Abstract
Fatty acid and glycerolipid biosynthesis from [14C]acetate by isolated pea root plastids is completely dependent on exogenously supplied ATP. CTP, GTP, and UTP are ineffective in supporting fatty acid biosynthesis, all resulting in <3% of the activity obtained with ATP. However, ADP alone or in combination with inorganic phosphate (Pi) or pyrophosphate (PPi) gave up to 28% of the ATP control activity, whereas AMP + PPi, PPi alone, or Pi alone were ineffective in promoting fatty acid biosynthesis. The components of the dihydroxyacetonephosphate (DHAP) shuttle (DHAP, oxaloacetate, and Pi), which promote intraplastidic ATP synthesis, restored 41% of the control ATP activity, whereas the omission of any of the shuttle components abolished this activity. When the DHAP shuttle components were supplemented with ADP, the rate of fatty acid biosynthesis was completely restored to that observed in the presence of ATP. Under the conditions of ADP + DHAP shuttle-driven fatty acid biosynthesis, exogenously supplied ATP gave only a 6% additional stimulation of activity. In general, variations in the energy source had only small effects on the proportions of radioactive fatty acids and glycerolipids synthesized. Most notably, higher amounts of radioactive oleic acid, free fatty acids, and diacylglycerol and lower amounts of phosphatidic acid were observed when ADP and/or the DHAP shuttle were substituted for ATP. The results presented here indicate that, although isolated pea root plastids readily utilize exogenously supplied ATP for fatty acid biosynthesis, these plastids can also synthesize sufficient ATP when provided with the appropriate cofactors.
Full text
PDF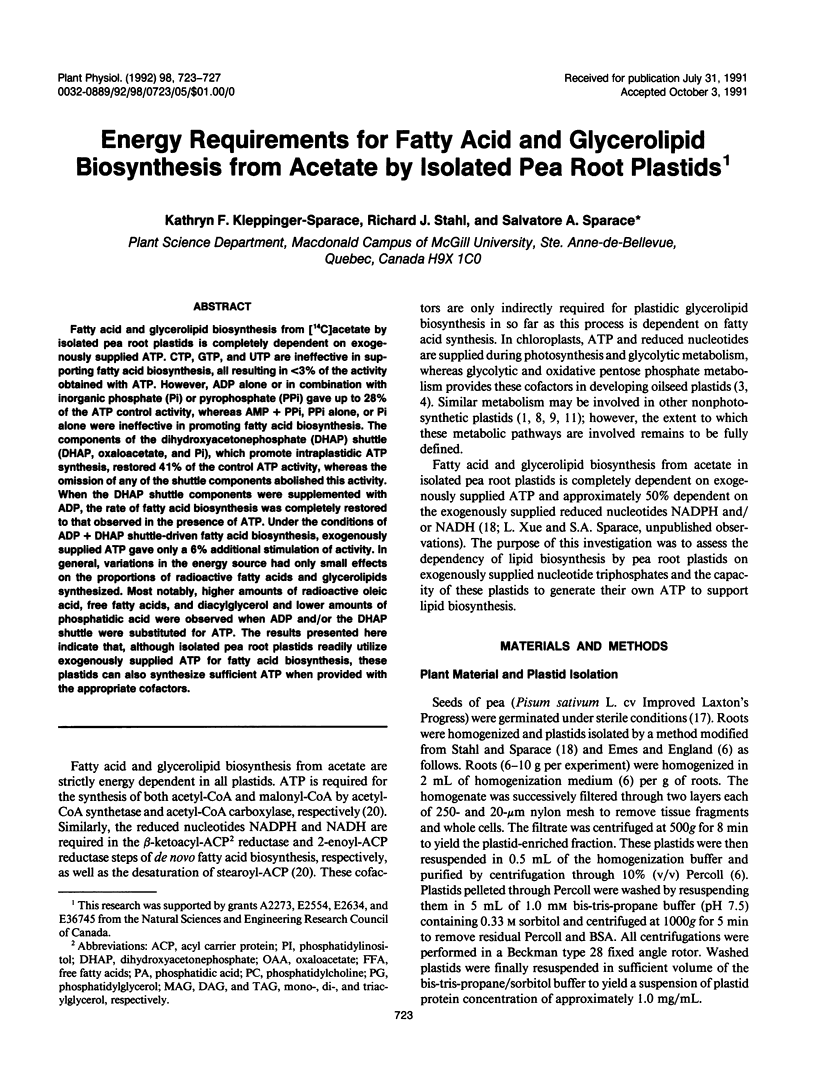
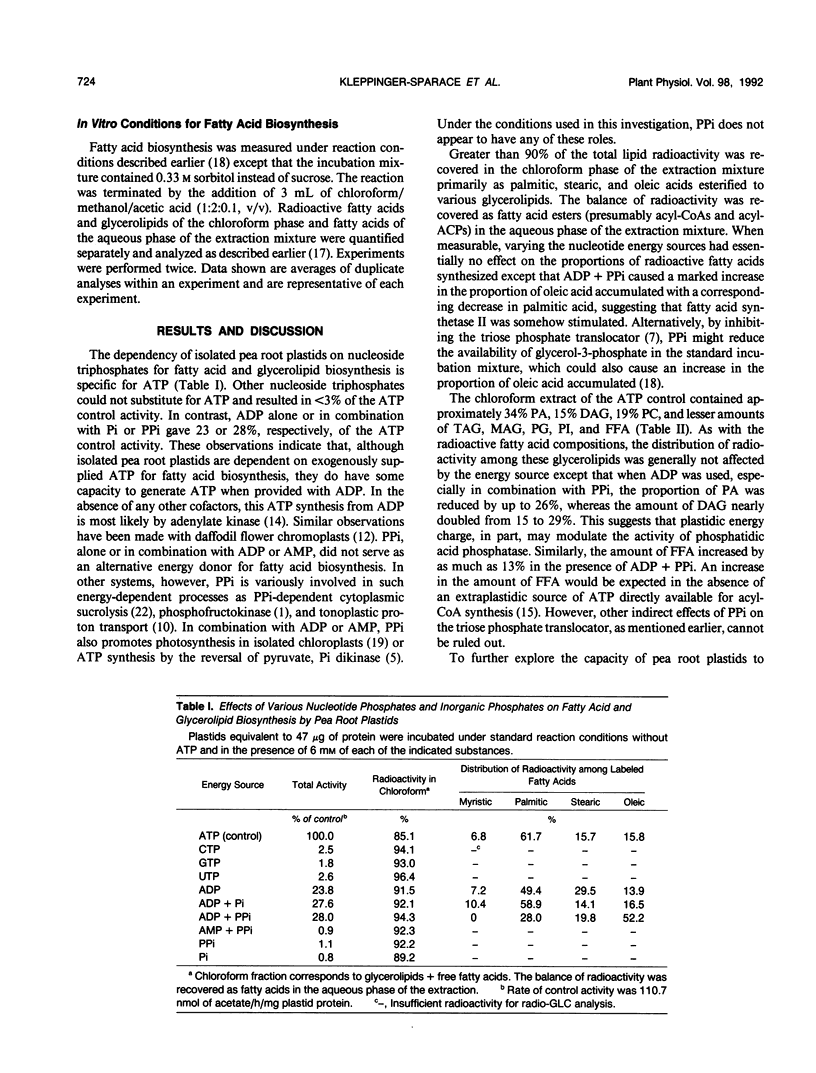
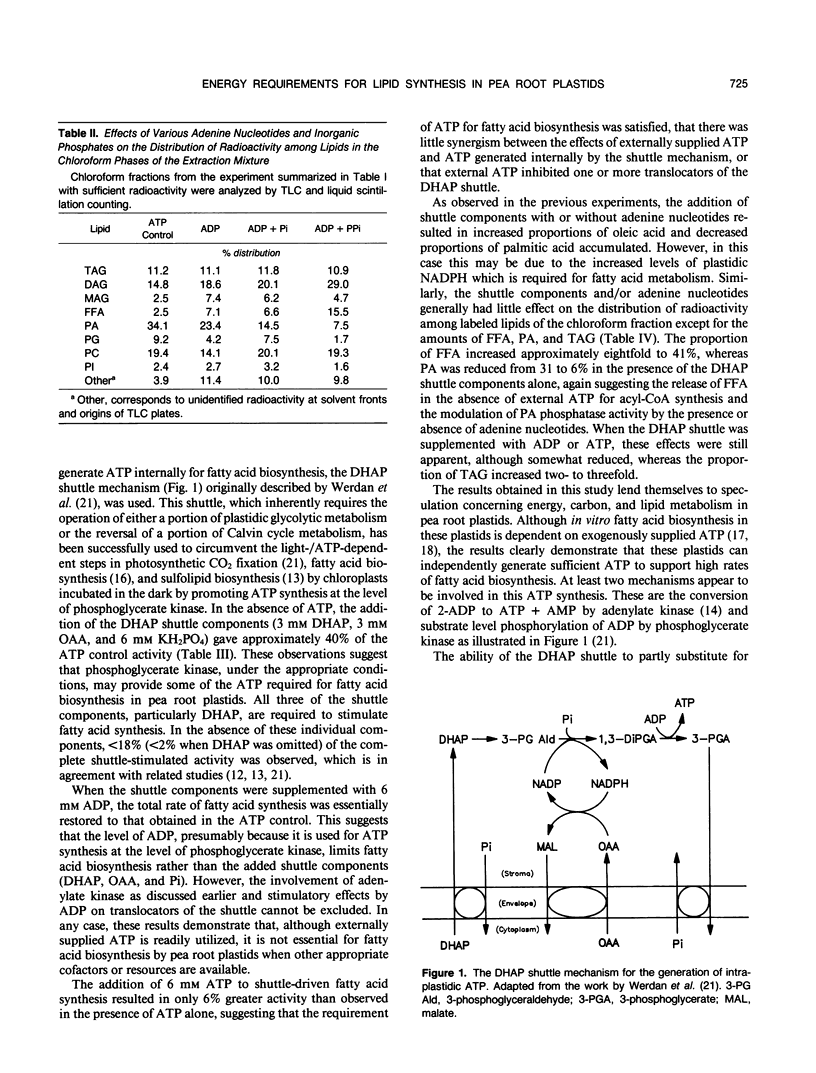
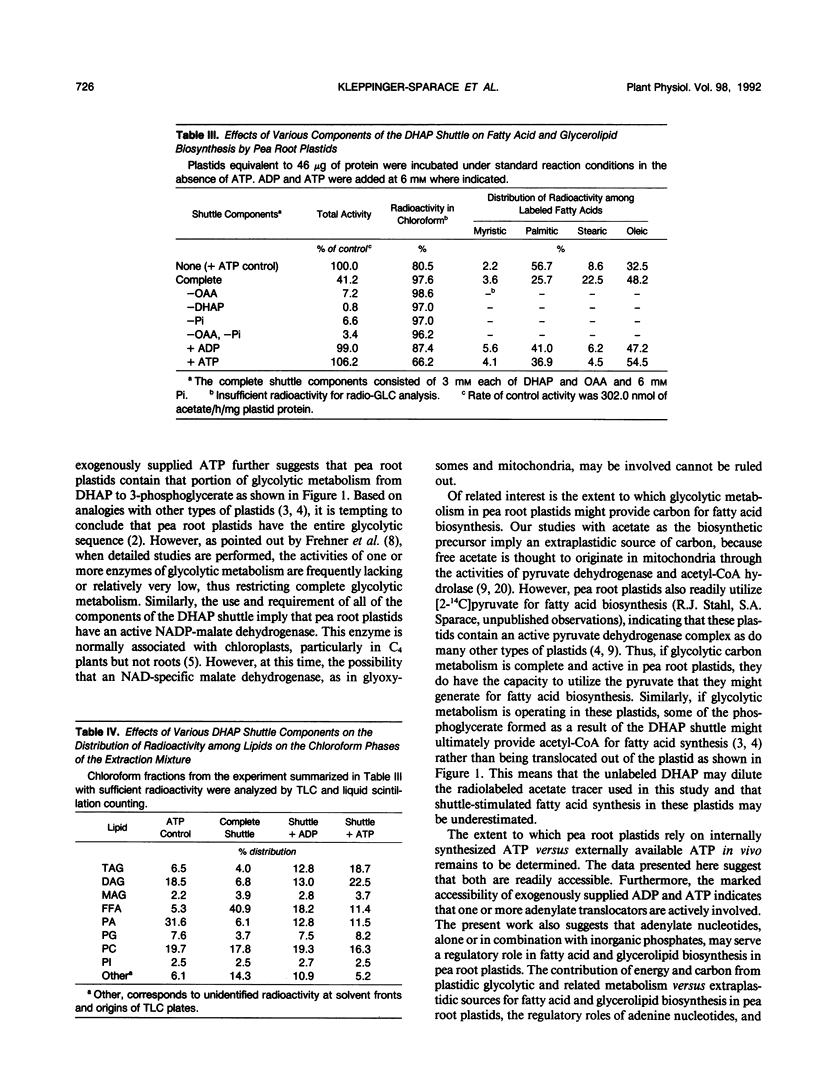
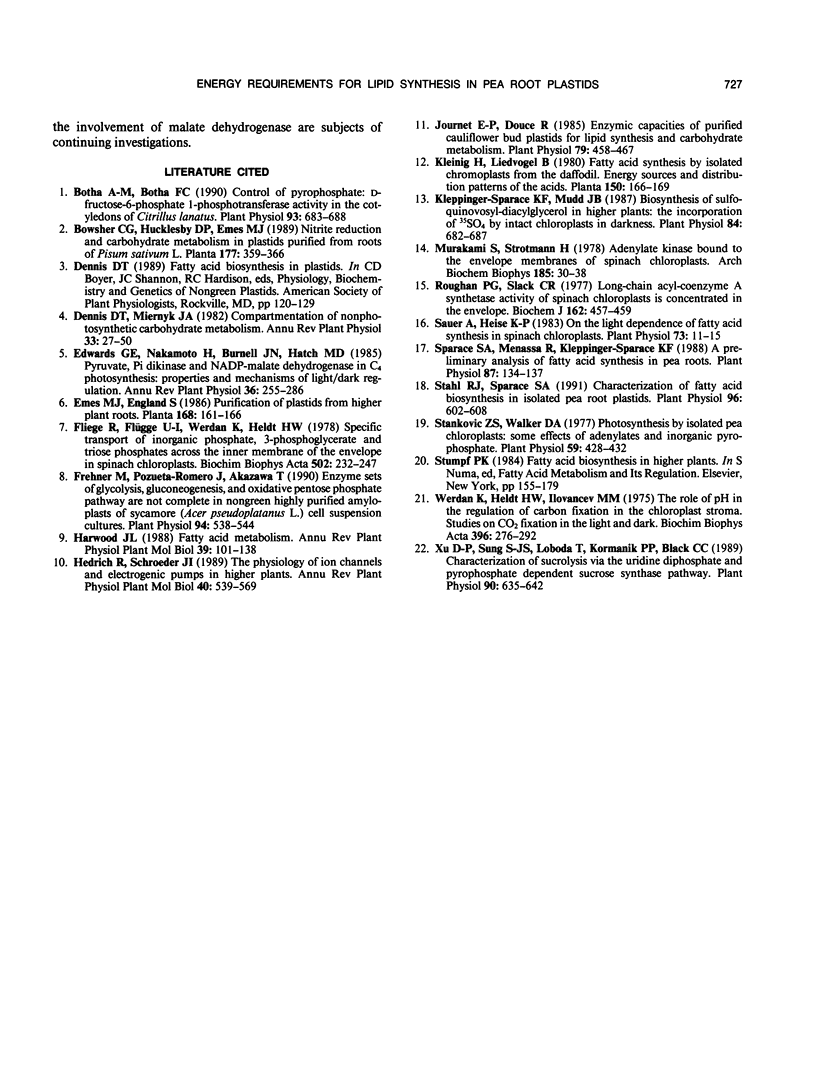
Selected References
These references are in PubMed. This may not be the complete list of references from this article.
- Botha A. M., Botha F. C. Control of Pyrophosphated-Fructose-6-Phosphate 1-Phosphotransferase Activity in the Cotyledons of Citrullus lanatus. Plant Physiol. 1990 Jun;93(2):683–688. doi: 10.1104/pp.93.2.683. [DOI] [PMC free article] [PubMed] [Google Scholar]
- Fliege R., Flügge U. I., Werdan K., Heldt H. W. Specific transport of inorganic phosphate, 3-phosphoglycerate and triosephosphates across the inner membrane of the envelope in spinach chloroplasts. Biochim Biophys Acta. 1978 May 10;502(2):232–247. doi: 10.1016/0005-2728(78)90045-2. [DOI] [PubMed] [Google Scholar]
- Frehner M., Pozueta-Romero J., Akazawa T. Enzyme Sets of Glycolysis, Gluconeogenesis, and Oxidative Pentose Phosphate Pathway Are Not Complete in Nongreen Highly Purified Amyloplasts of Sycamore (Acer pseudoplatanus L.) Cell Suspension Cultures. Plant Physiol. 1990 Oct;94(2):538–544. doi: 10.1104/pp.94.2.538. [DOI] [PMC free article] [PubMed] [Google Scholar]
- Journet E. P., Douce R. Enzymic capacities of purified cauliflower bud plastids for lipid synthesis and carbohydrate metabolism. Plant Physiol. 1985 Oct;79(2):458–467. doi: 10.1104/pp.79.2.458. [DOI] [PMC free article] [PubMed] [Google Scholar]
- Kleppinger-Sparace K. F., Mudd J. B. Biosynthesis of Sulfoquinovosyldiacylglycerol in Higher Plants: The Incorporation of SO(4) by Intact Chloroplasts in Darkness. Plant Physiol. 1987 Jul;84(3):682–687. doi: 10.1104/pp.84.3.682. [DOI] [PMC free article] [PubMed] [Google Scholar]
- Murakami S., Strotmann H. Adenylate kinase bound to the envelope membranes of spinach chloroplasts. Arch Biochem Biophys. 1978 Jan 15;185(1):30–38. doi: 10.1016/0003-9861(78)90140-6. [DOI] [PubMed] [Google Scholar]
- Roughan P. G., Slack C. R. Long-chain acyl-coenzyme A synthetase activity of spinach chloroplasts is concentrated in the envelope. Biochem J. 1977 Feb 15;162(2):457–459. doi: 10.1042/bj1620457. [DOI] [PMC free article] [PubMed] [Google Scholar]
- Sauer A., Heise K. P. On the light dependence of Fatty Acid synthesis in spinach chloroplasts. Plant Physiol. 1983 Sep;73(1):11–15. doi: 10.1104/pp.73.1.11. [DOI] [PMC free article] [PubMed] [Google Scholar]
- Sparace S. A., Menassa R., Kleppinger-Sparace K. F. A preliminary analysis of Fatty Acid synthesis in pea roots. Plant Physiol. 1988 May;87(1):134–137. doi: 10.1104/pp.87.1.134. [DOI] [PMC free article] [PubMed] [Google Scholar]
- Stahl R. J., Sparace S. A. Characterization of Fatty Acid biosynthesis in isolated pea root plastids. Plant Physiol. 1991 Jun;96(2):602–608. doi: 10.1104/pp.96.2.602. [DOI] [PMC free article] [PubMed] [Google Scholar]
- Stankovic Z. S., Walker D. A. Photosynthesis by isolated pea chloroplasts: some effects of adenylates and inorganic pyrophosphate. Plant Physiol. 1977 Mar;59(3):428–432. doi: 10.1104/pp.59.3.428. [DOI] [PMC free article] [PubMed] [Google Scholar]
- Werdan K., Heldt H. W., Milovancev M. The role of pH in the regulation of carbon fixation in the chloroplast stroma. Studies on CO2 fixation in the light and dark. Biochim Biophys Acta. 1975 Aug 11;396(2):276–292. doi: 10.1016/0005-2728(75)90041-9. [DOI] [PubMed] [Google Scholar]
- Xu D. P., Sung S. J., Loboda T., Kormanik P. P., Black C. C. Characterization of Sucrolysis via the Uridine Diphosphate and Pyrophosphate-Dependent Sucrose Synthase Pathway. Plant Physiol. 1989 Jun;90(2):635–642. doi: 10.1104/pp.90.2.635. [DOI] [PMC free article] [PubMed] [Google Scholar]