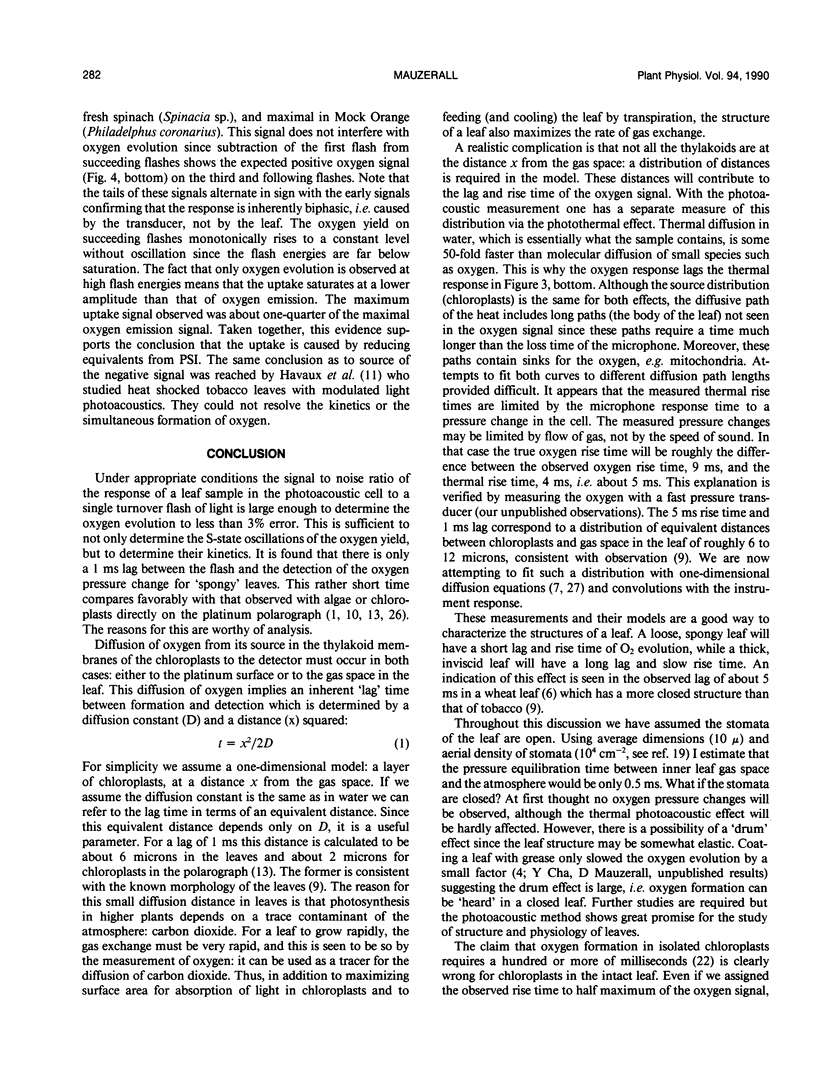
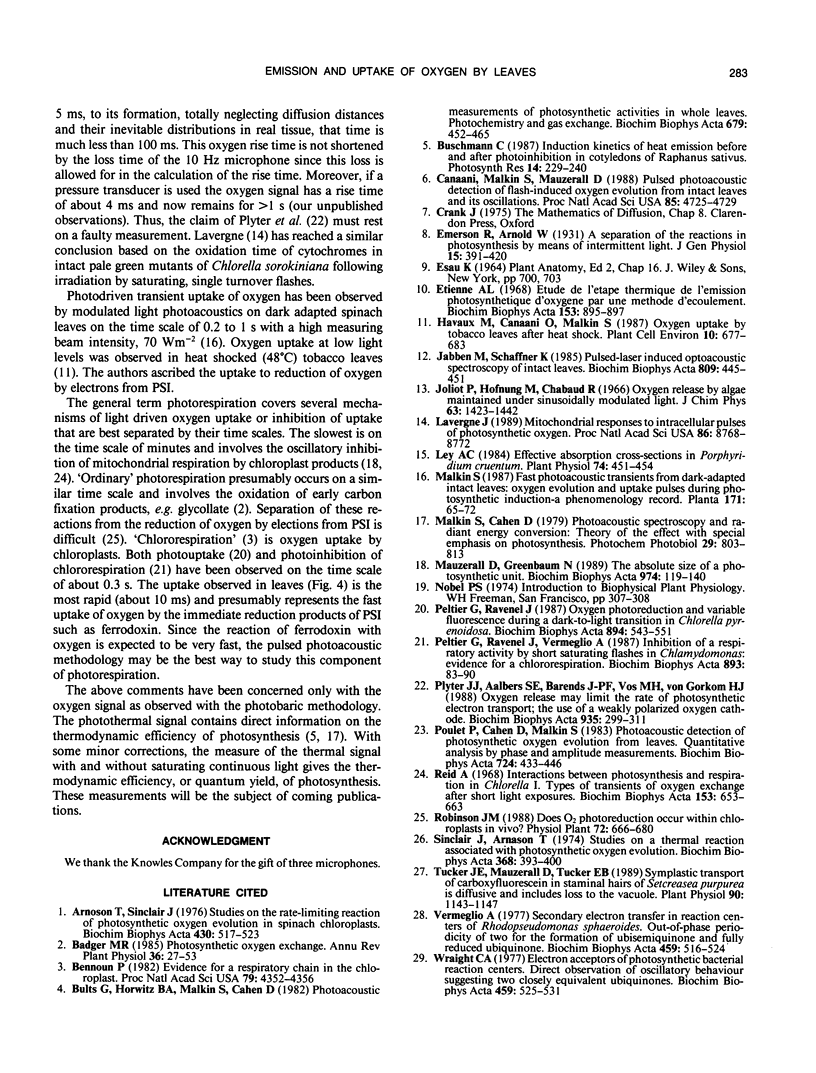
Selected References
These references are in PubMed. This may not be the complete list of references from this article.
- Arnason T., Sinclair J. Studies on the rate-limiting reaction of photosynthetic oxygen evolution in spinach chloroplasts. Biochim Biophys Acta. 1976 Jun 8;430(3):517–523. doi: 10.1016/0005-2728(76)90027-x. [DOI] [PubMed] [Google Scholar]
- Bennoun P. Evidence for a respiratory chain in the chloroplast. Proc Natl Acad Sci U S A. 1982 Jul;79(14):4352–4356. doi: 10.1073/pnas.79.14.4352. [DOI] [PMC free article] [PubMed] [Google Scholar]
- Canaani O., Malkin S., Mauzerall D. Pulsed photoacoustic detection of flash-induced oxygen evolution from intact leaves and its oscillations. Proc Natl Acad Sci U S A. 1988 Jul;85(13):4725–4729. doi: 10.1073/pnas.85.13.4725. [DOI] [PMC free article] [PubMed] [Google Scholar]
- Etienne A. Etude de l'étape thermique de l'émission photosynthétique d'oxygène par une méthode d'écoulement. Biochim Biophys Acta. 1968 May 28;153(4):895–897. doi: 10.1016/0005-2728(68)90018-2. [DOI] [PubMed] [Google Scholar]
- Lavergne J. Mitochondrial responses to intracellular pulses of photosynthetic oxygen. Proc Natl Acad Sci U S A. 1989 Nov;86(22):8768–8772. doi: 10.1073/pnas.86.22.8768. [DOI] [PMC free article] [PubMed] [Google Scholar]
- Ley A. C. Effective Absorption Cross-Sections in Porphyridium cruentum: Implications for Energy Transfer between Phycobilisomes and Photosystem II Reaction Centers. Plant Physiol. 1984 Feb;74(2):451–454. doi: 10.1104/pp.74.2.451. [DOI] [PMC free article] [PubMed] [Google Scholar]
- Ried A. Interactions between photosynthesis and respiration in chlorella. I. Types of transients of oxygen exchange after short light exposures. Biochim Biophys Acta. 1968 Apr 2;153(3):653–663. doi: 10.1016/0005-2728(68)90192-8. [DOI] [PubMed] [Google Scholar]
- Sinclair J., Arnason T. Studies on a thermal reaction associated with photosynthetic oxygen evolution. Biochim Biophys Acta. 1974 Dec 19;368(3):393–400. doi: 10.1016/0005-2728(74)90184-4. [DOI] [PubMed] [Google Scholar]
- Tucker J. E., Mauzerall D., Tucker E. B. Symplastic Transport of Carboxyfluorescein in Staminal Hairs of Setcreasea purpurea Is Diffusive and Includes Loss to the Vacuole. Plant Physiol. 1989 Jul;90(3):1143–1147. doi: 10.1104/pp.90.3.1143. [DOI] [PMC free article] [PubMed] [Google Scholar]
- Vermeglio A. Secondary electron transfer in reaction centers of Rhodopseudomonas sphaeroides. Out-of-phase periodicity of two for the formation of ubisemiquinone and fully reduced ubiquinone. Biochim Biophys Acta. 1977 Mar 11;459(3):516–524. doi: 10.1016/0005-2728(77)90050-0. [DOI] [PubMed] [Google Scholar]
- Wraight C. A. Electron acceptors of photosynthetic bacterial reaction centers. Direct observation of oscillatory behaviour suggesting two closely equivalent ubiquinones. Biochim Biophys Acta. 1977 Mar 11;459(3):525–531. doi: 10.1016/0005-2728(77)90051-2. [DOI] [PubMed] [Google Scholar]