Abstract
Poly(A)+ mRNA was isolated from rabbit kidney cortex and injected into Xenopus laevis oocytes. Injection of mRNA resulted in a time- and dose-dependent increase in Na(+)-independent uptake of L-[3H]alanine and L-[3H]arginine. L-Alanine uptake was stimulated about 3-fold and L-arginine uptake was stimulated about 8-fold after injection of mRNA (25-50 ng, after 3-6 days) as compared with water-injected oocytes. T.I.C. of oocyte extracts suggested that the increased uptake actually represented an increase in the oocyte content of labelled L-alanine and L-arginine. The expressed L-alanine uptake, obtained by subtracting the uptake in water-injected oocytes from that in mRNA-injected oocytes, showed saturability and was inhibited completely by 2-aminobicyclo[2,2,1]heptane-2-carboxylic acid (BCH) and L-arginine. The expressed L-arginine uptake in mRNA-injected oocytes also showed saturability, being completely inhibited by L-dibasic amino acids) and partially inhibited by BCH. Expression of both L-alanine and L-arginine uptake showed clear cis-inhibition by cationic (e.g. L-arginine) and neutral (e.g. L-leucine) amino acids. In all, this points to the expression of a Na(+)-independent transport system with broad specificity (i.e. b degree, (+)-like). In addition, part of the expressed uptake of L-arginine could be due to a system y(+)-like transporter. After size fractionation through a sucrose density gradient, the mRNA species encoding these increased transport activities (Na(+)-independent transport of L-alanine and of L-arginine) were found in fractions of an average mRNA chain-length of 1.8-2.4 kb. On the basis of these results, we conclude that Na(+)-independent transport system(s) for L-alanine and L-arginine from rabbit renal cortical tissues, most likely proximal tubules, are expressed in Xenopus laevis oocytes. These observations may represent the first steps towards expression and cloning of these transport pathways.
Full text
PDF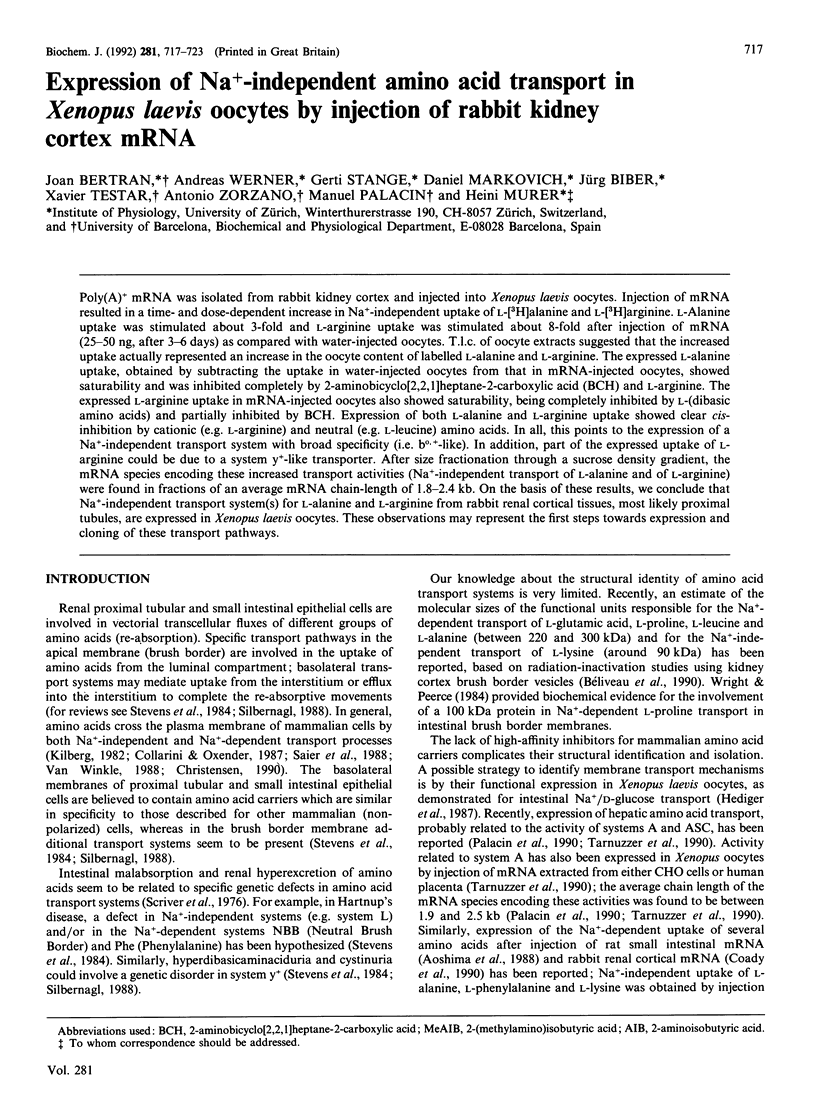
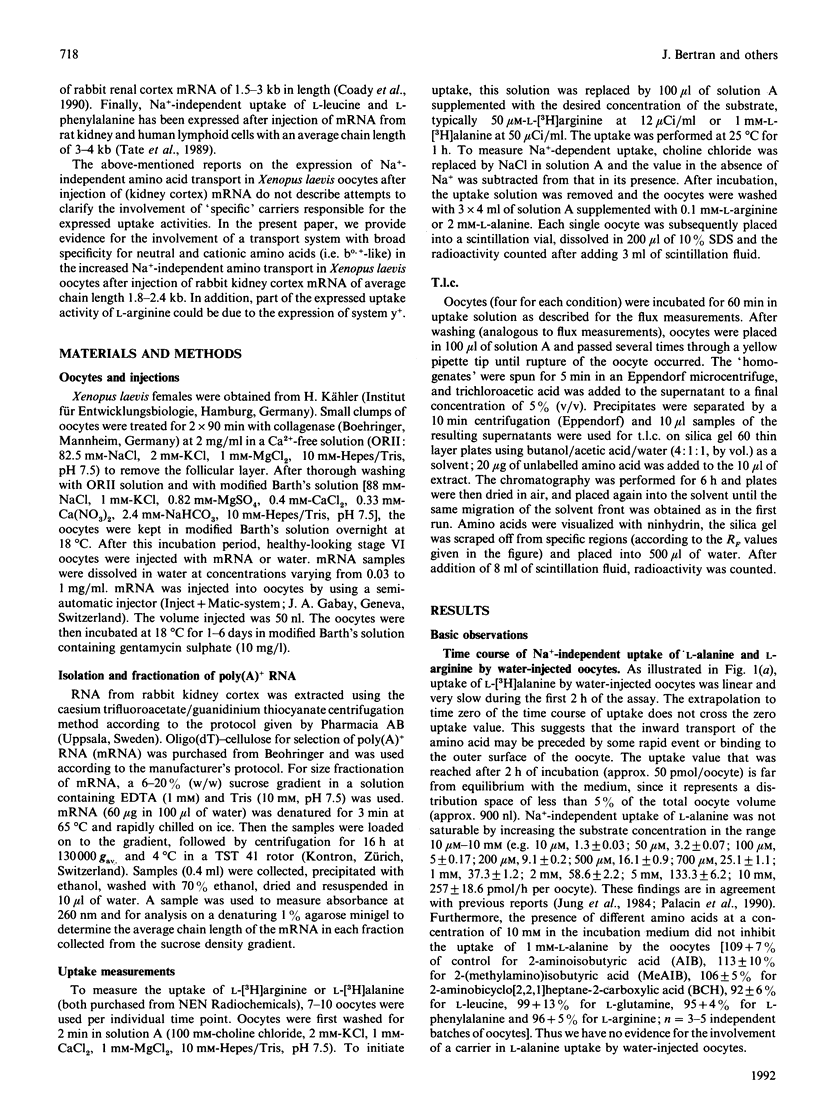
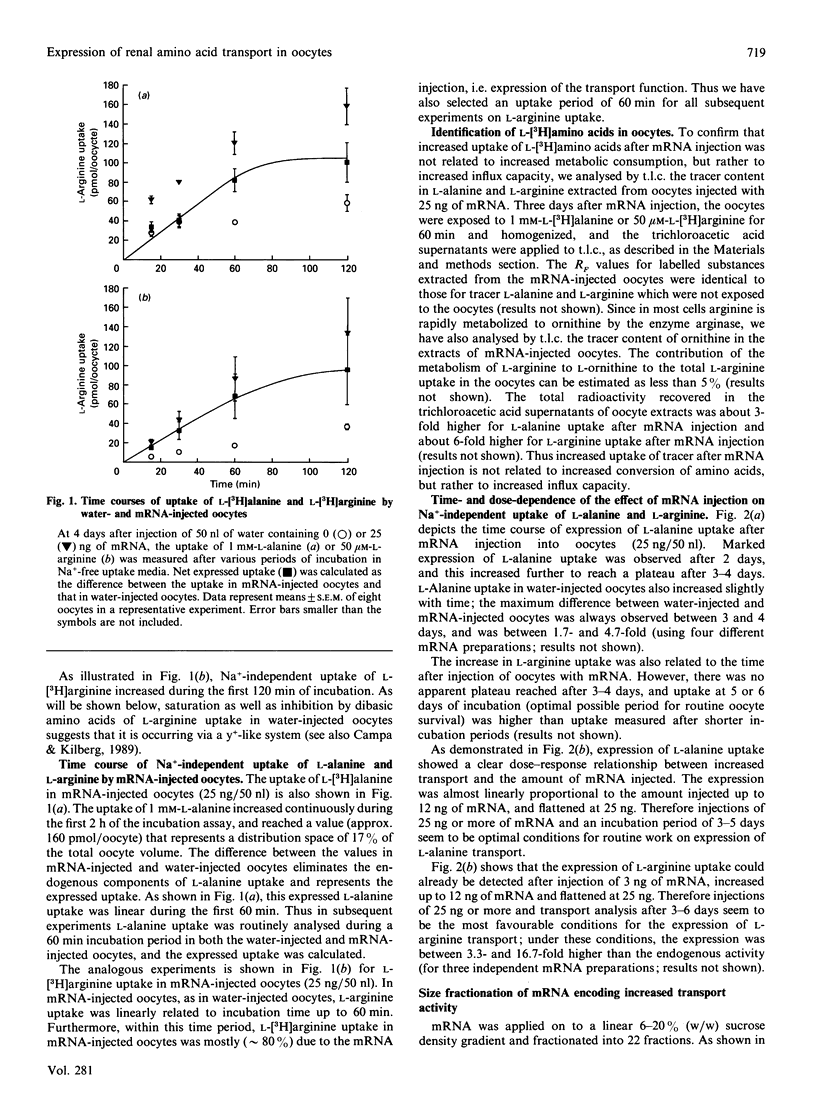
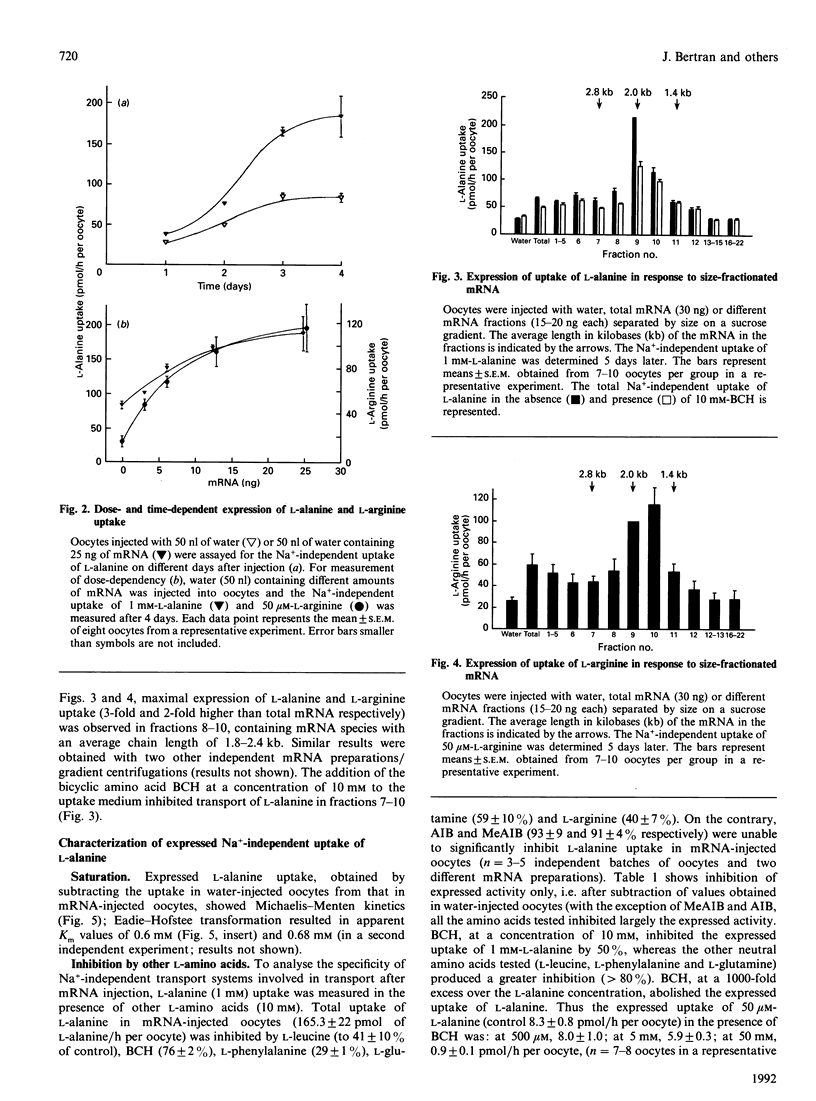
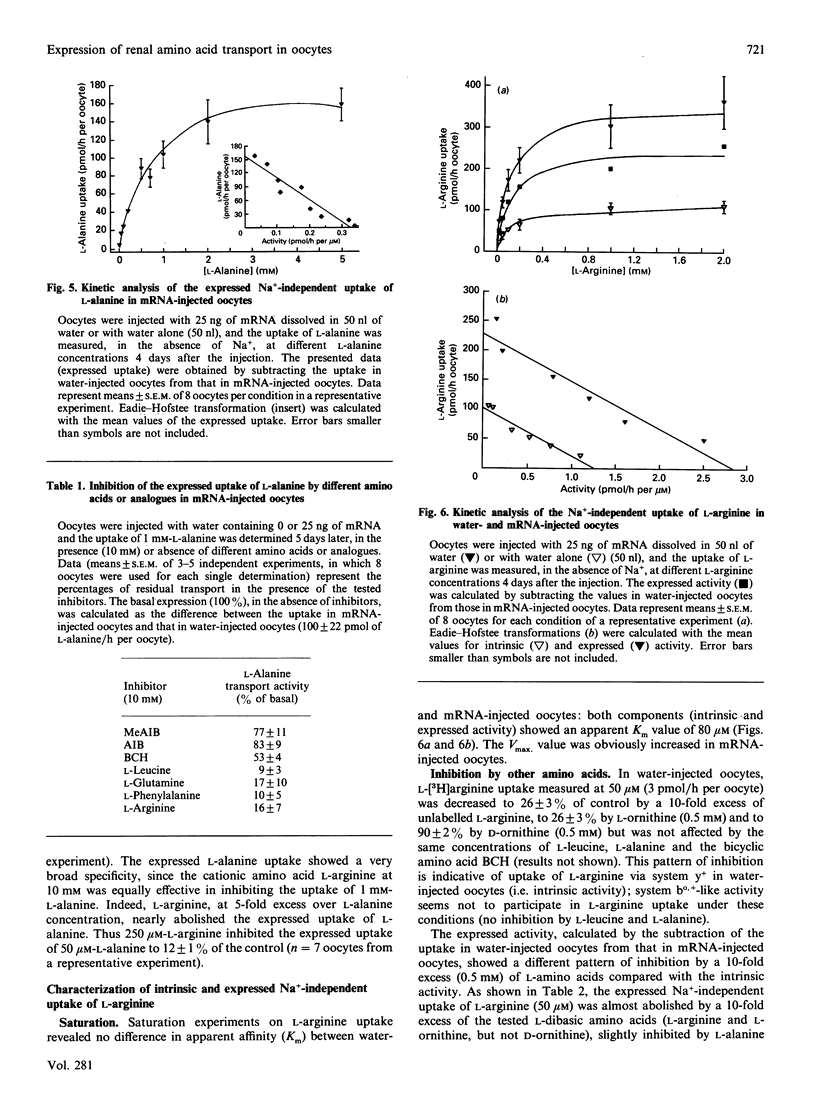
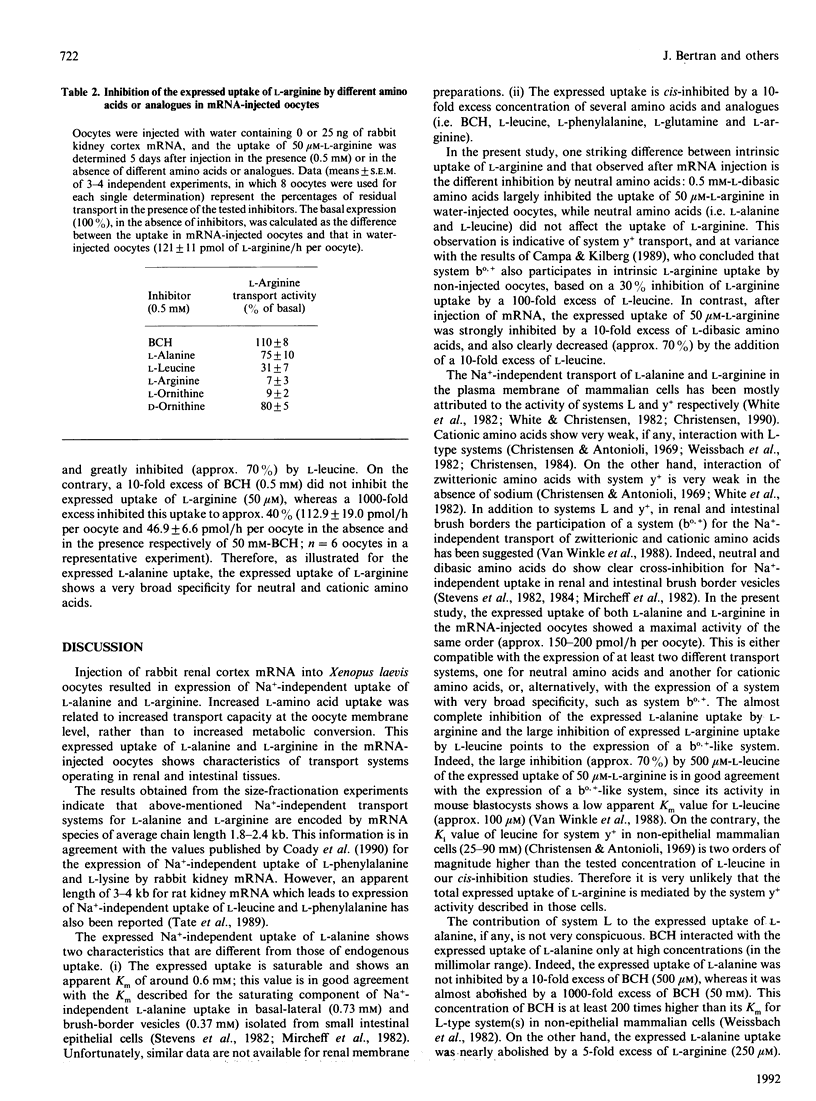
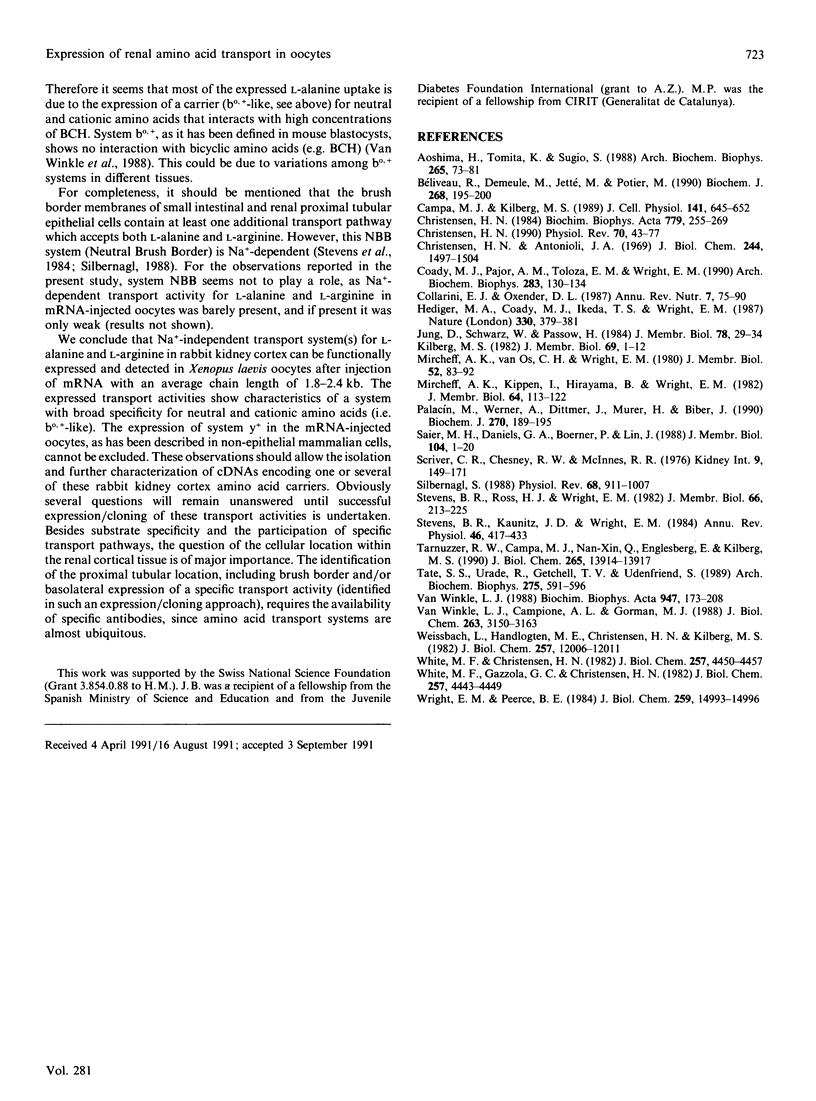
Selected References
These references are in PubMed. This may not be the complete list of references from this article.
- Aoshima H., Tomita K., Sugio S. Expression of amino acid transport systems in Xenopus oocytes injected with mRNA of rat small intestine and kidney. Arch Biochem Biophys. 1988 Aug 15;265(1):73–81. doi: 10.1016/0003-9861(88)90372-4. [DOI] [PubMed] [Google Scholar]
- Béliveau R., Demeule M., Jetté M., Potier M. Molecular sizes of amino acid transporters in the luminal membrane from the kidney cortex, estimated by the radiation-inactivation method. Biochem J. 1990 May 15;268(1):195–200. doi: 10.1042/bj2680195. [DOI] [PMC free article] [PubMed] [Google Scholar]
- Campa M. J., Kilberg M. S. Characterization of neutral and cationic amino acid transport in Xenopus oocytes. J Cell Physiol. 1989 Dec;141(3):645–652. doi: 10.1002/jcp.1041410324. [DOI] [PubMed] [Google Scholar]
- Christensen H. N., Antonioli J. A. Cationic amino acid transport in the rabbit reticulocyte. Na+-dependent inhibition of Na+-independent transport. J Biol Chem. 1969 Mar 25;244(6):1497–1504. [PubMed] [Google Scholar]
- Christensen H. N. Organic ion transport during seven decades. The amino acids. Biochim Biophys Acta. 1984 Sep 3;779(3):255–269. doi: 10.1016/0304-4157(84)90012-1. [DOI] [PubMed] [Google Scholar]
- Christensen H. N. Role of amino acid transport and countertransport in nutrition and metabolism. Physiol Rev. 1990 Jan;70(1):43–77. doi: 10.1152/physrev.1990.70.1.43. [DOI] [PubMed] [Google Scholar]
- Coady M. J., Pajor A. M., Toloza E. M., Wright E. M. Expression of mammalian renal transporters in Xenopus laevis oocytes. Arch Biochem Biophys. 1990 Nov 15;283(1):130–134. doi: 10.1016/0003-9861(90)90622-6. [DOI] [PubMed] [Google Scholar]
- Collarini E. J., Oxender D. L. Mechanisms of transport of amino acids across membranes. Annu Rev Nutr. 1987;7:75–90. doi: 10.1146/annurev.nu.07.070187.000451. [DOI] [PubMed] [Google Scholar]
- Hediger M. A., Coady M. J., Ikeda T. S., Wright E. M. Expression cloning and cDNA sequencing of the Na+/glucose co-transporter. 1987 Nov 26-Dec 2Nature. 330(6146):379–381. doi: 10.1038/330379a0. [DOI] [PubMed] [Google Scholar]
- Jung D., Schwarz W., Passow H. Sodium-alanine cotransport in oocytes of Xenopus laevis: correlation of alanine and sodium fluxes with potential and current changes. J Membr Biol. 1984;78(1):29–34. doi: 10.1007/BF01872529. [DOI] [PubMed] [Google Scholar]
- Kilberg M. S. Amino acid transport in isolated rat hepatocytes. J Membr Biol. 1982;69(1):1–12. doi: 10.1007/BF01871236. [DOI] [PubMed] [Google Scholar]
- Mircheff A. K., Kippen I., Hirayama B., Wright E. M. Delineation of sodium-stimulated amino acid transport pathways in rabbit kidney brush border vesicles. J Membr Biol. 1982;64(1-2):113–122. doi: 10.1007/BF01870773. [DOI] [PubMed] [Google Scholar]
- Mircheff A. K., van Os C. H., Wright E. M. Pathways for alanine transport in intestinal basal lateral membrane vesicles. J Membr Biol. 1980 Jan 31;52(1):83–92. doi: 10.1007/BF01869009. [DOI] [PubMed] [Google Scholar]
- Palacin M., Werner A., Dittmer J., Murer H., Biber J. Expression of rat liver Na+/L-alanine co-transport in Xenopus laevis oocytes. Effect of glucagon in vivo. Biochem J. 1990 Aug 15;270(1):189–195. doi: 10.1042/bj2700189. [DOI] [PMC free article] [PubMed] [Google Scholar]
- Saier M. H., Jr, Daniels G. A., Boerner P., Lin J. Neutral amino acid transport systems in animal cells: potential targets of oncogene action and regulators of cellular growth. J Membr Biol. 1988 Aug;104(1):1–20. doi: 10.1007/BF01871898. [DOI] [PubMed] [Google Scholar]
- Scriver C. R., Chesney R. W., McInnes R. R. Genetic aspects of renal tubular transport: diversity and topology of carriers. Kidney Int. 1976 Feb;9(2):149–171. doi: 10.1038/ki.1976.18. [DOI] [PubMed] [Google Scholar]
- Silbernagl S. The renal handling of amino acids and oligopeptides. Physiol Rev. 1988 Jul;68(3):911–1007. doi: 10.1152/physrev.1988.68.3.911. [DOI] [PubMed] [Google Scholar]
- Stevens B. R., Kaunitz J. D., Wright E. M. Intestinal transport of amino acids and sugars: advances using membrane vesicles. Annu Rev Physiol. 1984;46:417–433. doi: 10.1146/annurev.ph.46.030184.002221. [DOI] [PubMed] [Google Scholar]
- Stevens B. R., Ross H. J., Wright E. M. Multiple transport pathways for neutral amino acids in rabbit jejunal brush border vesicles. J Membr Biol. 1982;66(3):213–225. doi: 10.1007/BF01868496. [DOI] [PubMed] [Google Scholar]
- Tarnuzzer R. W., Campa M. J., Qian N. X., Englesberg E., Kilberg M. S. Expression of the mammalian system A neutral amino acid transporter in Xenopus oocytes. J Biol Chem. 1990 Aug 15;265(23):13914–13917. [PubMed] [Google Scholar]
- Tate S. S., Urade R., Getchell T. V., Udenfriend S. Expression of the mammalian Na+-independent L system amino acid transporter in Xenopus laevis oocytes. Arch Biochem Biophys. 1989 Dec;275(2):591–596. doi: 10.1016/0003-9861(89)90405-0. [DOI] [PubMed] [Google Scholar]
- Van Winkle L. J. Amino acid transport in developing animal oocytes and early conceptuses. Biochim Biophys Acta. 1988 Feb 24;947(1):173–208. doi: 10.1016/0304-4157(88)90024-x. [DOI] [PubMed] [Google Scholar]
- Van Winkle L. J., Campione A. L., Gorman J. M. Na+-independent transport of basic and zwitterionic amino acids in mouse blastocysts by a shared system and by processes which distinguish between these substrates. J Biol Chem. 1988 Mar 5;263(7):3150–3163. [PubMed] [Google Scholar]
- Weissbach L., Handlogten M. E., Christensen H. N., Kilberg M. S. Evidence for two Na+-independent neutral amino acid transport systems in primary cultures of rat hepatocytes. Time-dependent changes in activity. J Biol Chem. 1982 Oct 25;257(20):12006–12011. [PubMed] [Google Scholar]
- White M. F., Christensen H. N. Cationic amino acid transport into cultured animal cells. II. Transport system barely perceptible in ordinary hepatocytes, but active in hepatoma cell lines. J Biol Chem. 1982 Apr 25;257(8):4450–4457. [PubMed] [Google Scholar]
- White M. F., Gazzola G. C., Christensen H. N. Cationic amino acid transport into cultured animal cells. I. Influx into cultured human fibroblasts. J Biol Chem. 1982 Apr 25;257(8):4443–4449. [PubMed] [Google Scholar]
- Wright E. M., Peerce B. E. Identification and conformational changes of the intestinal proline carrier. J Biol Chem. 1984 Dec 25;259(24):14993–14996. [PubMed] [Google Scholar]