Abstract
Tobacco (Nicotiana tabacum var Samsun) was transformed using the bacterial gor gene coding for the enzyme glutathione reductase. Transgenic plants were selected by their kanamycin resistence and expression of the bacterial gor gene. After separation by isoelectric focusing techniques, leaf extracts from transgenic plants having both native and bacterial glutathione reductase activity gave, in addition to the six bands of the native enzyme, two further closely running isoenzymes. These additional bands originating from the expression of the bacterial gor gene were nonchloroplastic. Leaves from transgenic plants had two- to 10-fold higher glutathione reductase activity than non-transgenic controls. The amount of extractable glutathione reductase activity obtained in transgenic plants was dependent on leaf age and the conditions to which leaves were exposed. Both light and exposure to methylviologen increased leaf glutathione reductase activity. Elevated levels of cytosolic glutathione reductase activity in transgenic plants had no effect on the amount or reduction state of the reduced glutathione/oxidized glutathione pool under optimal conditions or oxidative conditions induced by methylviologen. The glutathione pool was unaltered despite the oxidation-dependent loss of CO2 assimilation and oxidation of enzymes involved in photosynthesis. However, the reduction state of the ascorbate pool was greater in transgenic plants relative to nontransgenic controls following illumination of methylviologen-treated leaf discs. Therefore, we conclude that in the natural state glutathione reductase is present in tobacco at levels above those required for maximal operation of the ascorbate-glutathione pathway.
Full text
PDF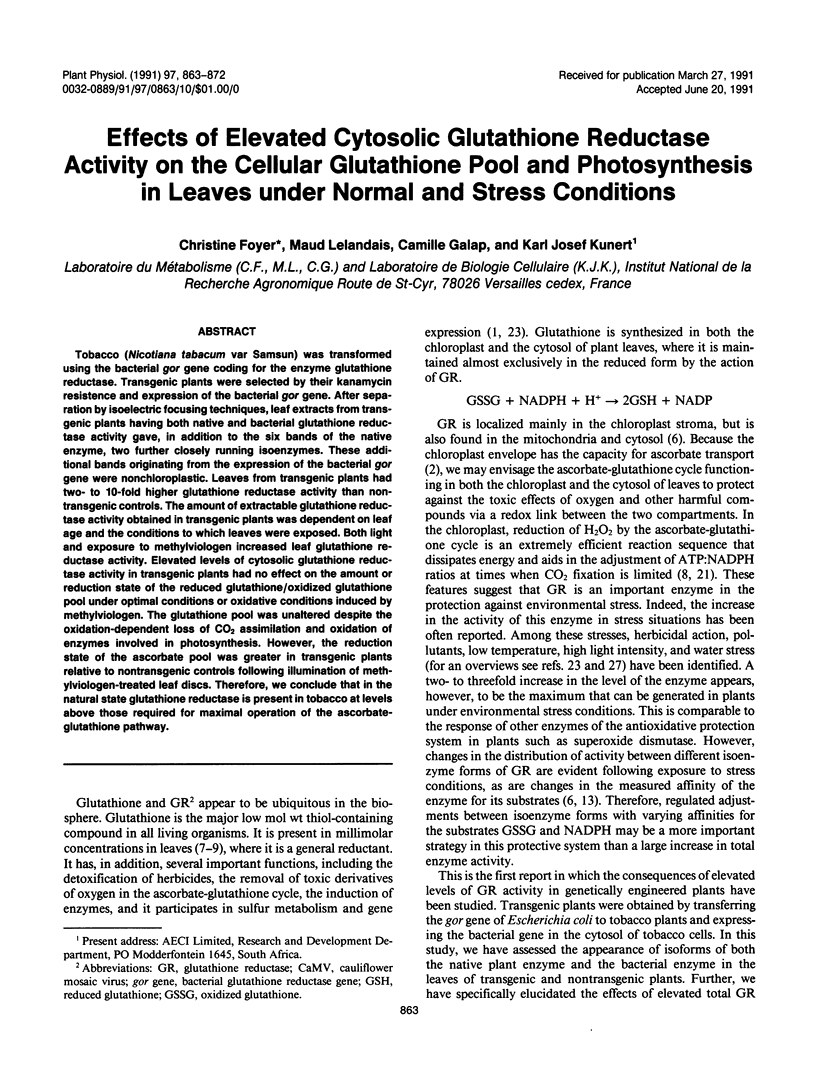
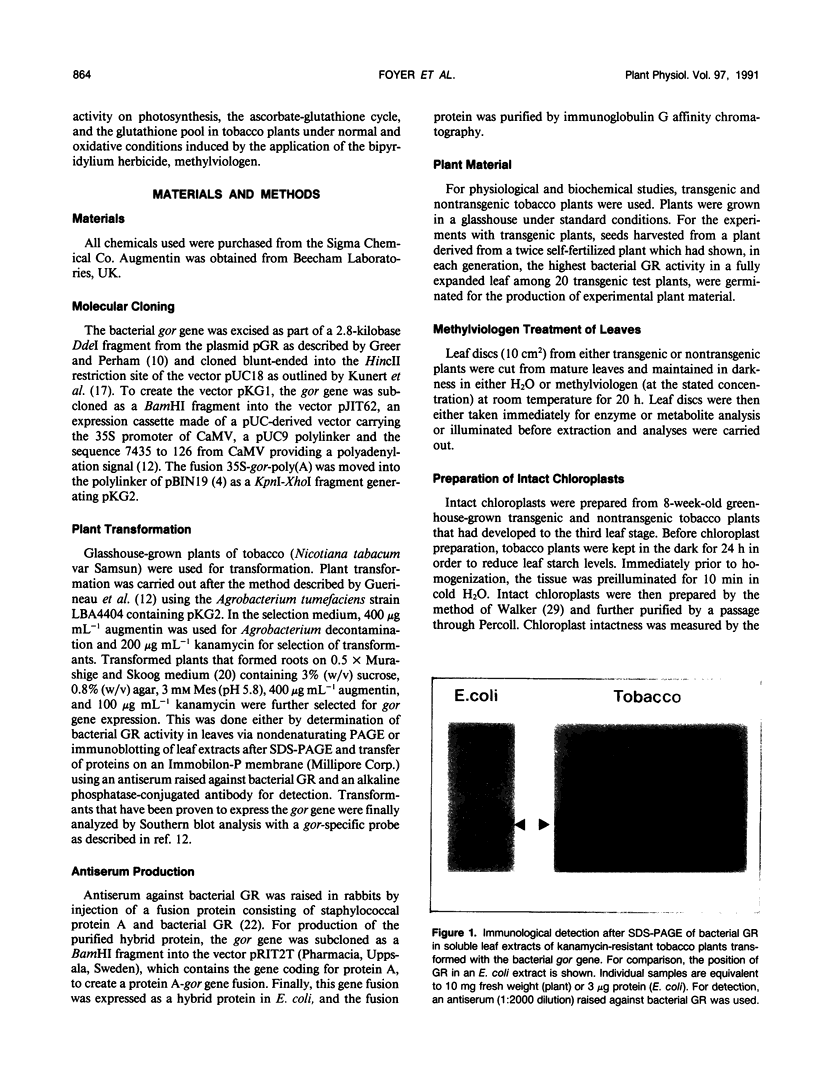
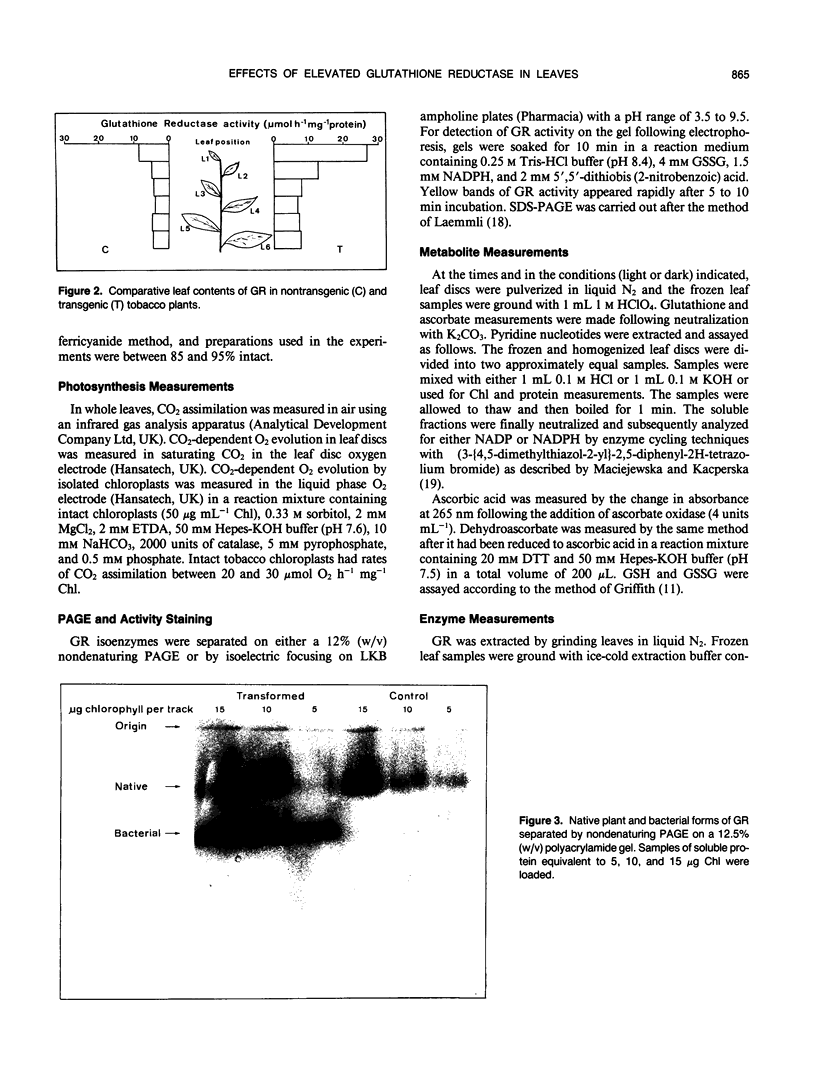
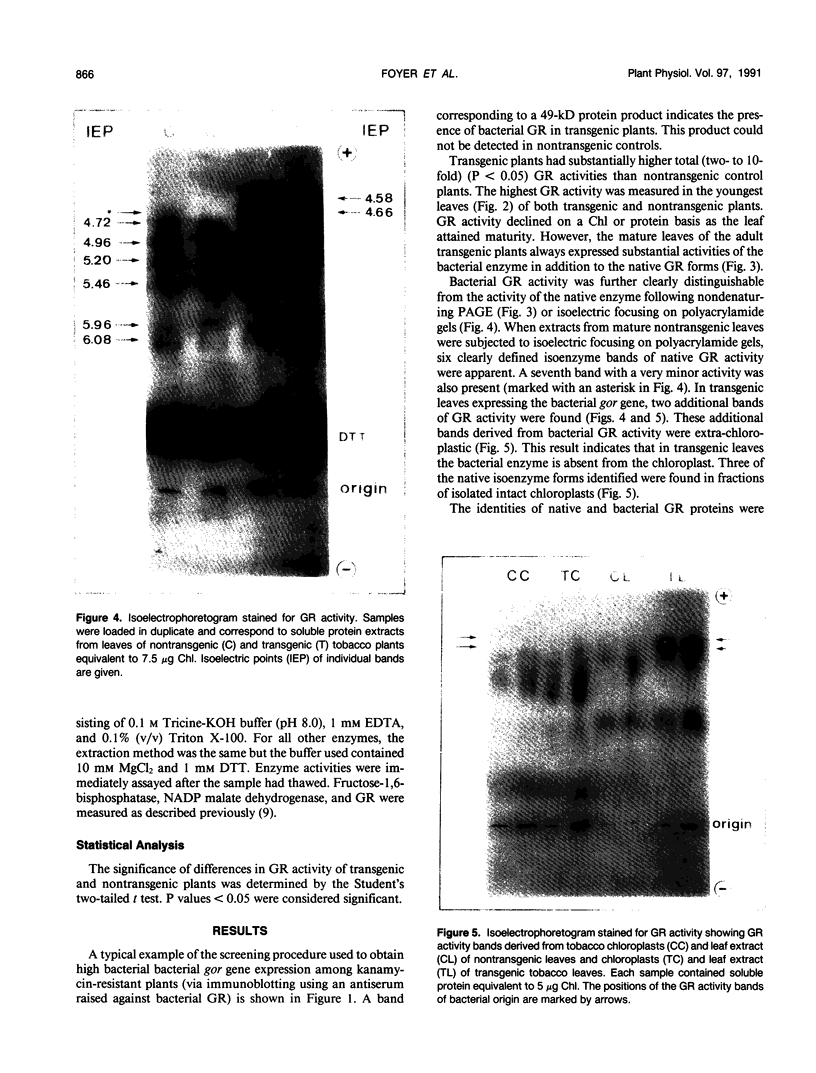
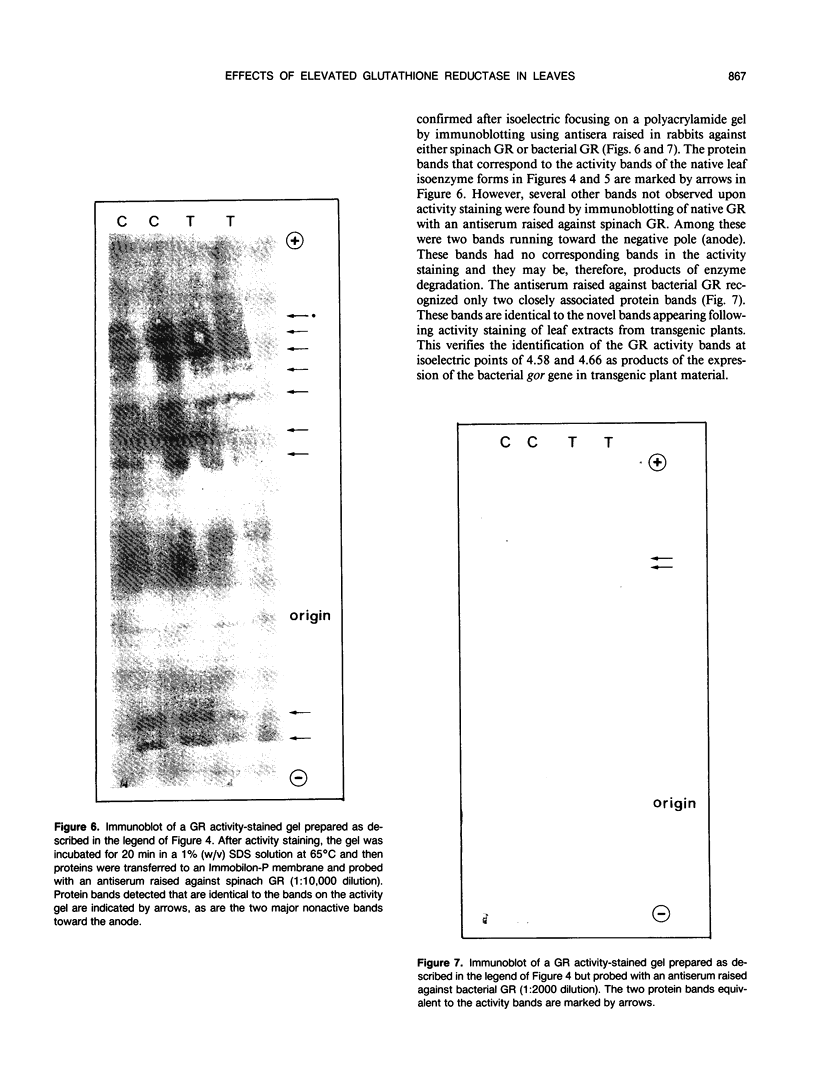
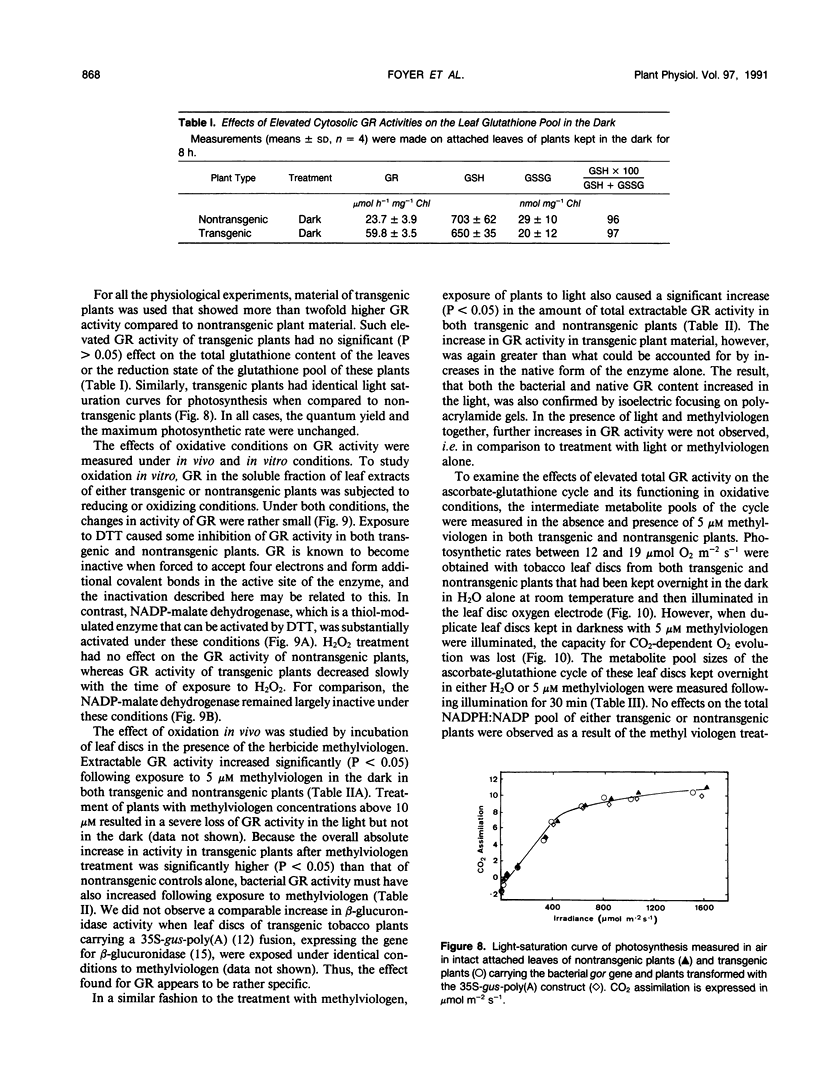
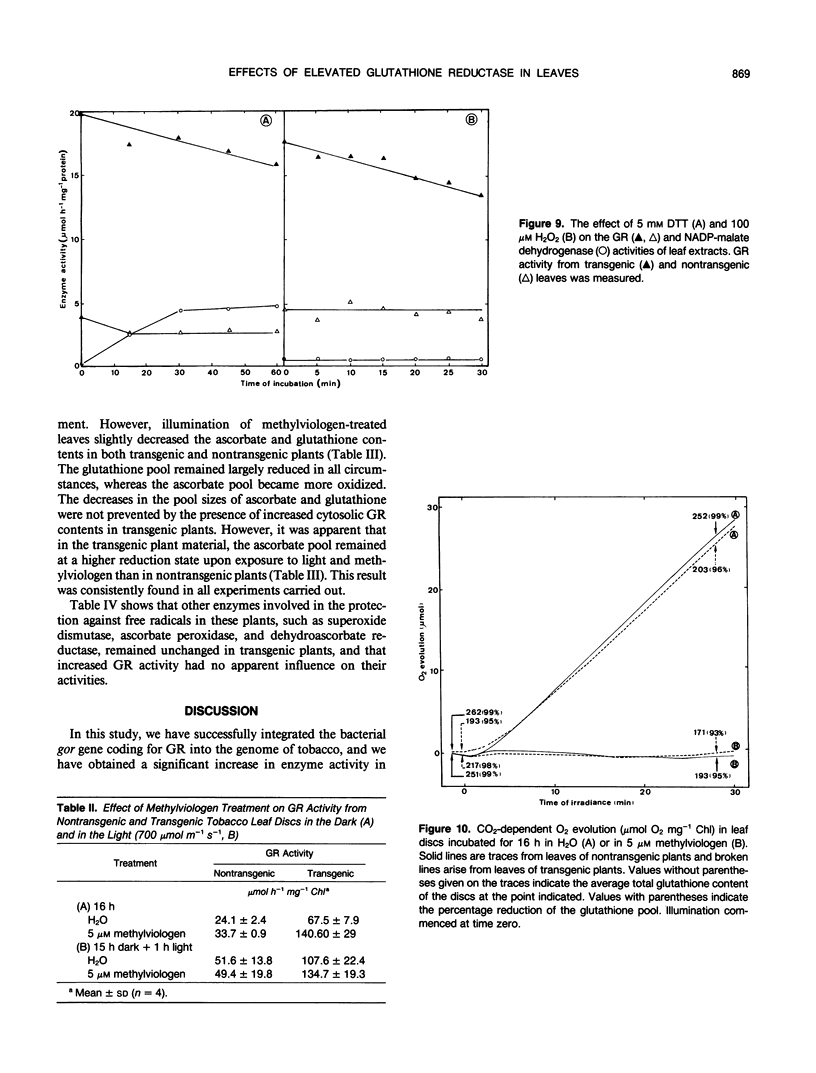
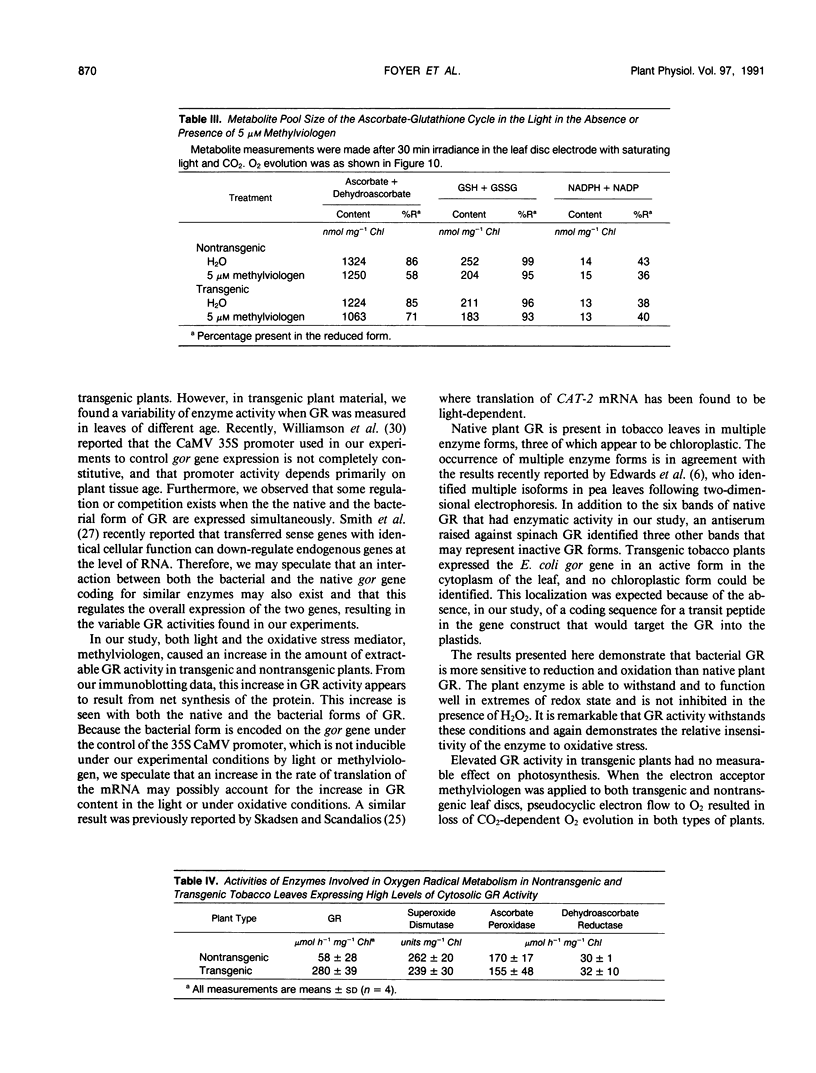
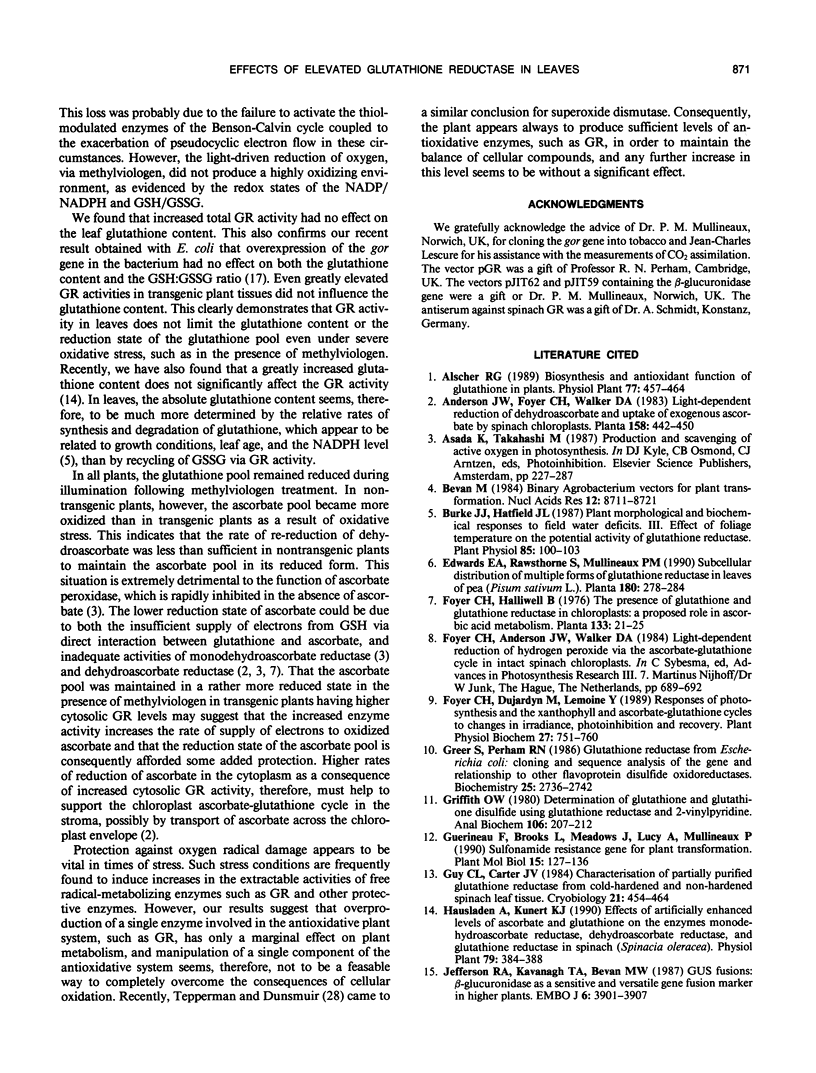
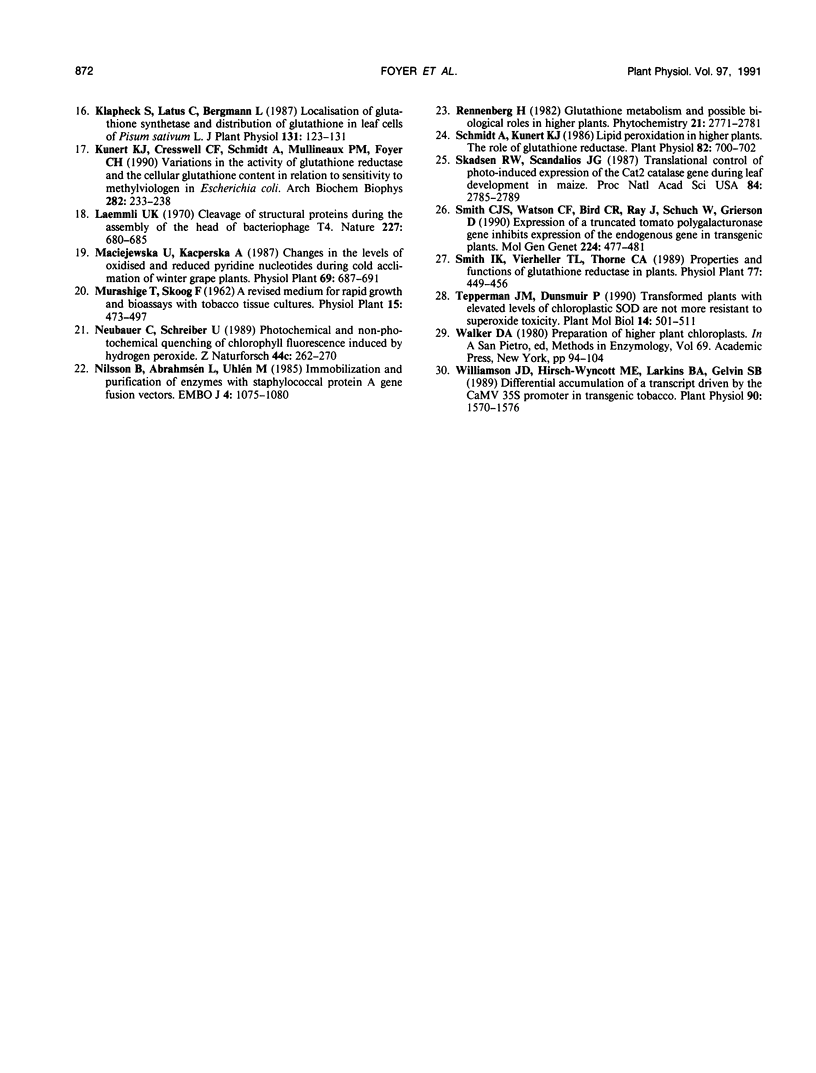
Images in this article
Selected References
These references are in PubMed. This may not be the complete list of references from this article.
- Bevan M. Binary Agrobacterium vectors for plant transformation. Nucleic Acids Res. 1984 Nov 26;12(22):8711–8721. doi: 10.1093/nar/12.22.8711. [DOI] [PMC free article] [PubMed] [Google Scholar]
- Burke J. J., Hatfield J. L. Plant Morphological and Biochemical Responses to Field Water Deficits: III. Effect of Foliage Temperature on the Potential Activity of Glutathione Reductase. Plant Physiol. 1987 Sep;85(1):100–103. doi: 10.1104/pp.85.1.100. [DOI] [PMC free article] [PubMed] [Google Scholar]
- Greer S., Perham R. N. Glutathione reductase from Escherichia coli: cloning and sequence analysis of the gene and relationship to other flavoprotein disulfide oxidoreductases. Biochemistry. 1986 May 6;25(9):2736–2742. doi: 10.1021/bi00357a069. [DOI] [PubMed] [Google Scholar]
- Griffith O. W. Determination of glutathione and glutathione disulfide using glutathione reductase and 2-vinylpyridine. Anal Biochem. 1980 Jul 15;106(1):207–212. doi: 10.1016/0003-2697(80)90139-6. [DOI] [PubMed] [Google Scholar]
- Guerineau F., Brooks L., Meadows J., Lucy A., Robinson C., Mullineaux P. Sulfonamide resistance gene for plant transformation. Plant Mol Biol. 1990 Jul;15(1):127–136. doi: 10.1007/BF00017730. [DOI] [PubMed] [Google Scholar]
- Jefferson R. A., Kavanagh T. A., Bevan M. W. GUS fusions: beta-glucuronidase as a sensitive and versatile gene fusion marker in higher plants. EMBO J. 1987 Dec 20;6(13):3901–3907. doi: 10.1002/j.1460-2075.1987.tb02730.x. [DOI] [PMC free article] [PubMed] [Google Scholar]
- Kunert K. J., Cresswell C. F., Schmidt A., Mullineaux P. M., Foyer C. H. Variations in the activity of glutathione reductase and the cellular glutathione content in relation to sensitivity to methylviologen in Escherichia coli. Arch Biochem Biophys. 1990 Nov 1;282(2):233–238. doi: 10.1016/0003-9861(90)90110-k. [DOI] [PubMed] [Google Scholar]
- Laemmli U. K. Cleavage of structural proteins during the assembly of the head of bacteriophage T4. Nature. 1970 Aug 15;227(5259):680–685. doi: 10.1038/227680a0. [DOI] [PubMed] [Google Scholar]
- Nilsson B., Abrahmsén L., Uhlén M. Immobilization and purification of enzymes with staphylococcal protein A gene fusion vectors. EMBO J. 1985 Apr;4(4):1075–1080. doi: 10.1002/j.1460-2075.1985.tb03741.x. [DOI] [PMC free article] [PubMed] [Google Scholar]
- Schmidt A., Kunert K. J. Lipid peroxidation in higher plants : the role of glutathione reductase. Plant Physiol. 1986 Nov;82(3):700–702. doi: 10.1104/pp.82.3.700. [DOI] [PMC free article] [PubMed] [Google Scholar]
- Skadsen R. W., Scandalios J. G. Translational control of photo-induced expression of the Cat2 catalase gene during leaf development in maize. Proc Natl Acad Sci U S A. 1987 May;84(9):2785–2789. doi: 10.1073/pnas.84.9.2785. [DOI] [PMC free article] [PubMed] [Google Scholar]
- Smith C. J., Watson C. F., Bird C. R., Ray J., Schuch W., Grierson D. Expression of a truncated tomato polygalacturonase gene inhibits expression of the endogenous gene in transgenic plants. Mol Gen Genet. 1990 Dec;224(3):477–481. doi: 10.1007/BF00262443. [DOI] [PubMed] [Google Scholar]
- Tepperman J. M., Dunsmuir P. Transformed plants with elevated levels of chloroplastic SOD are not more resistant to superoxide toxicity. Plant Mol Biol. 1990 Apr;14(4):501–511. doi: 10.1007/BF00027496. [DOI] [PubMed] [Google Scholar]
- Williamson J. D., Hirsch-Wyncott M. E., Larkins B. A., Gelvin S. B. Differential Accumulation of a Transcript Driven by the CaMV 35S Promoter in Transgenic Tobacco. Plant Physiol. 1989 Aug;90(4):1570–1576. doi: 10.1104/pp.90.4.1570. [DOI] [PMC free article] [PubMed] [Google Scholar]