Abstract
Mushroom tyrosinase, which is known to convert a variety of o-diphenols into o-benzoquinones, has been shown to catalyse an unusual oxidative decarboxylation of 3,4-dihydroxymandelic acid to 3,4-dihydroxybenzaldehyde [Sugumaran (1986) Biochemistry 25, 4489-4492]. The mechanism of this reaction was re-investigated. Although visible-region spectral studies of the reaction mixture containing 3,4-dihydroxymandelic acid and tyrosinase failed to generate the spectrum of a quinone product during the steady state of the reaction, both trapping experiments and non-steady-state kinetic experiments provided evidence for the transient formation of unstable 3,4-mandeloquinone in the reaction mixture. The visible-region spectrum of mandeloquinone resembled related quinones and exhibited an absorbance maximum at 394 nm. Since attempts to trap the second intermediate, namely alpha,2-dihydroxy-p-quinone methide, were in vain, mechanistic studies were undertaken to provide evidence for its participation. The decarboxylative quinone methide formation from 3,4-mandeloquinone dictates the retention of a proton on the alpha-carbon atom. Hence, if we replace this proton with deuterium, the resultant 3,4-dihydroxybenzaldehyde should retain the deuterium present in the original substrate. To test this hypothesis, we chemoenzymically synthesized alpha-deuterated 3,4-dihydroxymandelic acid and examined its enzymic oxidation. Our studies reveal that the resultant 3,4-dihydroxybenzaldehyde retained nearly 90% of the deuterium, strongly indicating the transient formation of quinone methide. On the basis of these findings it is concluded that the enzymic oxidation of 3,4-dihydroxymandelic acid generates the conventional quinone product, which, owing to its unstability, is rapidly decarboxylated to generate transient alpha,2-dihydroxy-p-quinone methide. The coupled dienone-phenol re-arrangement and keto-enol tautomerism of this quinone methide produce the observed 3,4-dihydroxybenzaldehyde.
Full text
PDF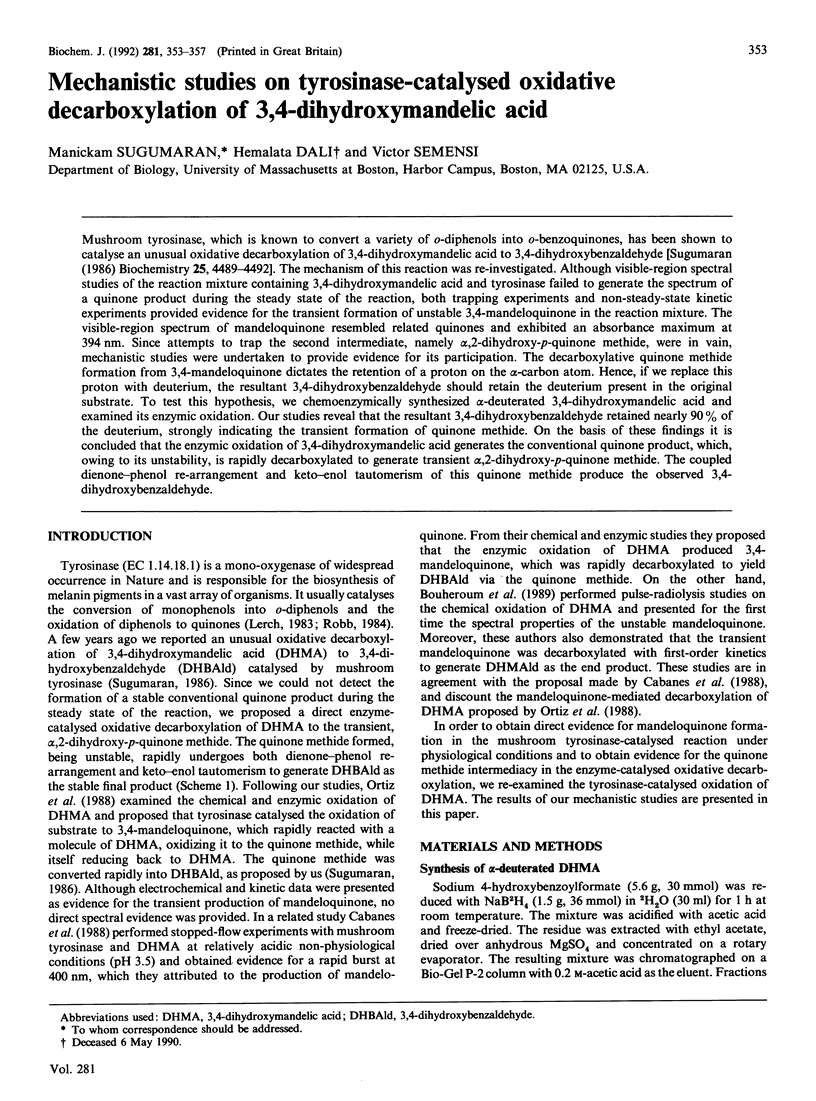
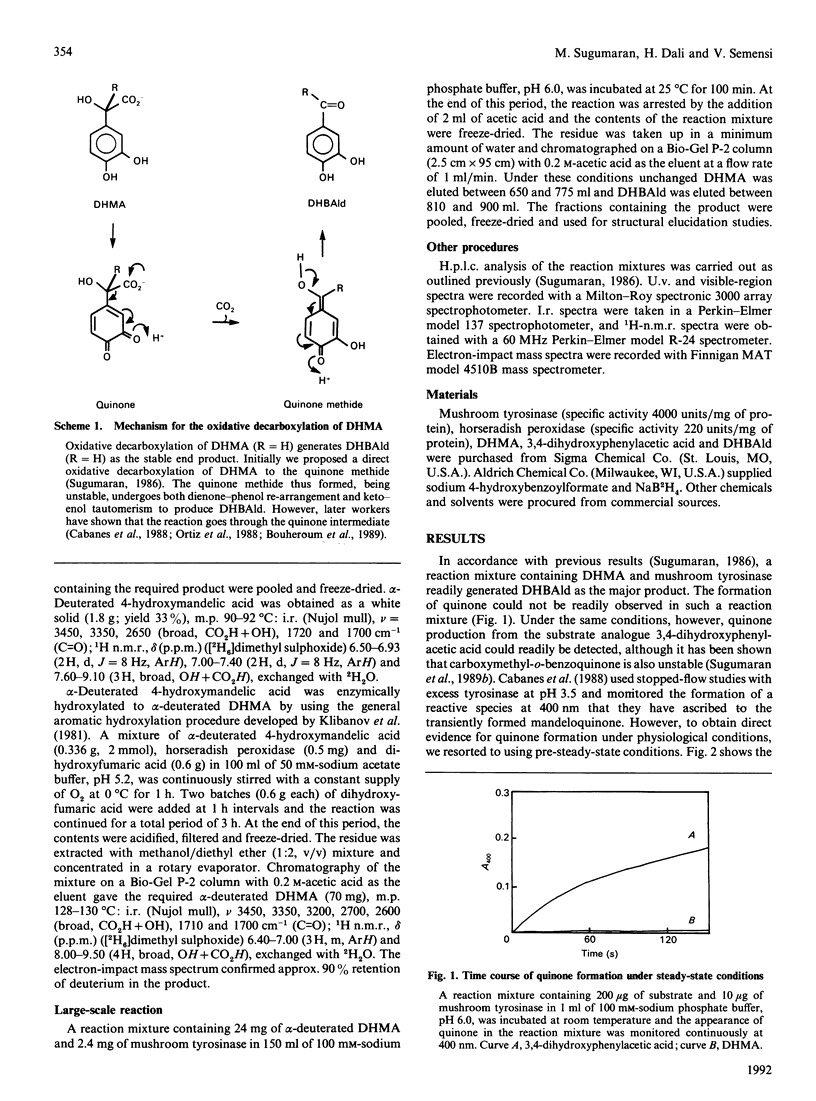
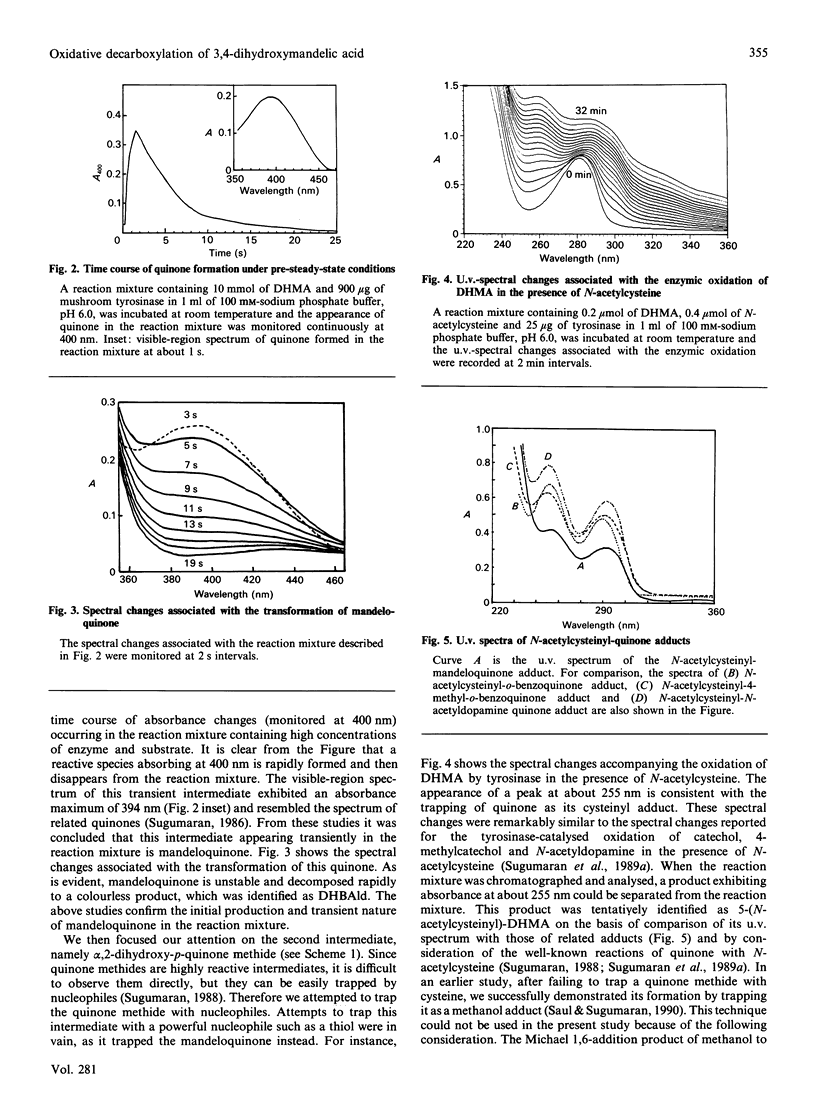
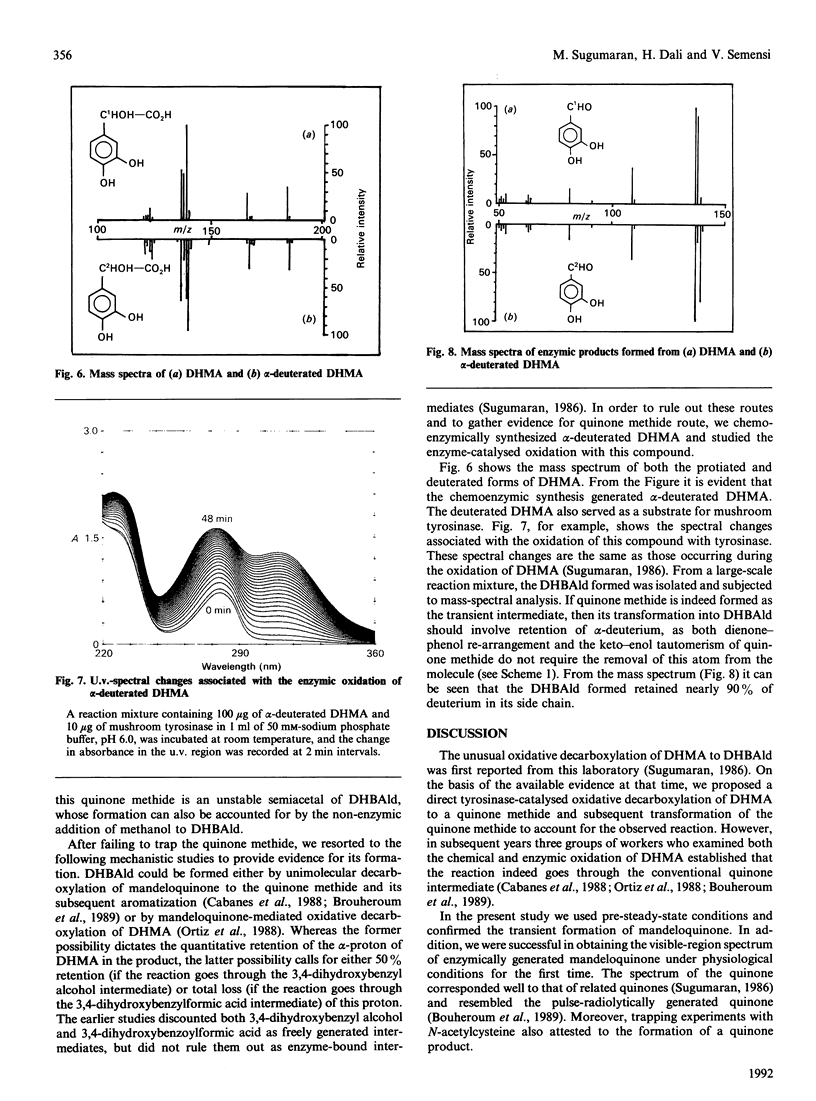
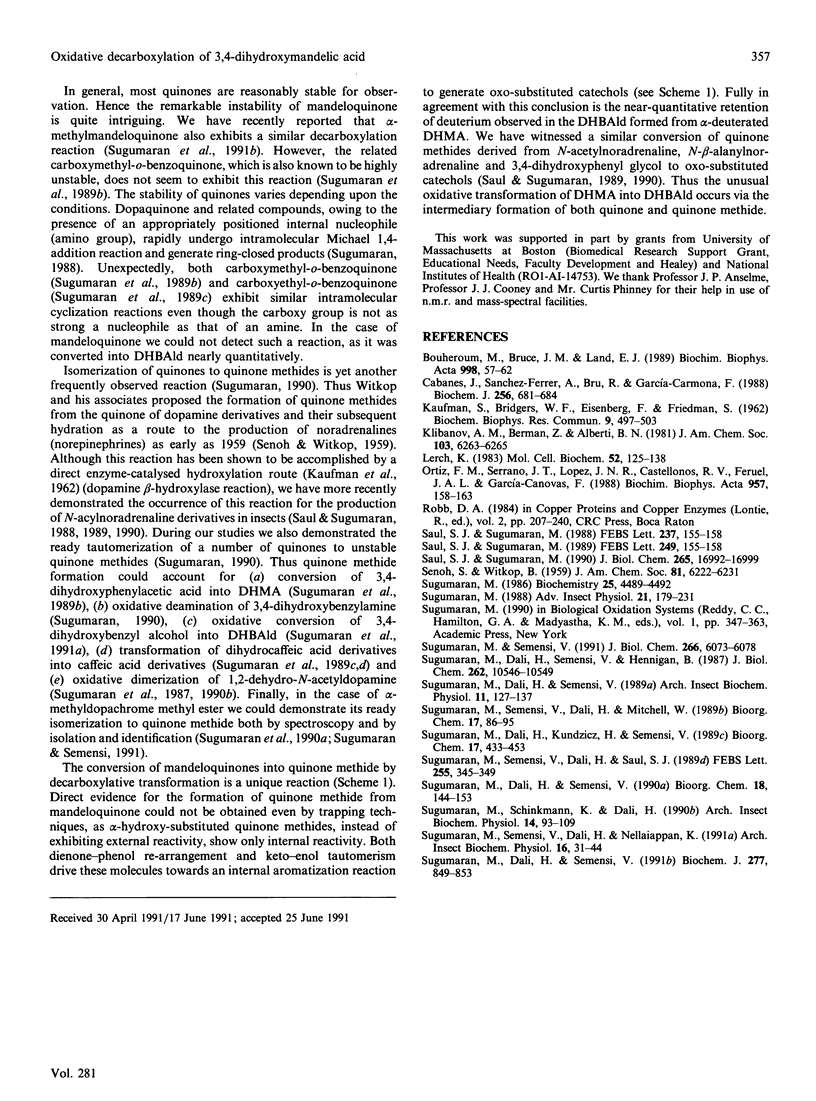
Images in this article
Selected References
These references are in PubMed. This may not be the complete list of references from this article.
- Cabanes J., Sanchez-Ferrer A., Bru R., García-Carmona F. Chemical and enzymic oxidation by tyrosinase of 3,4-dihydroxymandelate. Biochem J. 1988 Dec 1;256(2):681–684. doi: 10.1042/bj2560681. [DOI] [PMC free article] [PubMed] [Google Scholar]
- KAUFMAN S., BRIDGERS W. F., EISENBERG F., FRIEDMAN S. The source of oxygen in the phenylalanine hydroxylase and the copamine-beta-hydroxylase catalyzed rections. Biochem Biophys Res Commun. 1962 Dec 19;9:497–502. doi: 10.1016/0006-291x(62)90115-8. [DOI] [PubMed] [Google Scholar]
- Lerch K. Neurospora tyrosinase: structural, spectroscopic and catalytic properties. Mol Cell Biochem. 1983;52(2):125–138. doi: 10.1007/BF00224921. [DOI] [PubMed] [Google Scholar]
- Martínez Ortiz F., Tudela Serrano J., Rodríguez López J. N., Varón Castellanos R., Lozano Teruel J. A., García-Cánovas F. Oxidation of 3,4-dihydroxymandelic acid catalyzed by tyrosinase. Biochim Biophys Acta. 1988 Nov 2;957(1):158–163. doi: 10.1016/0167-4838(88)90169-0. [DOI] [PubMed] [Google Scholar]
- Saul S. J., Sugumaran M. 4-alkyl-o-quinone/2-hydroxy-p-quinone methide isomerase from the larval hemolymph of Sarcophaga bullata. I. Purification and characterization of enzyme-catalyzed reaction. J Biol Chem. 1990 Oct 5;265(28):16992–16999. [PubMed] [Google Scholar]
- Saul S. J., Sugumaran M. o-quinone/quinone methide isomerase: a novel enzyme preventing the destruction of self-matter by phenoloxidase-generated quinones during immune response in insects. FEBS Lett. 1989 Jun 5;249(2):155–158. doi: 10.1016/0014-5793(89)80614-3. [DOI] [PubMed] [Google Scholar]
- Saul S., Sugumaran M. A novel quinone: quinone methide isomerase generates quinone methides in insect cuticle. FEBS Lett. 1988 Sep 12;237(1-2):155–158. doi: 10.1016/0014-5793(88)80191-1. [DOI] [PubMed] [Google Scholar]
- Sugumaran M., Dali H., Semensi V., Hennigan B. Tyrosinase-catalyzed unusual oxidative dimerization of 1,2-dehydro-N-acetyldopamine. J Biol Chem. 1987 Aug 5;262(22):10546–10549. [PubMed] [Google Scholar]
- Sugumaran M., Dali H., Semensi V. The mechanism of tyrosinase-catalysed oxidative decarboxylation of alpha-(3,4-dihydroxyphenyl)-lactic acid. Biochem J. 1991 Aug 1;277(Pt 3):849–853. doi: 10.1042/bj2770849. [DOI] [PMC free article] [PubMed] [Google Scholar]
- Sugumaran M., Schinkmann K., Dali H. Mechanism of activation of 1,2-dehydro-N-acetyldopamine for cuticular sclerotization. Arch Insect Biochem Physiol. 1990;14(2):93–109. doi: 10.1002/arch.940140205. [DOI] [PubMed] [Google Scholar]
- Sugumaran M., Semensi V., Dali H., Nellaiappan K. Oxidation of 3,4-dihydroxybenzyl alcohol: a sclerotizing precursor for cockroach ootheca. Arch Insect Biochem Physiol. 1991;16(1):31–44. doi: 10.1002/arch.940160105. [DOI] [PubMed] [Google Scholar]
- Sugumaran M., Semensi V. Quinone methide as a new intermediate in eumelanin biosynthesis. J Biol Chem. 1991 Apr 5;266(10):6073–6078. [PubMed] [Google Scholar]
- Sugumaran M. Tyrosinase catalyzes an unusual oxidative decarboxylation of 3,4-dihydroxymandelate. Biochemistry. 1986 Aug 12;25(16):4489–4492. doi: 10.1021/bi00364a005. [DOI] [PubMed] [Google Scholar]