Abstract
In order to gain an insight into the pathogenesis of mouse muscular dystrophy, we investigated the natural suppressor serine tRNA. The natural suppressor seryl-tRNA was distinguished from the other seryl-tRNAs on the basis of its specific property of being converted into phosphoseryl-tRNA by a tRNA kinase. On a wet-weight basis, the content of total tRNA in dystrophic muscles was 47% of that in normal muscles. Although the serine-accepting activities of tRNA were similar in muscles of 3-month-old dystrophic and normal mice, the ratio of [32P]phosphoseryl-tRNA (suppressor tRNA) to the total serine tRNA was significantly enhanced in dystrophic muscles compared with that in normal muscles. This high content of suppressor tRNA in dystrophic muscles was further confirmed by dot-blot hybridization experiments with the DNA probes CGTAGTCGGCAGGAT and CGCCCGAAAGGTGGAA for major tRNA(IGASer) and suppressor tRNA respectively. At the early postnatal age of 3 weeks, when only a week had elapsed since the first manifestation of the dystrophic symptom (hindleg dragging), the ratio of suppressor tRNA to major tRNAs in dystrophic hindleg muscles was abnormally increased. Thereafter it decreased with age in normal mice but remained almost unchanged in dystrophic mice. Consequently, at 3 months old, it was 1.7 times higher in dystrophic than in normal mice. The suppressor tRNA is now accepted to play a role in the synthesis of glutathione peroxidase. The present study showed that the content of this enzyme was abnormally elevated in dystrophic mice. Previously we had demonstrated that the docosahexaenoic (C22:6) acid content in phospholipids was decreased, possibly resulting from the enhanced oxidative milieu caused by the dystrophic condition. Thus far, the findings suggest that an increase in the contents of suppressor tRNA and glutathione peroxidase in dystrophic muscle may have been secondarily induced by such a highly oxidative state in the dystrophic condition. However, it is difficult to exclude the possibility that the natural suppressor tRNA plays a primary role in the pathogenesis of muscular dystrophies.
Full text
PDF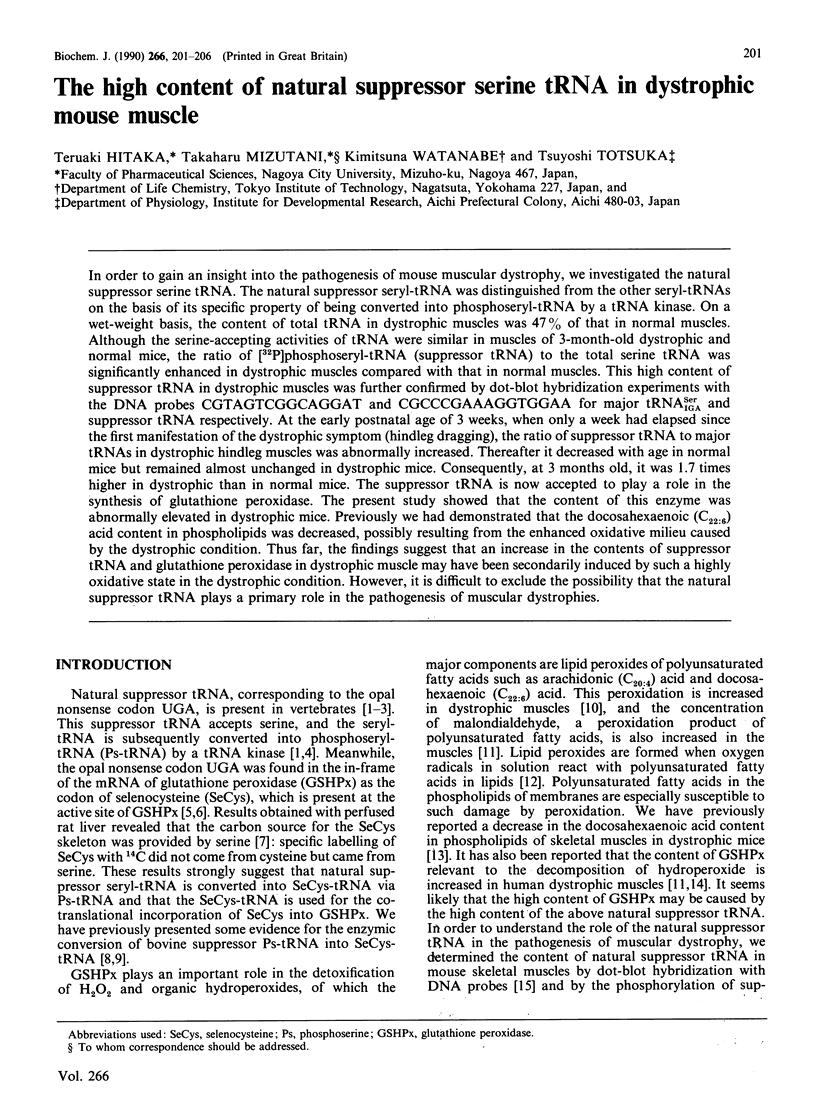
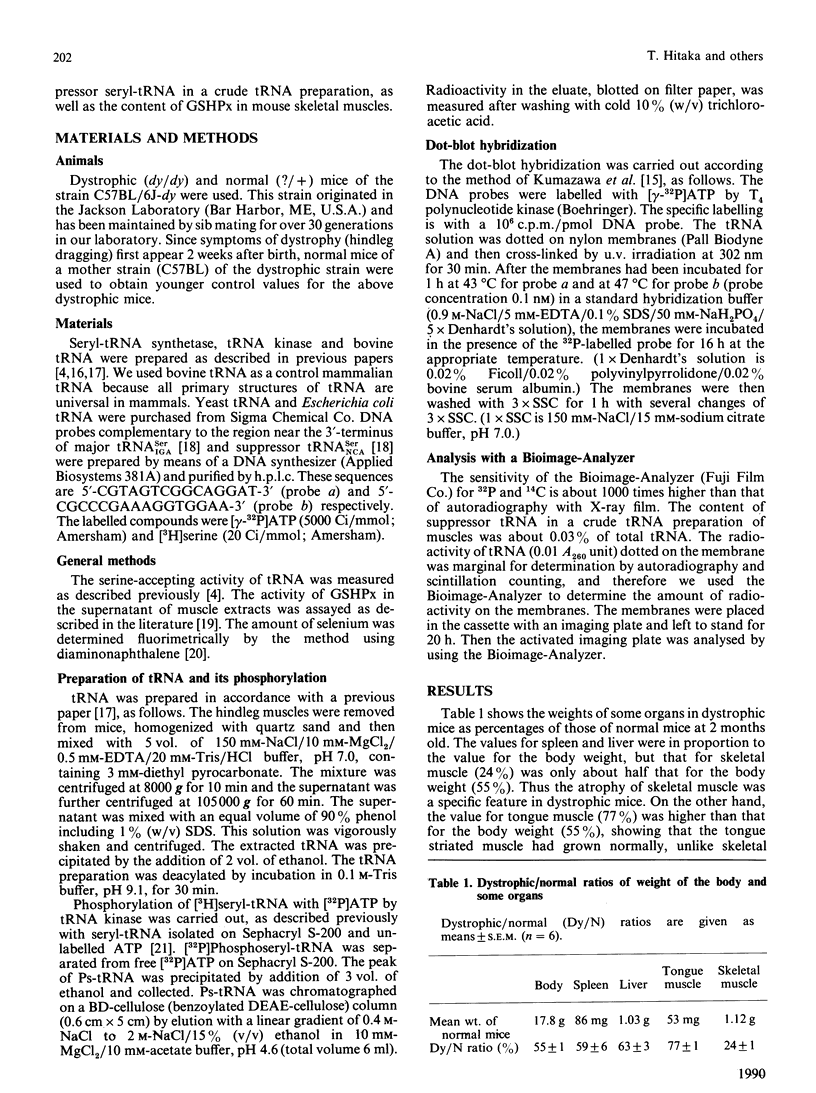
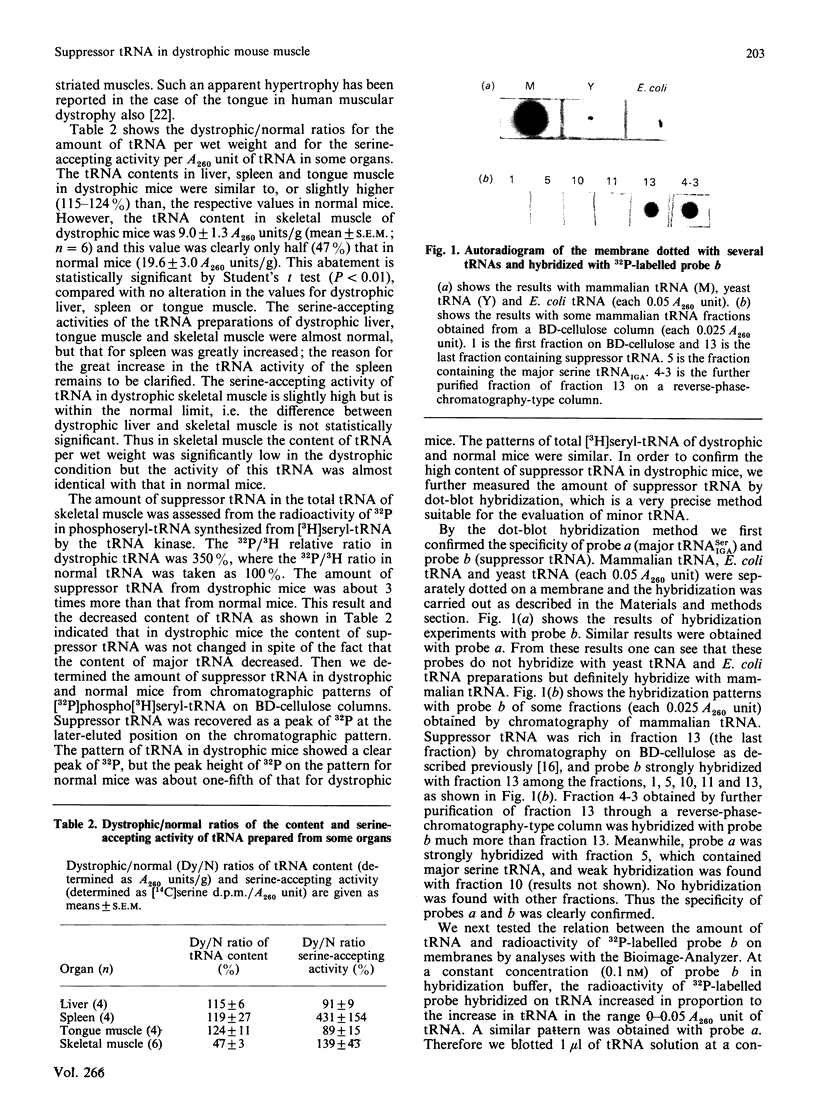
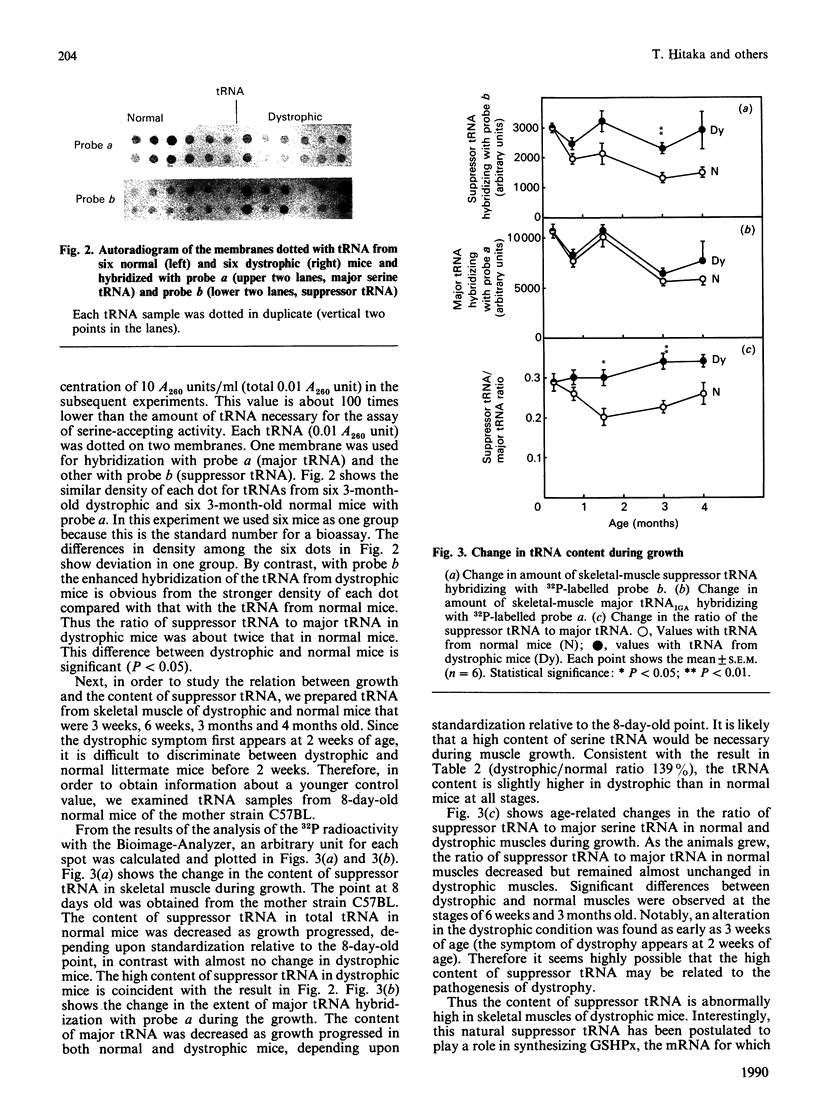
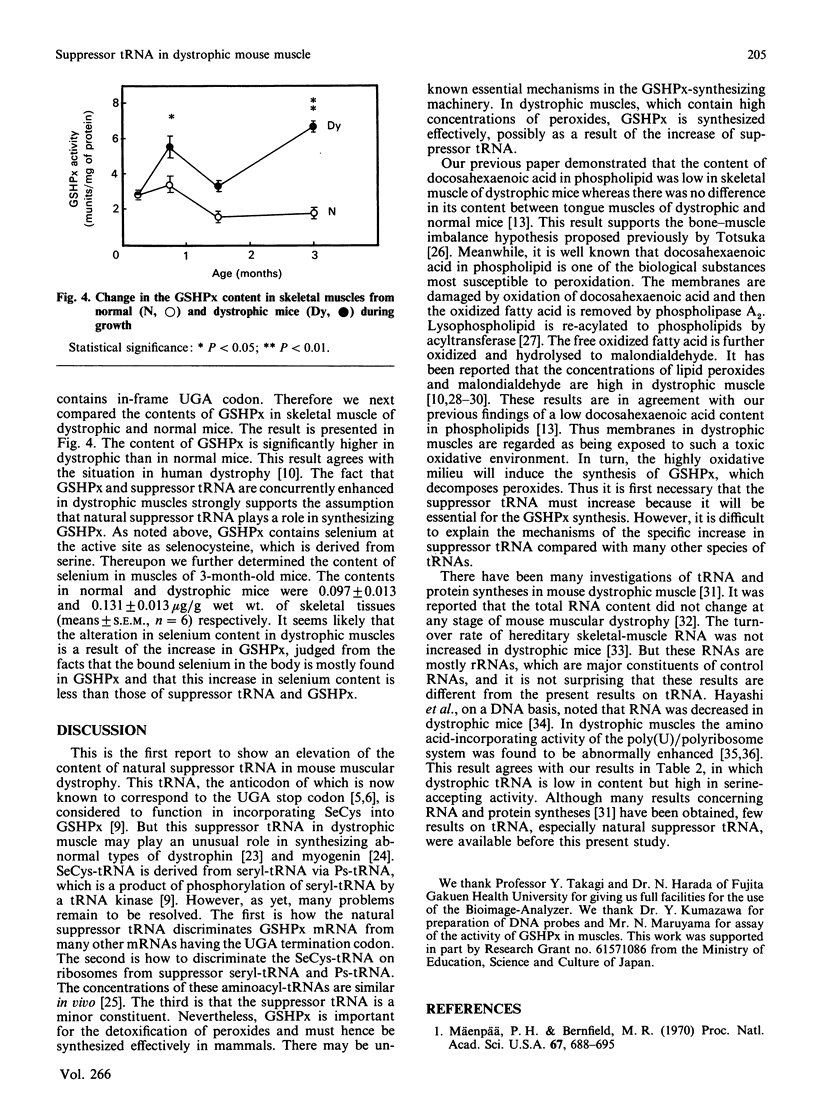
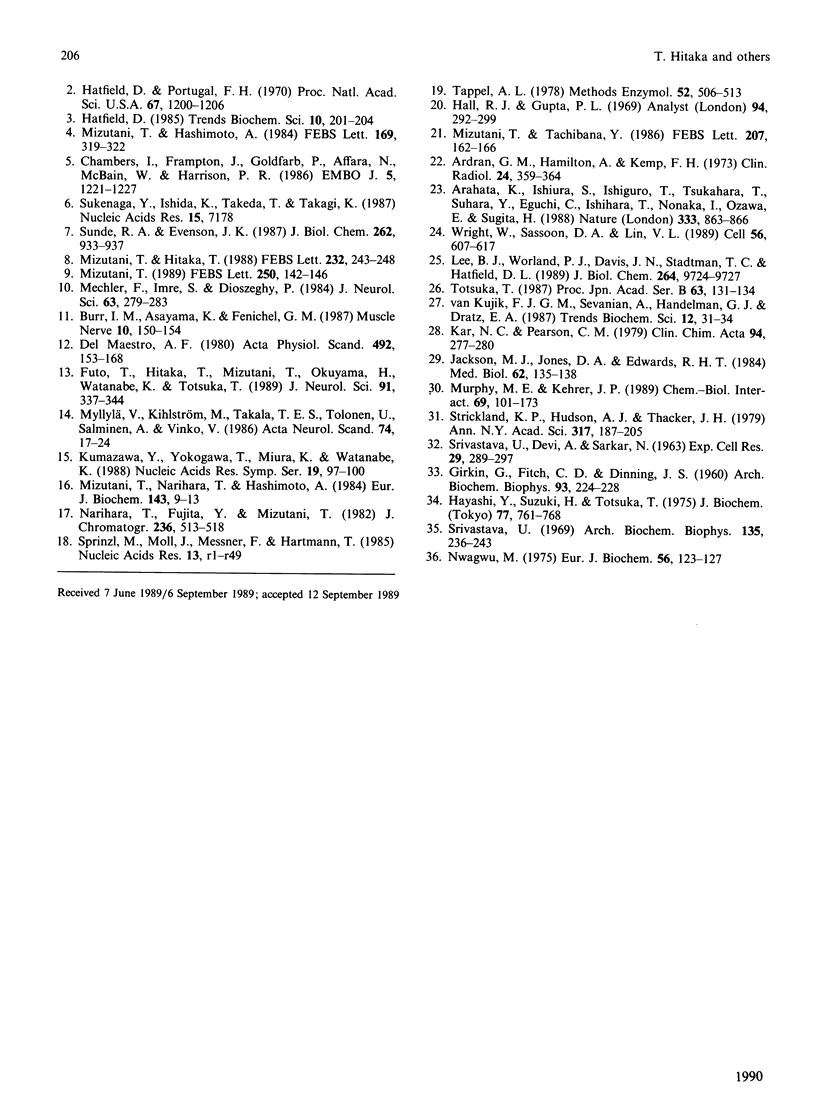
Images in this article
Selected References
These references are in PubMed. This may not be the complete list of references from this article.
- Arahata K., Ishiura S., Ishiguro T., Tsukahara T., Suhara Y., Eguchi C., Ishihara T., Nonaka I., Ozawa E., Sugita H. Immunostaining of skeletal and cardiac muscle surface membrane with antibody against Duchenne muscular dystrophy peptide. Nature. 1988 Jun 30;333(6176):861–863. doi: 10.1038/333861a0. [DOI] [PubMed] [Google Scholar]
- Ardran G. M., Hamilton A., Kemp F. H. Enlargement of the tongue and changes in the jaws with muscular dystrophy. Clin Radiol. 1973 Jul;24(3):359–364. doi: 10.1016/s0009-9260(73)80058-3. [DOI] [PubMed] [Google Scholar]
- Burr I. M., Asayama K., Fenichel G. M. Superoxide dismutases, glutathione peroxidase, and catalase in neuromuscular disease. Muscle Nerve. 1987 Feb;10(2):150–154. doi: 10.1002/mus.880100208. [DOI] [PubMed] [Google Scholar]
- Chambers I., Frampton J., Goldfarb P., Affara N., McBain W., Harrison P. R. The structure of the mouse glutathione peroxidase gene: the selenocysteine in the active site is encoded by the 'termination' codon, TGA. EMBO J. 1986 Jun;5(6):1221–1227. doi: 10.1002/j.1460-2075.1986.tb04350.x. [DOI] [PMC free article] [PubMed] [Google Scholar]
- Del Maestro R. F. An approach to free radicals in medicine and biology. Acta Physiol Scand Suppl. 1980;492:153–168. [PubMed] [Google Scholar]
- Futo T., Hitaka T., Mizutani T., Okuyama H., Watanabe K., Totsuka T. Fatty acid composition of lipids in tongue and hindleg muscles of muscular dystrophic mice. J Neurol Sci. 1989 Jul;91(3):337–344. doi: 10.1016/0022-510x(89)90062-2. [DOI] [PubMed] [Google Scholar]
- Hall R. J., Gupta P. L. The determination of very small amounts of selenium in plant samples. Analyst. 1969 Apr;94(117):292–299. doi: 10.1039/an9699400292. [DOI] [PubMed] [Google Scholar]
- Hatfield D., Portugal F. H. Seryl-tRNA in mammalian tissues: chromatographic differences in brain and liver and a specific response to the codon, UGA. Proc Natl Acad Sci U S A. 1970 Nov;67(3):1200–1206. doi: 10.1073/pnas.67.3.1200. [DOI] [PMC free article] [PubMed] [Google Scholar]
- Hayashi Y., Suzuki H. O., Totsuka T. Protein and RNA synthesis in the skeletal muscle of hereditary dystrophic mouse. J Biochem. 1975 Apr;77(4):761–768. doi: 10.1093/oxfordjournals.jbchem.a130780. [DOI] [PubMed] [Google Scholar]
- Jackson M. J., Jones D. A., Edwards R. H. Techniques for studying free radical damage in muscular dystrophy. Med Biol. 1984;62(2):135–138. [PubMed] [Google Scholar]
- Kar N. C., Pearson C. M. Catalase, superoxide dismutase, glutathione reductase and thiobarbituric acid-reactive products in normal and dystrophic human muscle. Clin Chim Acta. 1979 Jun 15;94(3):277–280. doi: 10.1016/0009-8981(79)90076-7. [DOI] [PubMed] [Google Scholar]
- Kumazawa Y., Yokogawa T., Miura K., Watanabe K. Bovine mitochondrial tRNAPhe, tRNASer (AGY) and tRNASer (UCN): preparation using a new detection method and their properties in aminoacylation. Nucleic Acids Symp Ser. 1988;(19):97–100. [PubMed] [Google Scholar]
- Lee B. J., Worland P. J., Davis J. N., Stadtman T. C., Hatfield D. L. Identification of a selenocysteyl-tRNA(Ser) in mammalian cells that recognizes the nonsense codon, UGA. J Biol Chem. 1989 Jun 15;264(17):9724–9727. [PubMed] [Google Scholar]
- Mechler F., Imre S., Dioszeghy P. Lipid peroxidation and superoxide dismutase activity in muscle and erythrocytes in Duchenne muscular dystrophy. J Neurol Sci. 1984 Mar;63(3):279–283. doi: 10.1016/0022-510x(84)90150-3. [DOI] [PubMed] [Google Scholar]
- Mizutani T., Hashimoto A. Purification and properties of suppressor seryl-tRNA: ATP phosphotransferase from bovine liver. FEBS Lett. 1984 Apr 24;169(2):319–322. doi: 10.1016/0014-5793(84)80342-7. [DOI] [PubMed] [Google Scholar]
- Mizutani T., Hitaka T. The conversion of phosphoserine residues to selenocysteine residues on an opal suppressor tRNA and casein. FEBS Lett. 1988 May 9;232(1):243–248. doi: 10.1016/0014-5793(88)80425-3. [DOI] [PubMed] [Google Scholar]
- Mizutani T., Narihara T., Hashimoto A. Purification and properties of bovine liver seryl-tRNA synthetase. Eur J Biochem. 1984 Aug 15;143(1):9–13. doi: 10.1111/j.1432-1033.1984.tb08331.x. [DOI] [PubMed] [Google Scholar]
- Mizutani T. Some evidence of the enzymatic conversion of bovine suppressor phosphoseryl-tRNA to selenocysteyl-tRNA. FEBS Lett. 1989 Jul 3;250(2):142–146. doi: 10.1016/0014-5793(89)80707-0. [DOI] [PubMed] [Google Scholar]
- Mizutani T., Tachibana Y. Possible incorporation of phosphoserine into globin readthrough protein via bovine opal suppressor phosphoseryl-tRNA. FEBS Lett. 1986 Oct 20;207(1):162–166. doi: 10.1016/0014-5793(86)80032-1. [DOI] [PubMed] [Google Scholar]
- Murphy M. E., Kehrer J. P. Oxidative stress and muscular dystrophy. Chem Biol Interact. 1989;69(2-3):101–173. doi: 10.1016/0009-2797(89)90075-6. [DOI] [PubMed] [Google Scholar]
- Myllylä V., Kihlström M., Takala T. E., Tolonen U., Salminen A., Vihko V. Activities of some antioxidative and hexose monophosphate shunt enzymes of skeletal muscle in neuromuscular diseases. Acta Neurol Scand. 1986 Jul;74(1):17–24. doi: 10.1111/j.1600-0404.1986.tb04619.x. [DOI] [PubMed] [Google Scholar]
- Mäenpä P. H., Bernfield M. R. A specific hepatic transfer RNA for phosphoserine. Proc Natl Acad Sci U S A. 1970 Oct;67(2):688–695. doi: 10.1073/pnas.67.2.688. [DOI] [PMC free article] [PubMed] [Google Scholar]
- Nwagwu M. Activity of polyribosomes from the muscle of normal and dystrophic mice in cell-free amino-acid incorporation. Eur J Biochem. 1975 Aug 1;56(1):123–127. doi: 10.1111/j.1432-1033.1975.tb02214.x. [DOI] [PubMed] [Google Scholar]
- SRIVASTAVA U., DEVI A., SARKAR N. Biochemical changes in progressive muscular dystrophy. I. Nucleic acid metabolism in normal and dystrophic rabbit and mouse liver, brain and muscle. Exp Cell Res. 1963 Jan;29:289–297. doi: 10.1016/0014-4827(63)90384-7. [DOI] [PubMed] [Google Scholar]
- Sprinzl M., Moll J., Meissner F., Hartmann T. Compilation of tRNA sequences. Nucleic Acids Res. 1985;13 (Suppl):r1–49. doi: 10.1093/nar/13.suppl.r1. [DOI] [PMC free article] [PubMed] [Google Scholar]
- Srivastava U. Biochemical changes in progressive muscular dystrophy. 8. Protein synthesis in skeletal muscle of mouse as a function of muscular dystrophy. Arch Biochem Biophys. 1969 Dec;135(1):237–243. doi: 10.1016/0003-9861(69)90535-9. [DOI] [PubMed] [Google Scholar]
- Strickland K. P., Hudson A. J., Thakar J. H. Biochemical studies in dystrophic mouse muscle. Ann N Y Acad Sci. 1979;317:187–205. doi: 10.1111/j.1749-6632.1979.tb56526.x. [DOI] [PubMed] [Google Scholar]
- Sukenaga Y., Ishida K., Takeda T., Takagi K. cDNA sequence coding for human glutathione peroxidase. Nucleic Acids Res. 1987 Sep 11;15(17):7178–7178. doi: 10.1093/nar/15.17.7178. [DOI] [PMC free article] [PubMed] [Google Scholar]
- Sunde R. A., Evenson J. K. Serine incorporation into the selenocysteine moiety of glutathione peroxidase. J Biol Chem. 1987 Jan 15;262(2):933–937. [PubMed] [Google Scholar]
- Tappel A. L. Glutathione peroxidase and hydroperoxides. Methods Enzymol. 1978;52:506–513. doi: 10.1016/s0076-6879(78)52055-7. [DOI] [PubMed] [Google Scholar]
- Wright W. E., Sassoon D. A., Lin V. K. Myogenin, a factor regulating myogenesis, has a domain homologous to MyoD. Cell. 1989 Feb 24;56(4):607–617. doi: 10.1016/0092-8674(89)90583-7. [DOI] [PubMed] [Google Scholar]