Abstract
Roots of intact 5-day-old maize (Zea mays L.) seedlings were exposed to 3 micromolar Cd during a 7-day period. Cysteine, γ-glutamylcysteine, glutathione (GSH), and Cd-induced acid-soluble thiols (ASTs), including phytochelatins, were quantified in roots and shoots. Adaptation to Cd and its cost to seedling development were evaluated by measuring Cd content, tissue fresh weight, and rate of root elongation. Roots contained 60 to 67% of the Cd in the seedlings between 4 and 7 days of exposure. Exposure to Cd decreased the fresh weight gain in roots from day 4 onward without affecting the shoots. Between days 1.5 and 3.5 of Cd treatment, roots elongated more slowly than controls; however, their growth rate recovered thereafter and exceeded that of controls. Exposure to Cd did not appreciably affect the concentration of cysteine in the seedlings. However, the initial low concentration of γ-glutamylcysteine increased (after a lag of 6 hours in roots and 2 days in shoots), reaching a plateau by day 6 at 28.5 nanomoles per gram of fresh weight in roots and by day 5 at 19.1 nanomoles per gram of fresh weight in shoots. During the first 9 hours of Cd exposure, the concentration of GSH in roots decreased dramatically (at 31.6 nanomoles per gram of fresh weight per hour) and thereafter decreased more slowly than in controls. The depletion of GSH in the roots (366 nanomoles per gram of fresh weight) matched the synthesis of ASTs (349 nanomoles per gram of fresh weight) during the first 48 hours. The concentration of ASTs in roots increased steadily thereafter to reach 662.2 nanomoles per gram of fresh weight by 6 days of Cd exposure. In shoots, Cd had little influence on the concentration of GSH, but ASTs still accumulated to 173.3 nanomoles per gram fresh weight after 5 days. The molar ratio of thiols in ASTs to Cd increased to a maximum of 10.24 in roots after 4 hours and of 4.25 in shoots after 2 days of Cd exposure. After 4 days, the ratio reached a plateau of approximately 2 in roots and between 2 and 3 in shoots, as if a steady state of Cd chelation had been achieved in both organs. The plateau coincided with recovered root elongation or an adaptation to Cd. The reduced fresh weight gain of the roots during this time, however, indicated that the synthesis of Cd-induced thiols was at a cost to root development.
Full text
PDF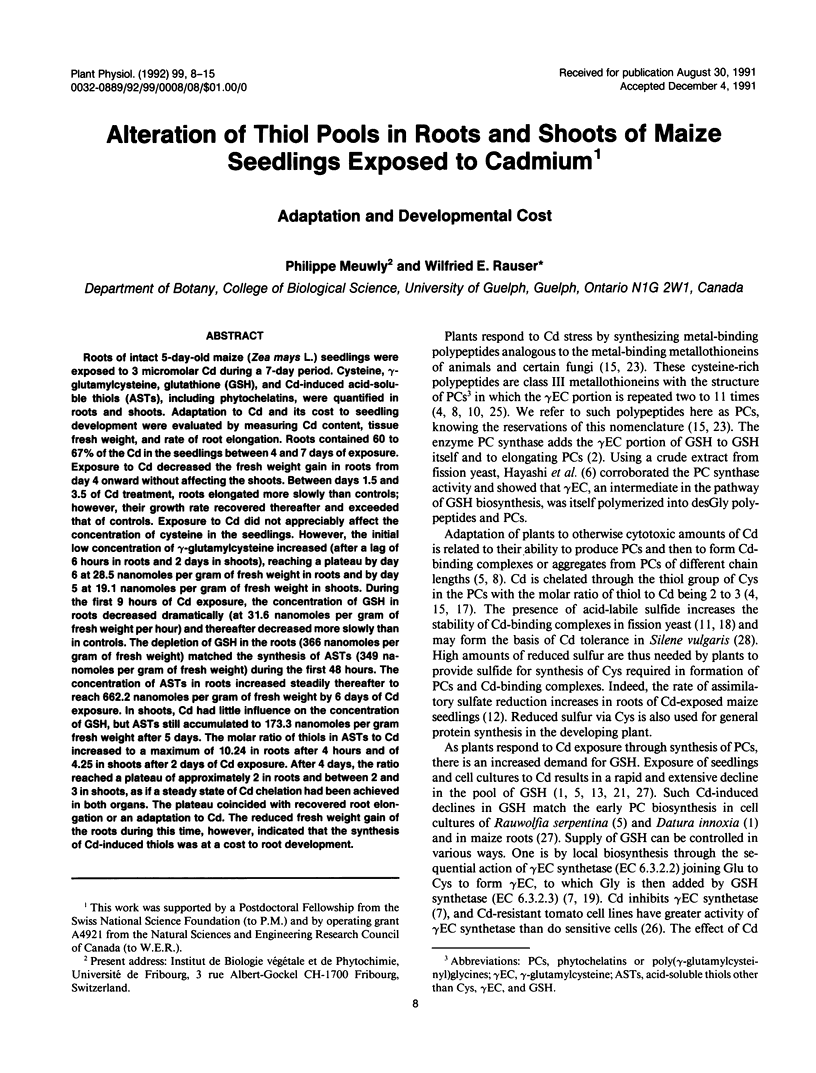
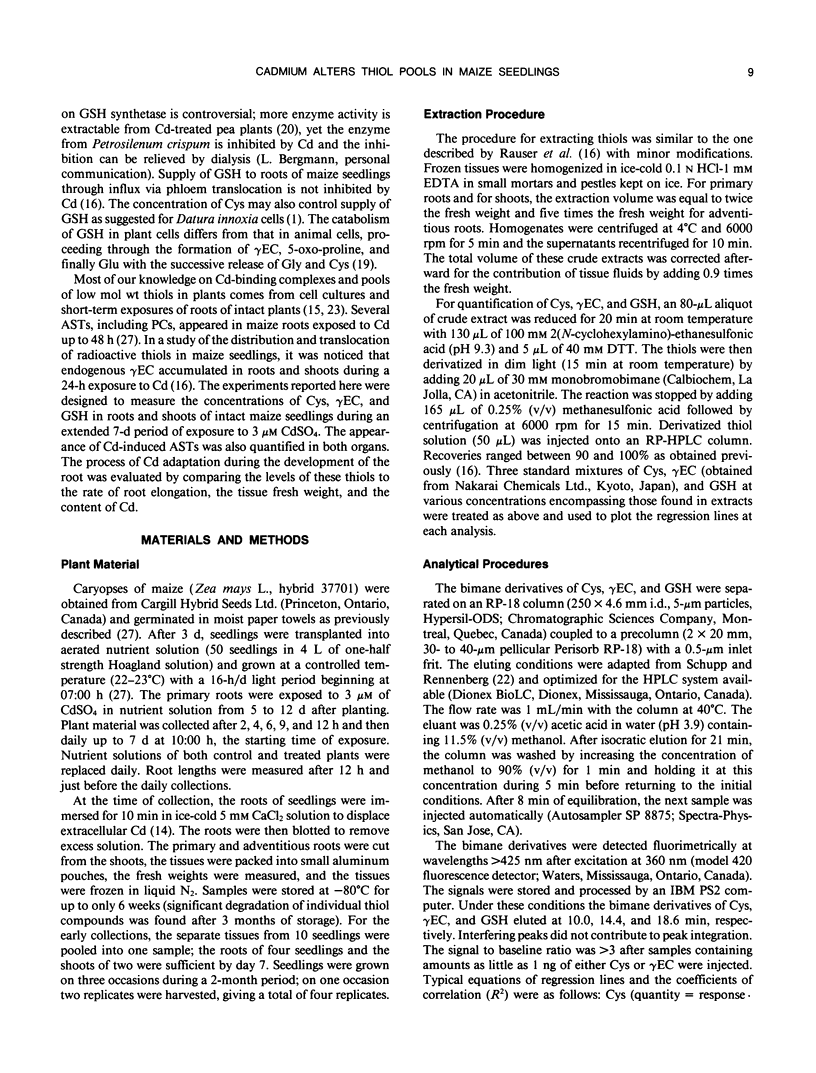
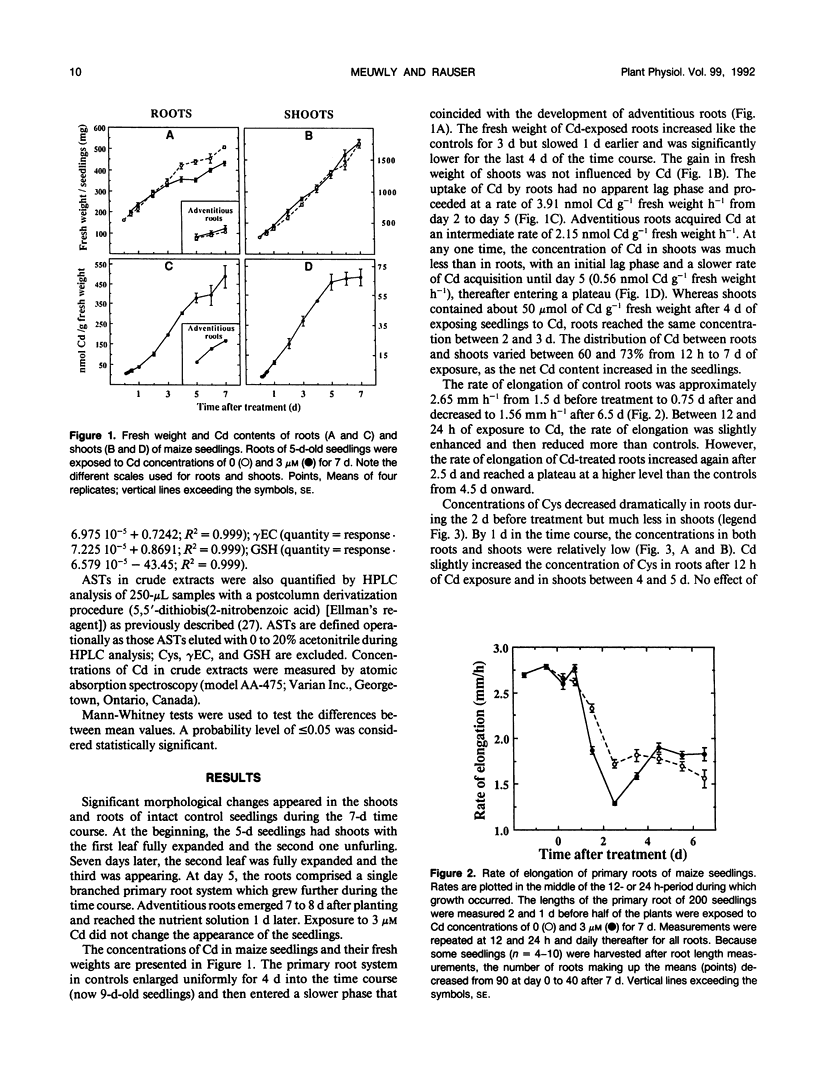
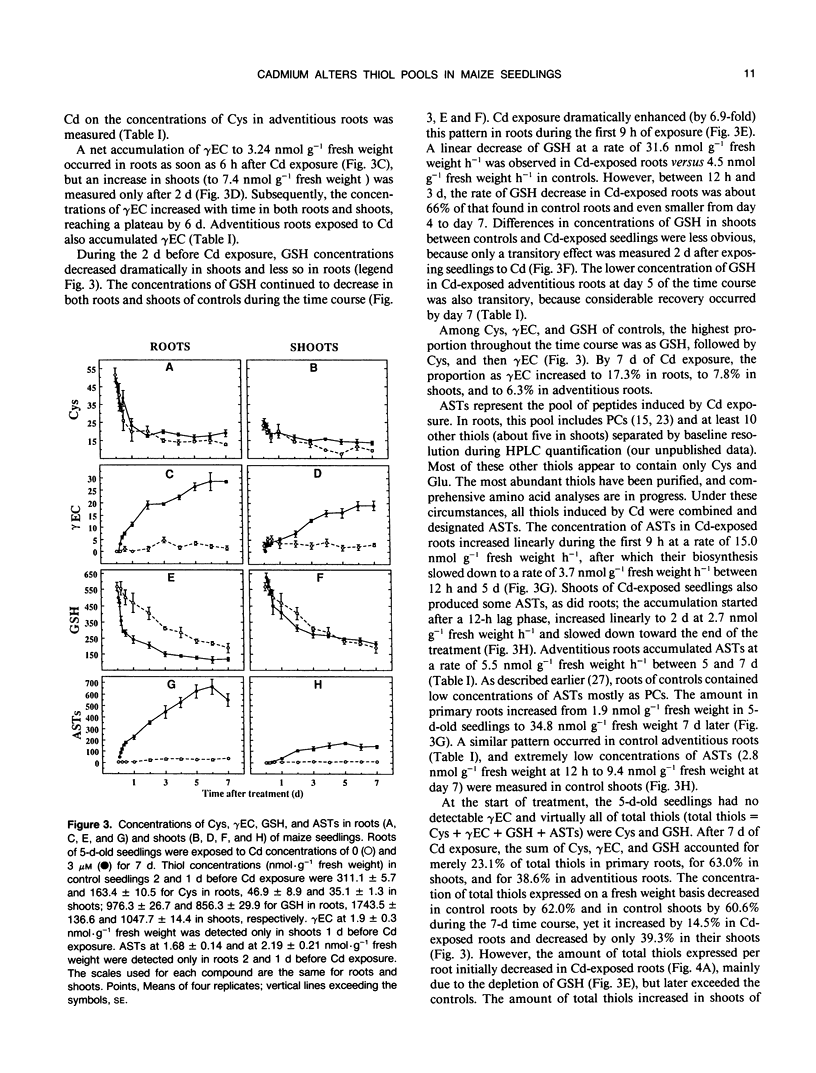
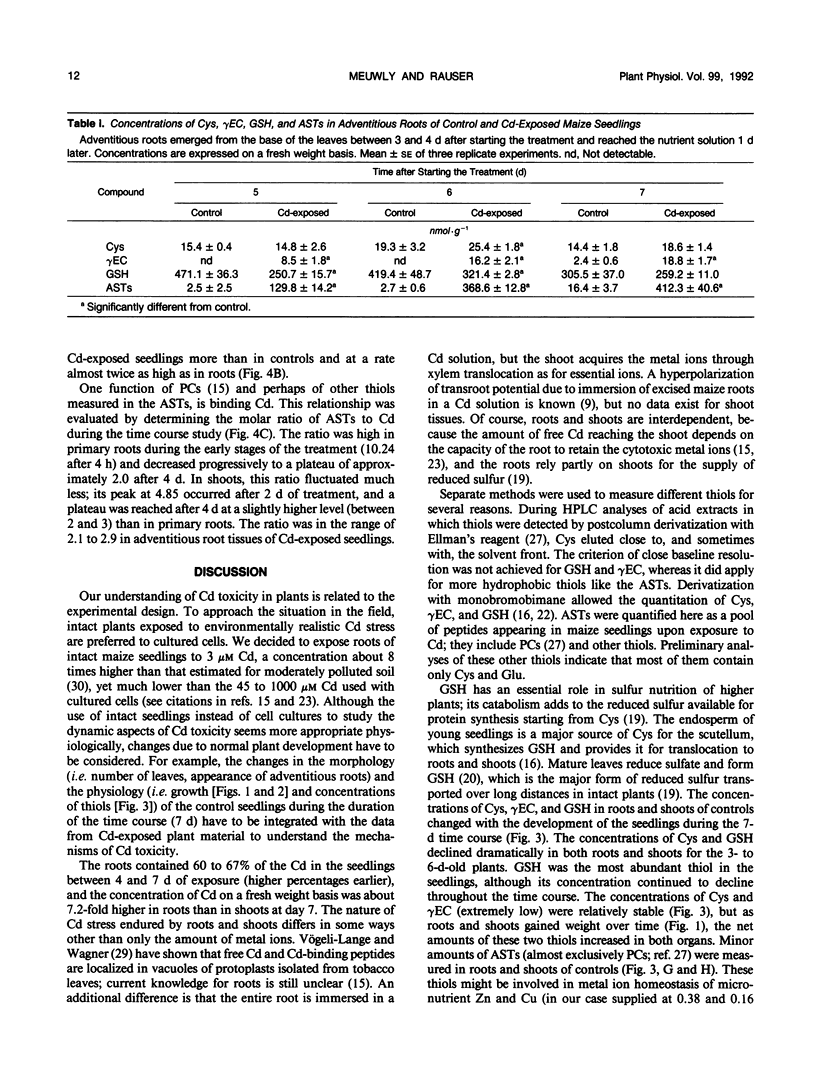
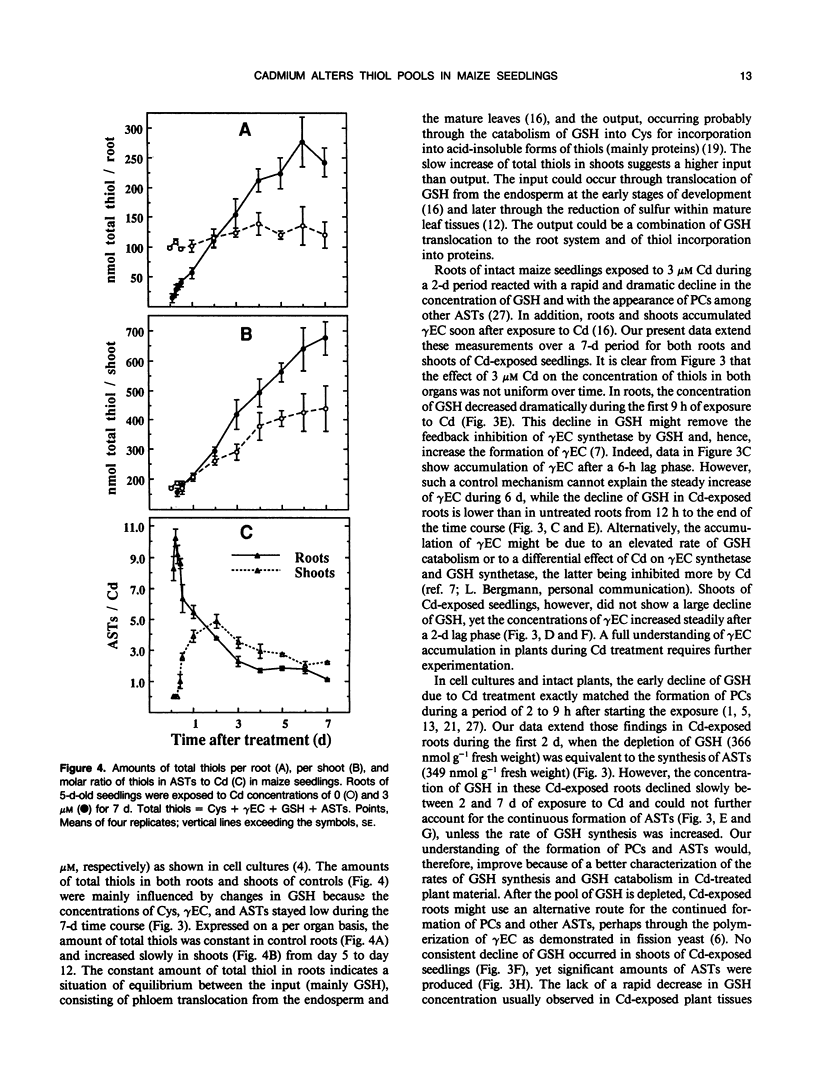
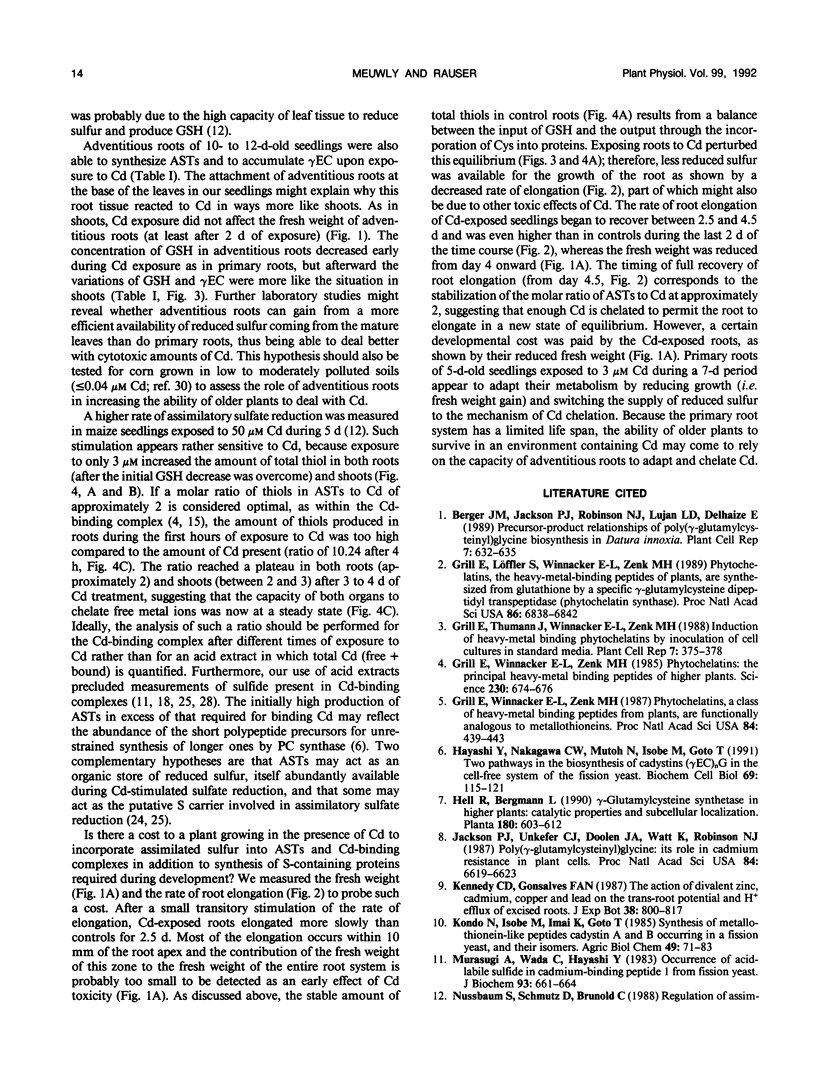
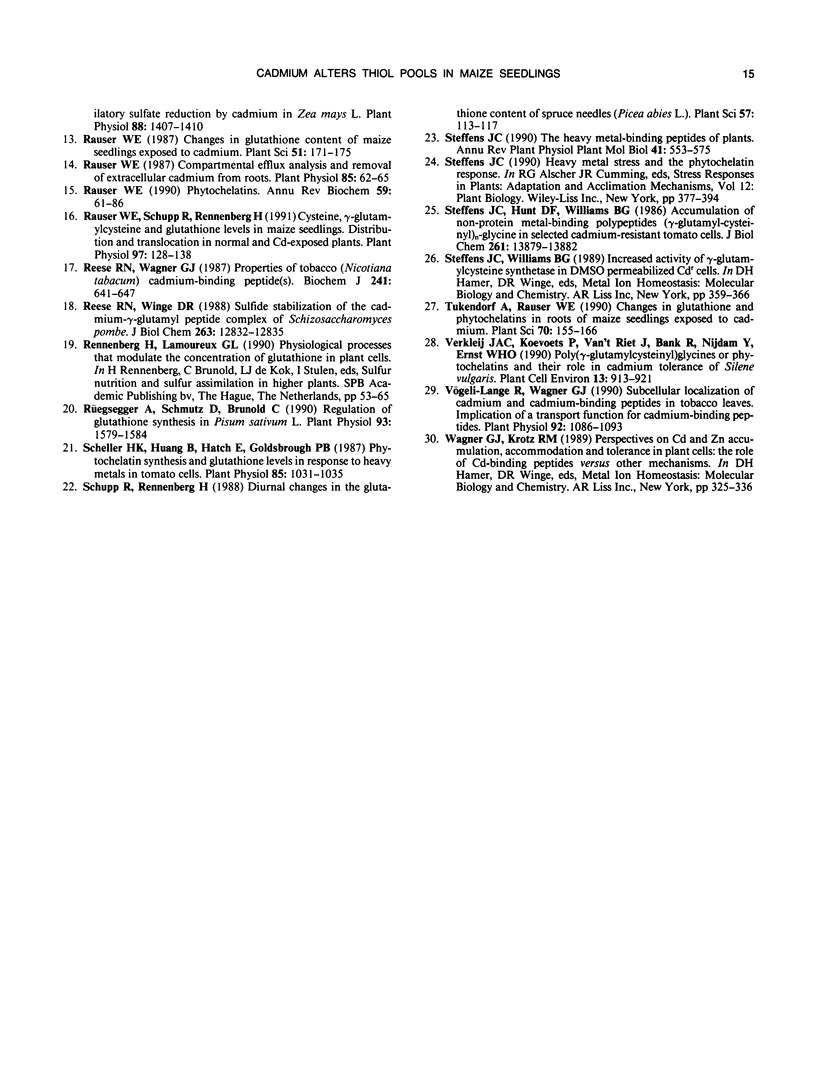
Selected References
These references are in PubMed. This may not be the complete list of references from this article.
- Grill E., Löffler S., Winnacker E. L., Zenk M. H. Phytochelatins, the heavy-metal-binding peptides of plants, are synthesized from glutathione by a specific gamma-glutamylcysteine dipeptidyl transpeptidase (phytochelatin synthase). Proc Natl Acad Sci U S A. 1989 Sep;86(18):6838–6842. doi: 10.1073/pnas.86.18.6838. [DOI] [PMC free article] [PubMed] [Google Scholar]
- Grill E., Winnacker E. L., Zenk M. H. Phytochelatins, a class of heavy-metal-binding peptides from plants, are functionally analogous to metallothioneins. Proc Natl Acad Sci U S A. 1987 Jan;84(2):439–443. doi: 10.1073/pnas.84.2.439. [DOI] [PMC free article] [PubMed] [Google Scholar]
- Grill E., Winnacker E. L., Zenk M. H. Phytochelatins: the principal heavy-metal complexing peptides of higher plants. Science. 1985 Nov 8;230(4726):674–676. doi: 10.1126/science.230.4726.674. [DOI] [PubMed] [Google Scholar]
- Hayashi Y., Nakagawa C. W., Mutoh N., Isobe M., Goto T. Two pathways in the biosynthesis of cadystins (gamma EC)nG in the cell-free system of the fission yeast. Biochem Cell Biol. 1991 Feb-Mar;69(2-3):115–121. doi: 10.1139/o91-018. [DOI] [PubMed] [Google Scholar]
- Jackson P. J., Unkefer C. J., Doolen J. A., Watt K., Robinson N. J. Poly(gamma-glutamylcysteinyl)glycine: its role in cadmium resistance in plant cells. Proc Natl Acad Sci U S A. 1987 Oct;84(19):6619–6623. doi: 10.1073/pnas.84.19.6619. [DOI] [PMC free article] [PubMed] [Google Scholar]
- Murasugi A., Wada C., Hayashi Y. Occurrence of acid-labile sulfide in cadmium-binding peptide 1 from fission yeast. J Biochem. 1983 Feb;93(2):661–664. doi: 10.1093/oxfordjournals.jbchem.a134222. [DOI] [PubMed] [Google Scholar]
- Nussbaum S., Schmutz D., Brunold C. Regulation of Assimilatory Sulfate Reduction by Cadmium in Zea mays L. Plant Physiol. 1988 Dec;88(4):1407–1410. doi: 10.1104/pp.88.4.1407. [DOI] [PMC free article] [PubMed] [Google Scholar]
- Rauser W. E. Compartmental efflux analysis and removal of extracellular cadmium from roots. Plant Physiol. 1987 Sep;85(1):62–65. doi: 10.1104/pp.85.1.62. [DOI] [PMC free article] [PubMed] [Google Scholar]
- Rauser W. E. Phytochelatins. Annu Rev Biochem. 1990;59:61–86. doi: 10.1146/annurev.bi.59.070190.000425. [DOI] [PubMed] [Google Scholar]
- Rauser W. E., Schupp R., Rennenberg H. Cysteine, gamma-Glutamylcysteine, and Glutathione Levels in Maize Seedlings : Distribution and Translocation in Normal and Cadmium-Exposed Plants. Plant Physiol. 1991 Sep;97(1):128–138. doi: 10.1104/pp.97.1.128. [DOI] [PMC free article] [PubMed] [Google Scholar]
- Reese R. N., Wagner G. J. Properties of tobacco (Nicotiana tabacum) cadmium-binding peptide(s). Unique non-metallothionein cadmium ligands. Biochem J. 1987 Feb 1;241(3):641–647. doi: 10.1042/bj2410641. [DOI] [PMC free article] [PubMed] [Google Scholar]
- Reese R. N., Winge D. R. Sulfide stabilization of the cadmium-gamma-glutamyl peptide complex of Schizosaccharomyces pombe. J Biol Chem. 1988 Sep 15;263(26):12832–12835. [PubMed] [Google Scholar]
- Rüegsegger A., Schmutz D., Brunold C. Regulation of Glutathione Synthesis by Cadmium in Pisum sativum L. Plant Physiol. 1990 Aug;93(4):1579–1584. doi: 10.1104/pp.93.4.1579. [DOI] [PMC free article] [PubMed] [Google Scholar]
- Scheller H. V., Huang B., Hatch E., Goldsbrough P. B. Phytochelatin synthesis and glutathione levels in response to heavy metals in tomato cells. Plant Physiol. 1987 Dec;85(4):1031–1035. doi: 10.1104/pp.85.4.1031. [DOI] [PMC free article] [PubMed] [Google Scholar]
- Steffens J. C., Hunt D. F., Williams B. G. Accumulation of non-protein metal-binding polypeptides (gamma-glutamyl-cysteinyl)n-glycine in selected cadmium-resistant tomato cells. J Biol Chem. 1986 Oct 25;261(30):13879–13882. [PubMed] [Google Scholar]
- Vögeli-Lange R., Wagner G. J. Subcellular localization of cadmium and cadmium-binding peptides in tobacco leaves : implication of a transport function for cadmium-binding peptides. Plant Physiol. 1990 Apr;92(4):1086–1093. doi: 10.1104/pp.92.4.1086. [DOI] [PMC free article] [PubMed] [Google Scholar]