Abstract
1. Isolated rat liver and heart mitochondria incubated in 150 mM-KSCN or sucrose medium in the presence of respiratory-chain inhibitors showed a large increase in swelling when exposed to 250 microM-Ca2+. Swelling was inhibited by bongkrekic acid and cyclosporin A in both media and by ADP in KSCN medium; the effect of ADP was reversed by carboxyatractyloside. These results demonstrate that this is a suitable technique with which to study the opening of the Ca2(+)-induced non-specific pore of the mitochondrial inner membrane and implicate the adenine nucleotide carrier in this process. 2. Titration of the rate of swelling with increasing concentrations of cyclosporin showed the number of cyclosporin-binding sites (+/- S.E.M.) in liver and heart mitochondria to be respectively 113.7 +/- 5.0 (n = 9) and 124.3 +/- 11.2 (n = 10) pmol/mg of protein, with a Ki of about 5 nM. 3. Liver and heart mitochondrial-matrix fractions were prepared free of membrane and cytosolic contamination and shown to contain cyclosporin-sensitive peptidyl-prolyl cis-trans isomerase (cyclophilin) activity. Titration of isomerase activity with cyclosporin gave values (+/- S.E.M.) of 110.6 +/- 10.1 (n = 5) and 165.4 +/- 15.0 (n = 3) pmol of enzyme/mg of liver and heart mitochondrial protein respectively, with a Ki of 2.5 nM. The similarity of these results to those from the swelling experiments suggest that the isomerase may be involved in the Ca2(+)-induced swelling. 4. The rapid light-scattering change induced in energized heart mitochondria exposed to submicromolar Ca2+ [Halestrap (1987) Biochem. J. 244, 159-164] was inhibited by ADP and bongkrekic acid, the former effect being reversed by carboxyatractyloside. These results suggest an interaction of Ca2+ with the adenine nucleotide carrier when the 'c' conformation. 5. A model is proposed in which mitochondrial peptidyl-prolyl cis-trans isomerase interacts with the adenine nucleotide carrier in the presence of Ca2+ to cause non-specific pore opening. The model also explains the involvement of the adenine nucleotide translocase in the PPi-mediated cyclosporin-insensitive increase in K+ permeability described in the preceding paper [Davidson & Halestrap (1990) Biochem. J. 268, 147-152]. 6. The physiological and pathological implications of the model are discussed in relation to reperfusion injury and cyclosporin toxicity.
Full text
PDF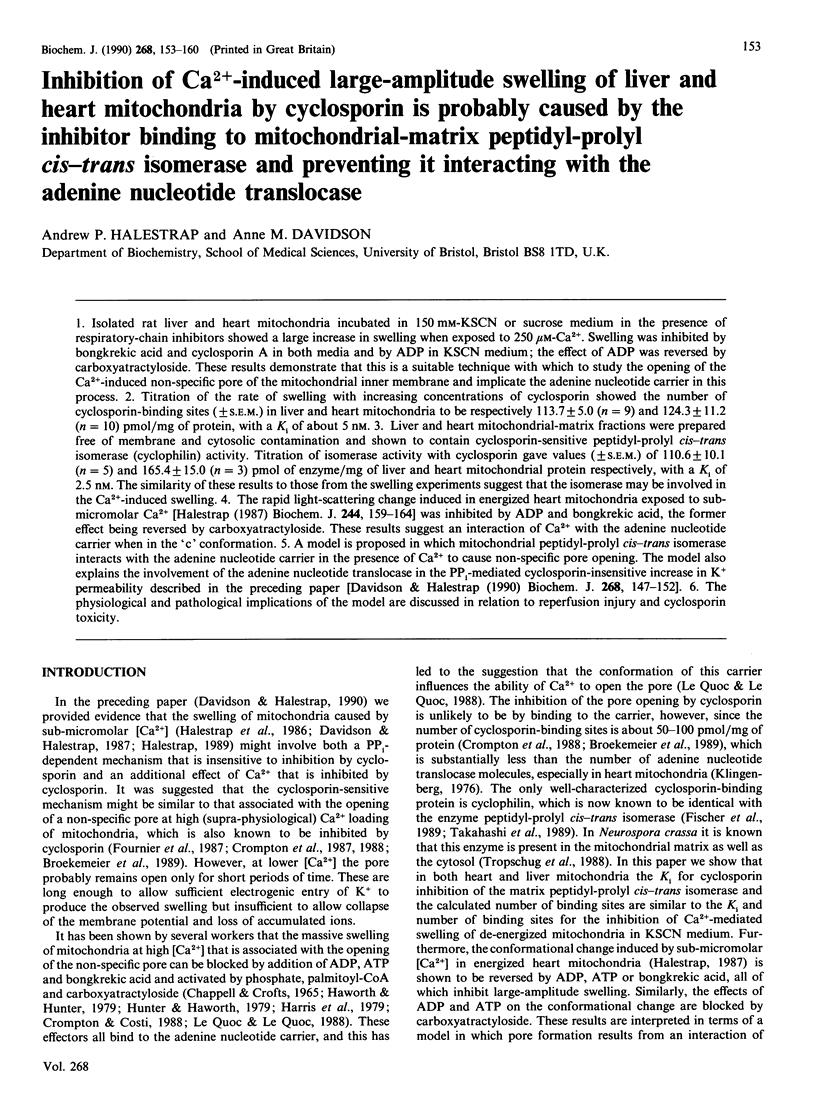
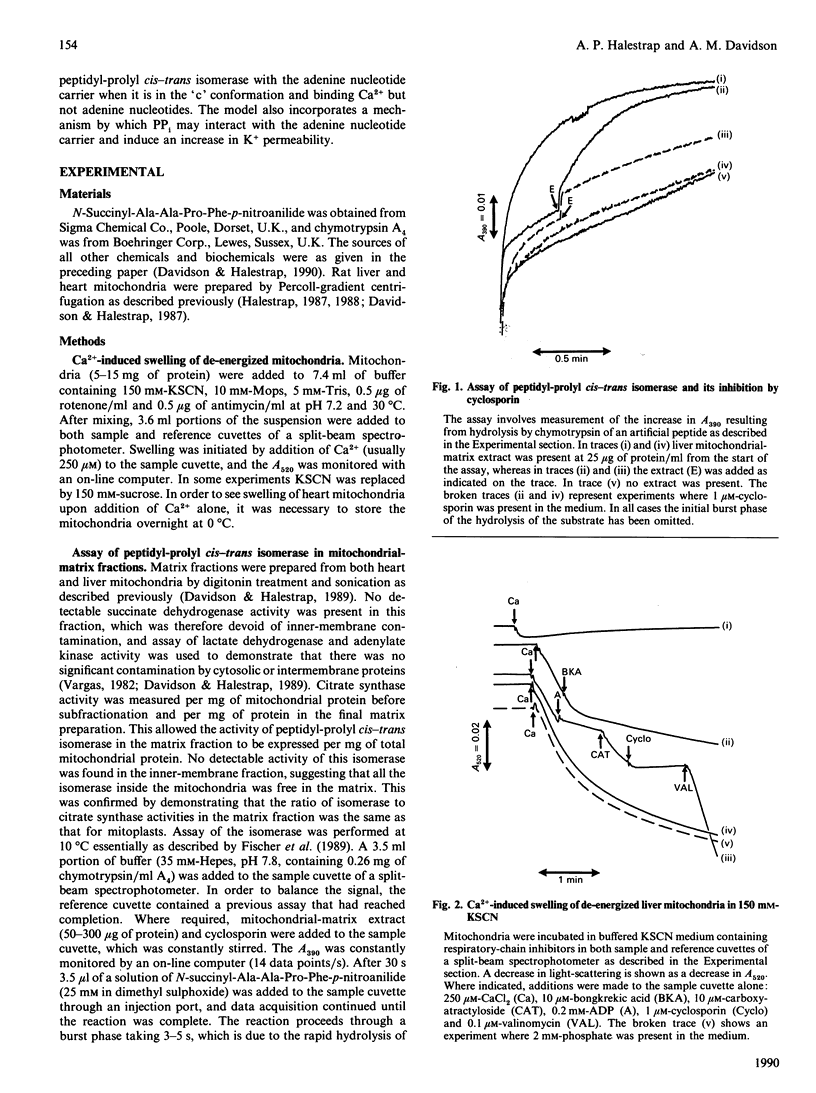
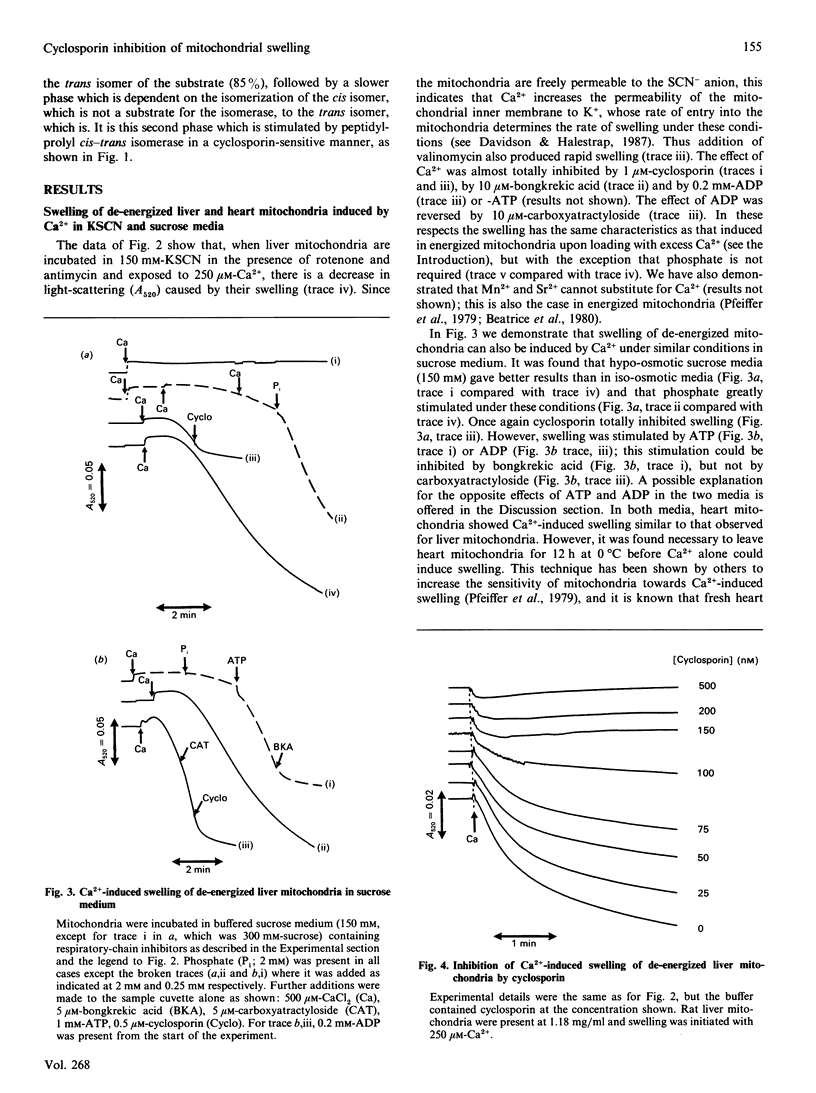
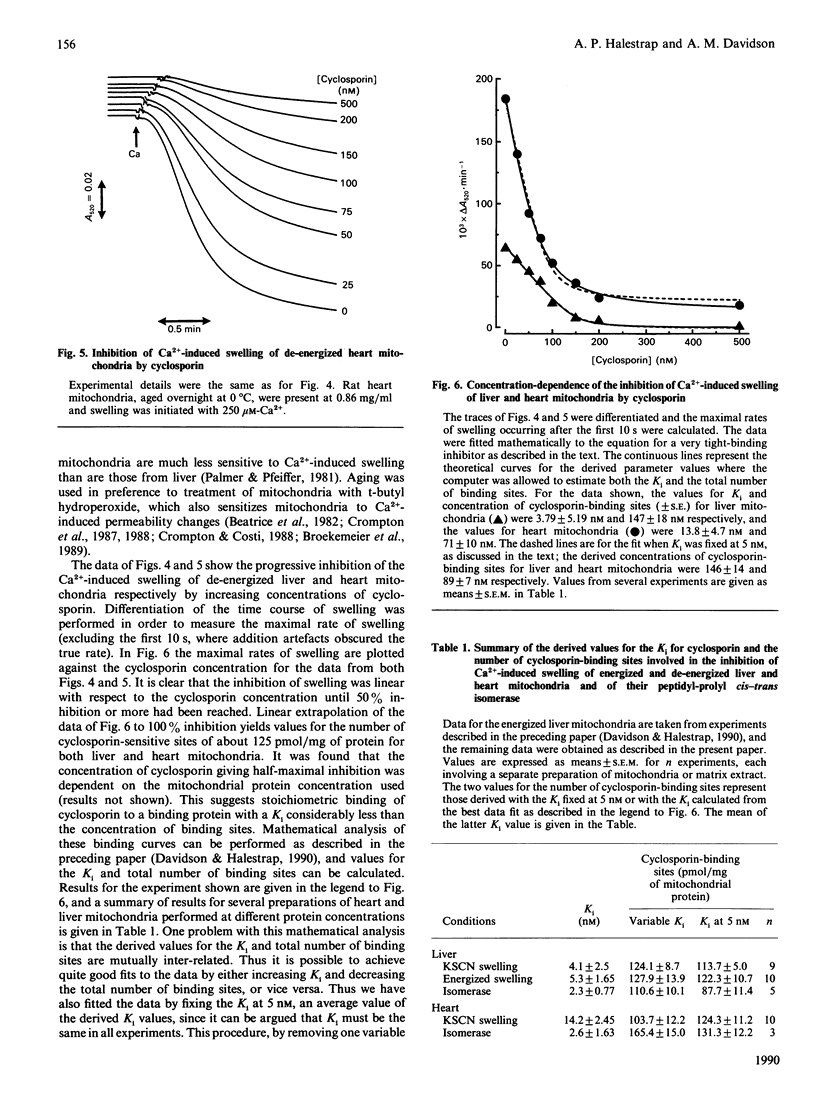
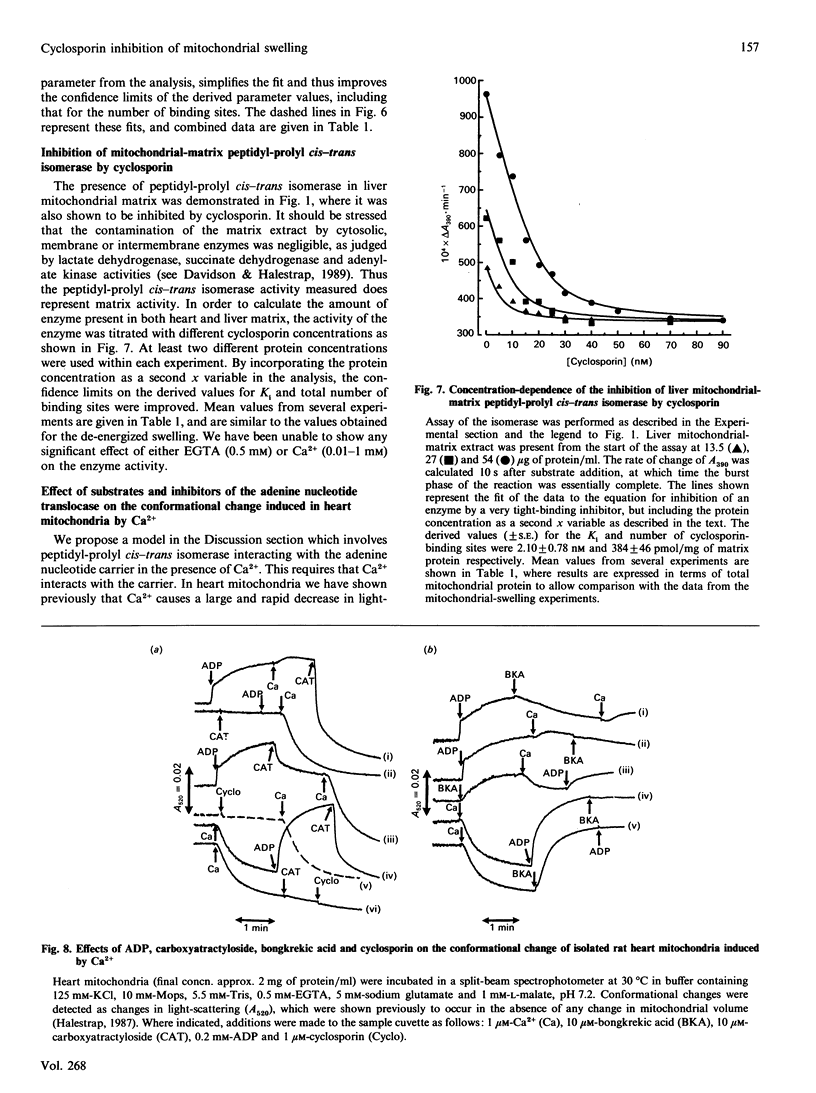
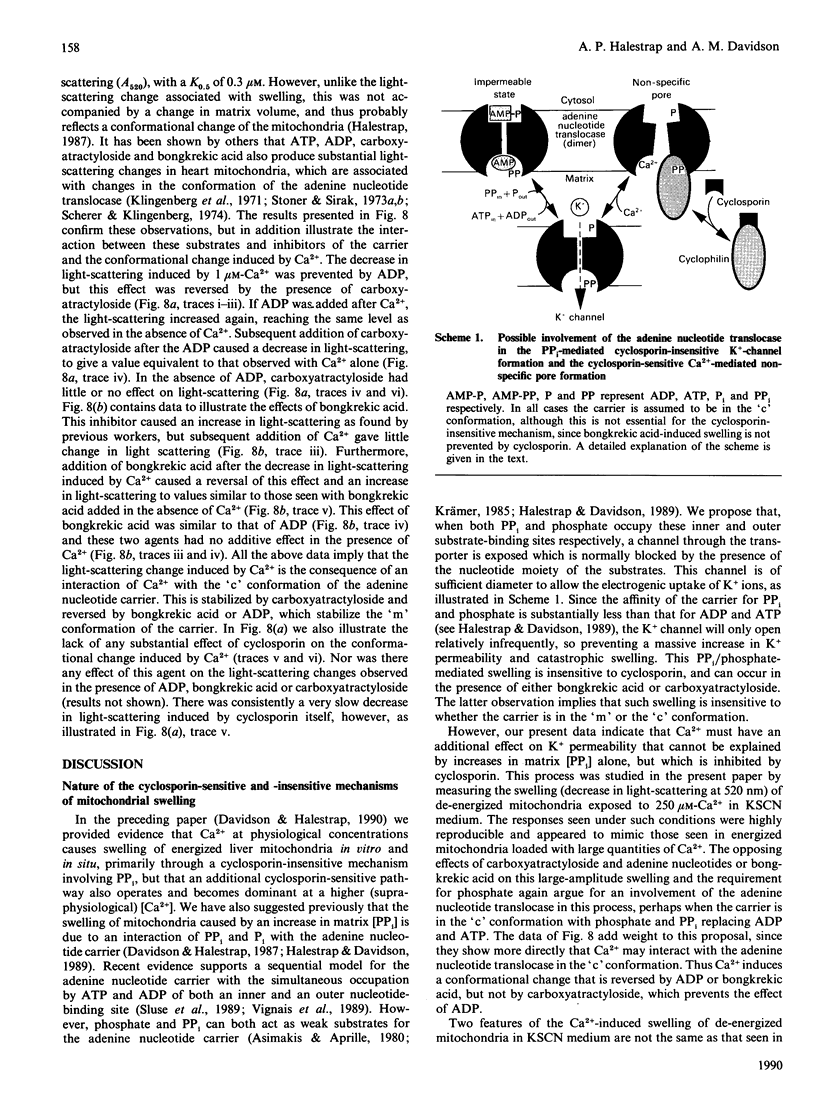
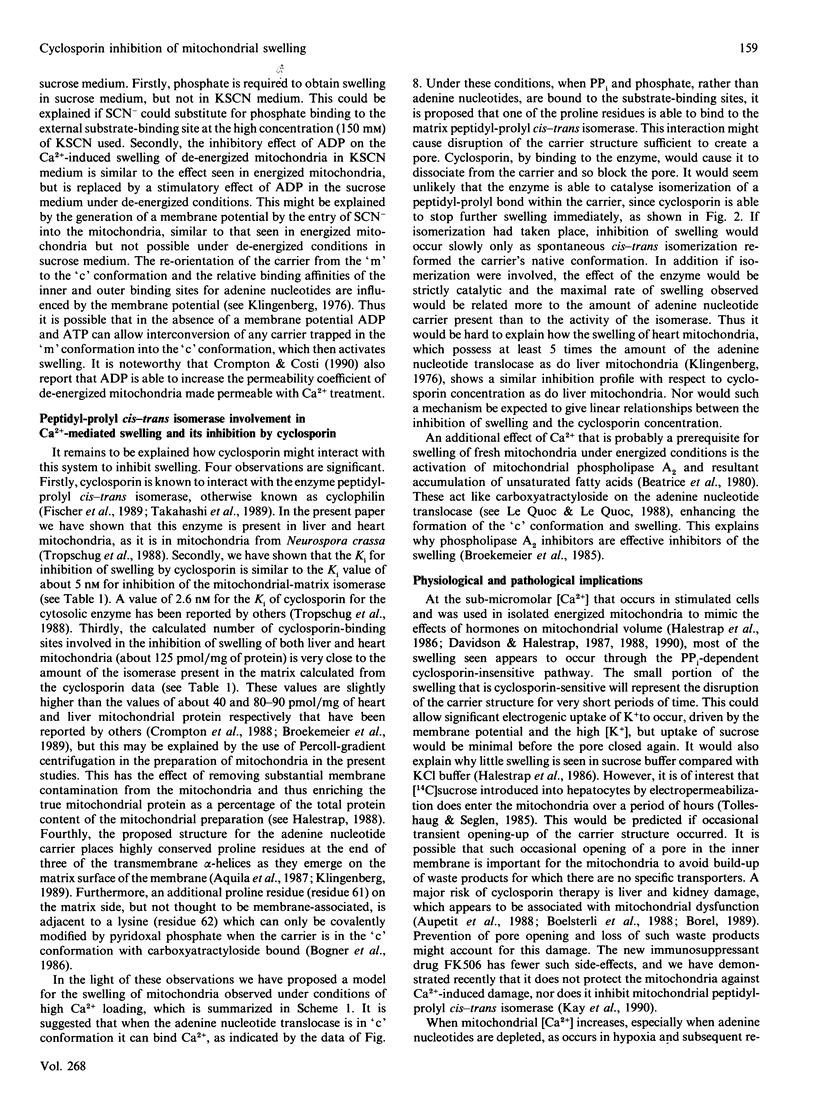
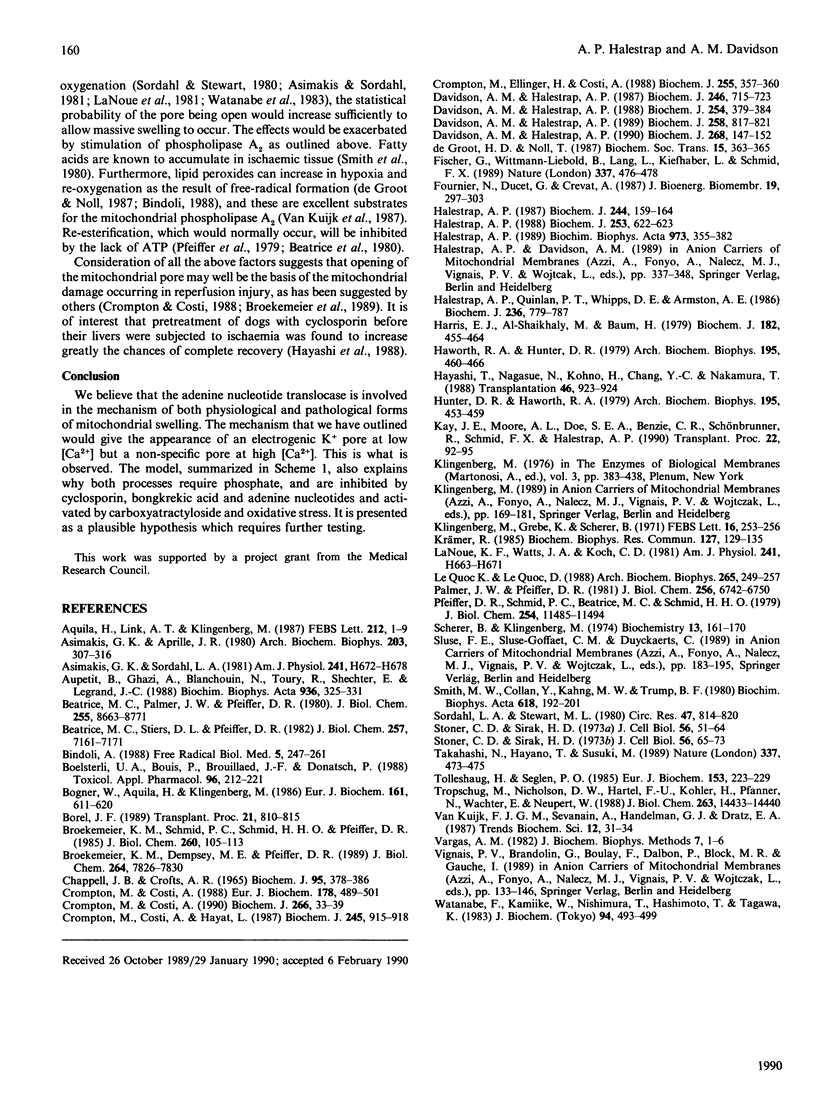
Images in this article
Selected References
These references are in PubMed. This may not be the complete list of references from this article.
- Aquila H., Link T. A., Klingenberg M. Solute carriers involved in energy transfer of mitochondria form a homologous protein family. FEBS Lett. 1987 Feb 9;212(1):1–9. doi: 10.1016/0014-5793(87)81546-6. [DOI] [PubMed] [Google Scholar]
- Asimakis G. K., Aprille J. R. In vitro alteration of the size of the liver mitochondrial adenine nucleotide pool: correlation with respiratory functions. Arch Biochem Biophys. 1980 Aug;203(1):307–316. doi: 10.1016/0003-9861(80)90181-2. [DOI] [PubMed] [Google Scholar]
- Asimakis G. K., Sordahl L. A. Intramitochondrial adenine nucleotides and energy-linked functions of heart mitochondria. Am J Physiol. 1981 Nov;241(5):H672–H678. doi: 10.1152/ajpheart.1981.241.5.H672. [DOI] [PubMed] [Google Scholar]
- Aupetit B., Ghazi A., Blanchouin N., Toury R., Shechter E., Legrand J. C. Impact on energy metabolism of quantitative and functional cyclosporine-induced damage of kidney mitochondria. Biochim Biophys Acta. 1988 Dec 7;936(3):325–331. doi: 10.1016/0005-2728(88)90008-4. [DOI] [PubMed] [Google Scholar]
- Beatrice M. C., Palmer J. W., Pfeiffer D. R. The relationship between mitochondrial membrane permeability, membrane potential, and the retention of Ca2+ by mitochondria. J Biol Chem. 1980 Sep 25;255(18):8663–8671. [PubMed] [Google Scholar]
- Beatrice M. C., Stiers D. L., Pfeiffer D. R. Increased permeability of mitochondria during Ca2+ release induced by t-butyl hydroperoxide or oxalacetate. the effect of ruthenium red. J Biol Chem. 1982 Jun 25;257(12):7161–7171. [PubMed] [Google Scholar]
- Bindoli A. Lipid peroxidation in mitochondria. Free Radic Biol Med. 1988;5(4):247–261. doi: 10.1016/0891-5849(88)90018-4. [DOI] [PubMed] [Google Scholar]
- Boelsterli U. A., Bouis P., Brouillard J. F., Donatsch P. In vitro toxicity assessment of cyclosporin A and its analogs in a primary rat hepatocyte culture model. Toxicol Appl Pharmacol. 1988 Nov;96(2):212–221. doi: 10.1016/0041-008x(88)90081-6. [DOI] [PubMed] [Google Scholar]
- Bogner W., Aquila H., Klingenberg M. The transmembrane arrangement of the ADP/ATP carrier as elucidated by the lysine reagent pyridoxal 5-phosphate. Eur J Biochem. 1986 Dec 15;161(3):611–620. doi: 10.1111/j.1432-1033.1986.tb10485.x. [DOI] [PubMed] [Google Scholar]
- Borel J. F. The cyclosporins. Transplant Proc. 1989 Feb;21(1 Pt 1):810–815. [PubMed] [Google Scholar]
- Broekemeier K. M., Dempsey M. E., Pfeiffer D. R. Cyclosporin A is a potent inhibitor of the inner membrane permeability transition in liver mitochondria. J Biol Chem. 1989 May 15;264(14):7826–7830. [PubMed] [Google Scholar]
- Broekemeier K. M., Schmid P. C., Schmid H. H., Pfeiffer D. R. Effects of phospholipase A2 inhibitors on ruthenium red-induced Ca2+ release from mitochondria. J Biol Chem. 1985 Jan 10;260(1):105–113. [PubMed] [Google Scholar]
- CHAPPELL J. B., CROFTS A. R. CALCIUM ION ACCUMULATION AND VOLUME CHANGES OF ISOLATED LIVER MITOCHONDRIA. CALCIUM ION-INDUCED SWELLING. Biochem J. 1965 May;95:378–386. doi: 10.1042/bj0950378. [DOI] [PMC free article] [PubMed] [Google Scholar]
- Crompton M., Costi A. A heart mitochondrial Ca2(+)-dependent pore of possible relevance to re-perfusion-induced injury. Evidence that ADP facilitates pore interconversion between the closed and open states. Biochem J. 1990 Feb 15;266(1):33–39. doi: 10.1042/bj2660033. [DOI] [PMC free article] [PubMed] [Google Scholar]
- Crompton M., Costi A., Hayat L. Evidence for the presence of a reversible Ca2+-dependent pore activated by oxidative stress in heart mitochondria. Biochem J. 1987 Aug 1;245(3):915–918. doi: 10.1042/bj2450915. [DOI] [PMC free article] [PubMed] [Google Scholar]
- Crompton M., Costi A. Kinetic evidence for a heart mitochondrial pore activated by Ca2+, inorganic phosphate and oxidative stress. A potential mechanism for mitochondrial dysfunction during cellular Ca2+ overload. Eur J Biochem. 1988 Dec 15;178(2):489–501. doi: 10.1111/j.1432-1033.1988.tb14475.x. [DOI] [PubMed] [Google Scholar]
- Crompton M., Ellinger H., Costi A. Inhibition by cyclosporin A of a Ca2+-dependent pore in heart mitochondria activated by inorganic phosphate and oxidative stress. Biochem J. 1988 Oct 1;255(1):357–360. [PMC free article] [PubMed] [Google Scholar]
- Davidson A. M., Halestrap A. P. Inhibition of mitochondrial-matrix inorganic pyrophosphatase by physiological [Ca2+], and its role in the hormonal regulation of mitochondrial matrix volume. Biochem J. 1989 Mar 15;258(3):817–821. doi: 10.1042/bj2580817. [DOI] [PMC free article] [PubMed] [Google Scholar]
- Davidson A. M., Halestrap A. P. Inorganic pyrophosphate is located primarily in the mitochondria of the hepatocyte and increases in parallel with the decrease in light-scattering induced by gluconeogenic hormones, butyrate and ionophore A23187. Biochem J. 1988 Sep 1;254(2):379–384. doi: 10.1042/bj2540379. [DOI] [PMC free article] [PubMed] [Google Scholar]
- Davidson A. M., Halestrap A. P. Liver mitochondrial pyrophosphate concentration is increased by Ca2+ and regulates the intramitochondrial volume and adenine nucleotide content. Biochem J. 1987 Sep 15;246(3):715–723. doi: 10.1042/bj2460715. [DOI] [PMC free article] [PubMed] [Google Scholar]
- Davidson A. M., Halestrap A. P. Partial inhibition by cyclosporin A of the swelling of liver mitochondria in vivo and in vitro induced by sub-micromolar [Ca2+], but not by butyrate. Evidence for two distinct swelling mechanisms. Biochem J. 1990 May 15;268(1):147–152. doi: 10.1042/bj2680147. [DOI] [PMC free article] [PubMed] [Google Scholar]
- Fischer G., Wittmann-Liebold B., Lang K., Kiefhaber T., Schmid F. X. Cyclophilin and peptidyl-prolyl cis-trans isomerase are probably identical proteins. Nature. 1989 Feb 2;337(6206):476–478. doi: 10.1038/337476a0. [DOI] [PubMed] [Google Scholar]
- Fournier N., Ducet G., Crevat A. Action of cyclosporine on mitochondrial calcium fluxes. J Bioenerg Biomembr. 1987 Jun;19(3):297–303. doi: 10.1007/BF00762419. [DOI] [PubMed] [Google Scholar]
- Halestrap A. P., Quinlan P. T., Whipps D. E., Armston A. E. Regulation of the mitochondrial matrix volume in vivo and in vitro. The role of calcium. Biochem J. 1986 Jun 15;236(3):779–787. doi: 10.1042/bj2360779. [DOI] [PMC free article] [PubMed] [Google Scholar]
- Halestrap A. P. The regulation of the matrix volume of mammalian mitochondria in vivo and in vitro and its role in the control of mitochondrial metabolism. Biochim Biophys Acta. 1989 Mar 23;973(3):355–382. doi: 10.1016/s0005-2728(89)80378-0. [DOI] [PubMed] [Google Scholar]
- Halestrap A. P. The regulation of the oxidation of fatty acids and other substrates in rat heart mitochondria by changes in the matrix volume induced by osmotic strength, valinomycin and Ca2+. Biochem J. 1987 May 15;244(1):159–164. doi: 10.1042/bj2440159. [DOI] [PMC free article] [PubMed] [Google Scholar]
- Harris E. J., Al-Shaikhaly M., Baum H. Stimulation of mitochondrial calcium ion efflux by thiol-specific reagents and by thyroxine. The relationship to adenosine diphosphate retention and to mitochondrial permeability. Biochem J. 1979 Aug 15;182(2):455–464. doi: 10.1042/bj1820455. [DOI] [PMC free article] [PubMed] [Google Scholar]
- Haworth R. A., Hunter D. R. The Ca2+-induced membrane transition in mitochondria. II. Nature of the Ca2+ trigger site. Arch Biochem Biophys. 1979 Jul;195(2):460–467. doi: 10.1016/0003-9861(79)90372-2. [DOI] [PubMed] [Google Scholar]
- Hayashi T., Nagasue N., Kohno H., Chang Y. C., Nakamura T. Beneficial effect of cyclosporine pretreatment in preventing ischemic damage to the liver in dogs. Transplantation. 1988 Dec;46(6):923–924. [PubMed] [Google Scholar]
- Hunter D. R., Haworth R. A. The Ca2+-induced membrane transition in mitochondria. I. The protective mechanisms. Arch Biochem Biophys. 1979 Jul;195(2):453–459. doi: 10.1016/0003-9861(79)90371-0. [DOI] [PubMed] [Google Scholar]
- Klingenberg M., Grebe K., Scherer B. Opposite effects of bongkrekic acid and atractyloside on the adenine nucleotides induced mitochondrial volume changes and on the efflux of adenine nucleotides. FEBS Lett. 1971 Sep 1;16(4):253–256. doi: 10.1016/0014-5793(71)80363-0. [DOI] [PubMed] [Google Scholar]
- Krämer R. Characterization of pyrophosphate exchange by the reconstituted adenine nucleotide translocator from mitochondria. Biochem Biophys Res Commun. 1985 Feb 28;127(1):129–135. doi: 10.1016/s0006-291x(85)80135-2. [DOI] [PubMed] [Google Scholar]
- LaNoue K. F., Watts J. A., Koch C. D. Adenine nucleotide transport during cardiac ischemia. Am J Physiol. 1981 Nov;241(5):H663–H671. doi: 10.1152/ajpheart.1981.241.5.H663. [DOI] [PubMed] [Google Scholar]
- Lê Quôc K., Lê Quôc D. Involvement of the ADP/ATP carrier in calcium-induced perturbations of the mitochondrial inner membrane permeability: importance of the orientation of the nucleotide binding site. Arch Biochem Biophys. 1988 Sep;265(2):249–257. doi: 10.1016/0003-9861(88)90125-7. [DOI] [PubMed] [Google Scholar]
- Palmer J. W., Pfeiffer D. R. The control of Ca2+ release from heart mitochondria. J Biol Chem. 1981 Jul 10;256(13):6742–6750. [PubMed] [Google Scholar]
- Pfeiffer D. R., Schmid P. C., Beatrice M. C., Schmid H. H. Intramitochondrial phospholipase activity and the effects of Ca2+ plus N-ethylmaleimide on mitochondrial function. J Biol Chem. 1979 Nov 25;254(22):11485–11494. [PubMed] [Google Scholar]
- Scherer B., Klingenberg M. Demonstration of the relationship between the adenine nucleotide carrier and the structural changes of mitochondria as induced by adenosine 5'-diphosphate. Biochemistry. 1974 Jan 1;13(1):161–170. doi: 10.1021/bi00698a025. [DOI] [PubMed] [Google Scholar]
- Schratter M., Kramer J., Prayer L., Wimberger D., Schmid W., Imhof H. Linear versus zirkular polarisierte Kopfspule--Vergleich am Phantom und in der Klinik. Rofo. 1990 Jul;153(1):92–95. doi: 10.1055/s-2008-1033339. [DOI] [PubMed] [Google Scholar]
- Smith M. W., Collan Y., Kahng M. W., Trump B. F. Changes in mitochondrial lipids of rat kidney during ischemia. Biochim Biophys Acta. 1980 May 28;618(2):192–201. doi: 10.1016/0005-2760(80)90025-9. [DOI] [PubMed] [Google Scholar]
- Sordahl L. A., Stewart M. L. Mechanism(s) of altered mitochondrial calcium transport in acutely ischemic canine hearts. Circ Res. 1980 Dec;47(6):814–820. doi: 10.1161/01.res.47.6.814. [DOI] [PubMed] [Google Scholar]
- Stoner C. D., Sirak H. D. Adenine nucleotide-induced contraction of the inner mitochondrial membrane. I. General characterization. J Cell Biol. 1973 Jan;56(1):51–64. doi: 10.1083/jcb.56.1.51. [DOI] [PMC free article] [PubMed] [Google Scholar]
- Stoner C. D., Sirak H. D. Adenine nucleotide-induced contraction on the inner mitochondrial membrane. II. Effect of bongkrekic acid. J Cell Biol. 1973 Jan;56(1):65–73. doi: 10.1083/jcb.56.1.65. [DOI] [PMC free article] [PubMed] [Google Scholar]
- Takahashi N., Hayano T., Suzuki M. Peptidyl-prolyl cis-trans isomerase is the cyclosporin A-binding protein cyclophilin. Nature. 1989 Feb 2;337(6206):473–475. doi: 10.1038/337473a0. [DOI] [PubMed] [Google Scholar]
- Tolleshaug H., Seglen P. O. Autophagic-lysosomal and mitochondrial sequestration of [14C]sucrose. Density gradient distribution of sequestered radioactivity. Eur J Biochem. 1985 Dec 2;153(2):223–229. doi: 10.1111/j.1432-1033.1985.tb09290.x. [DOI] [PubMed] [Google Scholar]
- Tropschug M., Nicholson D. W., Hartl F. U., Köhler H., Pfanner N., Wachter E., Neupert W. Cyclosporin A-binding protein (cyclophilin) of Neurospora crassa. One gene codes for both the cytosolic and mitochondrial forms. J Biol Chem. 1988 Oct 5;263(28):14433–14440. [PubMed] [Google Scholar]
- Vargas A. M. Rapid preparation of metabolically active mitochondria from control and hormone-treated rat liver cells. J Biochem Biophys Methods. 1982 Dec;7(1):1–6. doi: 10.1016/0165-022x(82)90030-6. [DOI] [PubMed] [Google Scholar]
- Watanabe F., Kamiike W., Nishimura T., Hashimoto T., Tagawa K. Decrease in mitochondrial levels of adenine nucleotides and concomitant mitochondrial dysfunction in ischemic rat liver. J Biochem. 1983 Aug;94(2):493–499. doi: 10.1093/oxfordjournals.jbchem.a134380. [DOI] [PubMed] [Google Scholar]
- de Groot H., Noll T. Oxygen gradients: the problem of hypoxia. Biochem Soc Trans. 1987 Jun;15(3):363–365. doi: 10.1042/bst0150363. [DOI] [PubMed] [Google Scholar]