Abstract
The pattern and kinetics of partial proteolysis of Arthrobacter D-xylose isomerase tetramer was studied in order to determine the flexibility of surface loops that may control its stability. It was completely resistant to trypsin, chymotrypsin and elastase at 37 degrees C, but thermolysin cleaved specifically and quantitatively at Thr-347-Leu-348 between helices 10 and 11 to remove 47 residues from the C-terminus of each 43.3 kDa subunit. At high temperatures, helices 9 and 10 were removed from each 38 kDa subunit to give a 36 kDa tetramer. The kinetics of nicking by thermolysin indicated that the Thr-347-Leu-348 loop is locked at low temperatures, but 'melts' at 25 degrees C and is fully flexible above 34 degrees C. The flexibility appears to be associated with binding of Ca2+ ions at the active site, since Co2+, Mg2+ and xylitol protect in proportion to their ability to displace Ca2+. The missing C-terminal helices make many intersubunit contacts that appear in the structure to stabilize the tetramer, but the properties of the purified nicked proteins are almost indistinguishable from the native enzyme. Both the 38 kDa tetramer and the 36 kDa tetramer are identically active and dissociate similarly in urea or SDS to fully active dimers, but the nicked dimers are slightly less stable to urea at 62 degrees C. In the Mg2+ form the thermostability of the 38 kDa tetramer is identical with that of the native enzyme, but the 36 kDa tetramer has a slightly lower 'melting point' (70 degrees C versus 80 degrees C), which may be due to unravelling from the end of helix 8. Since elimination of all the C-terminal helices and many intersubunit contacts has so little effect, one can conclude that the 'weak point' that controls the protein's thermostability lies within the N-terminal beta-barrel domain.
Full text
PDF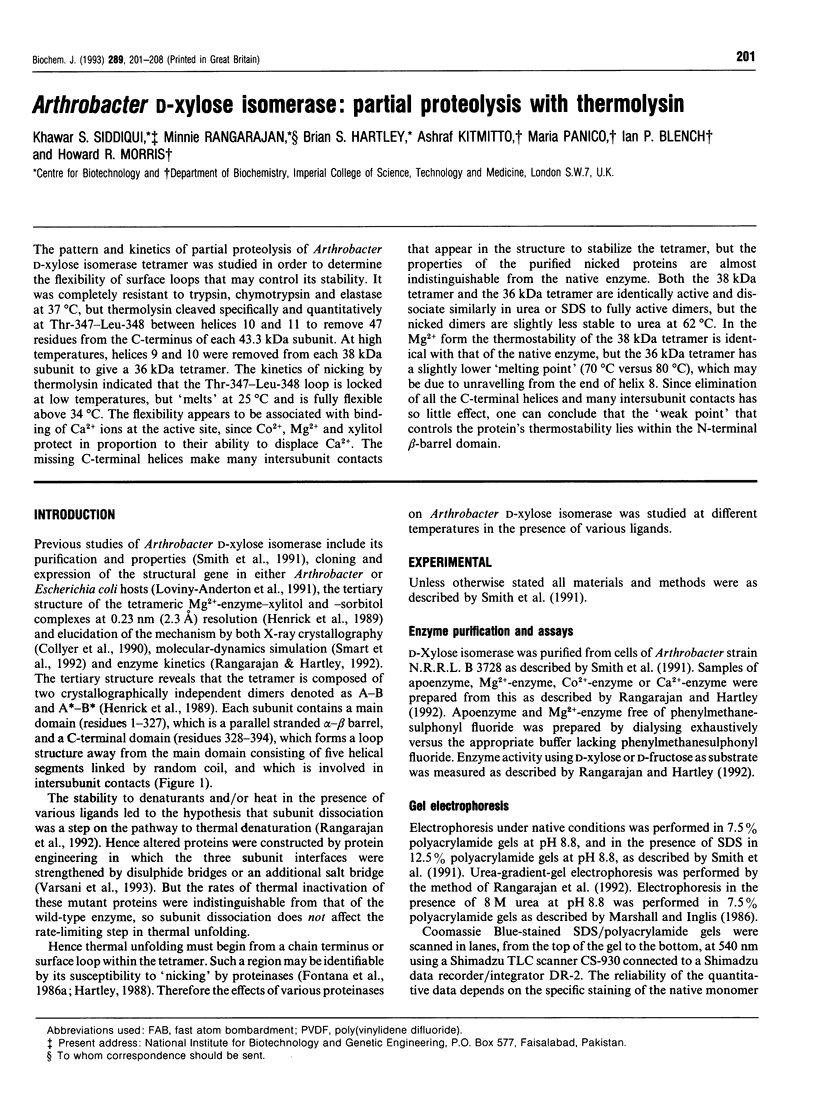
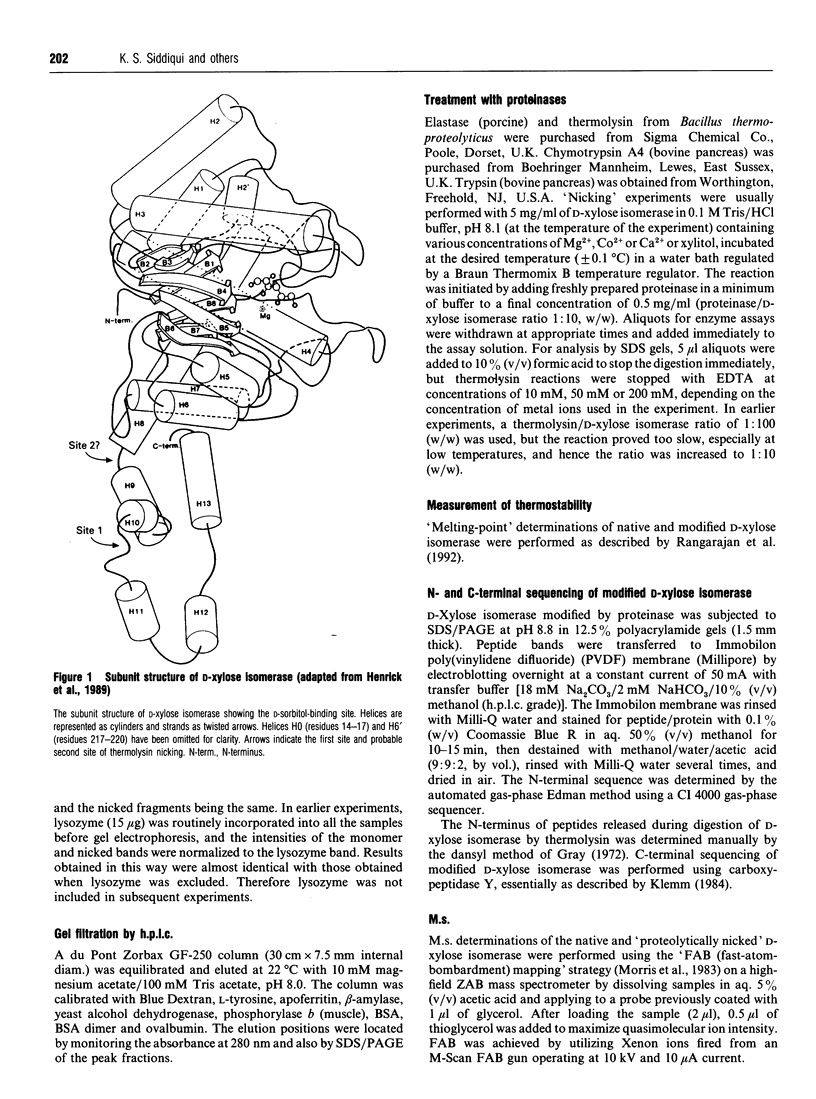
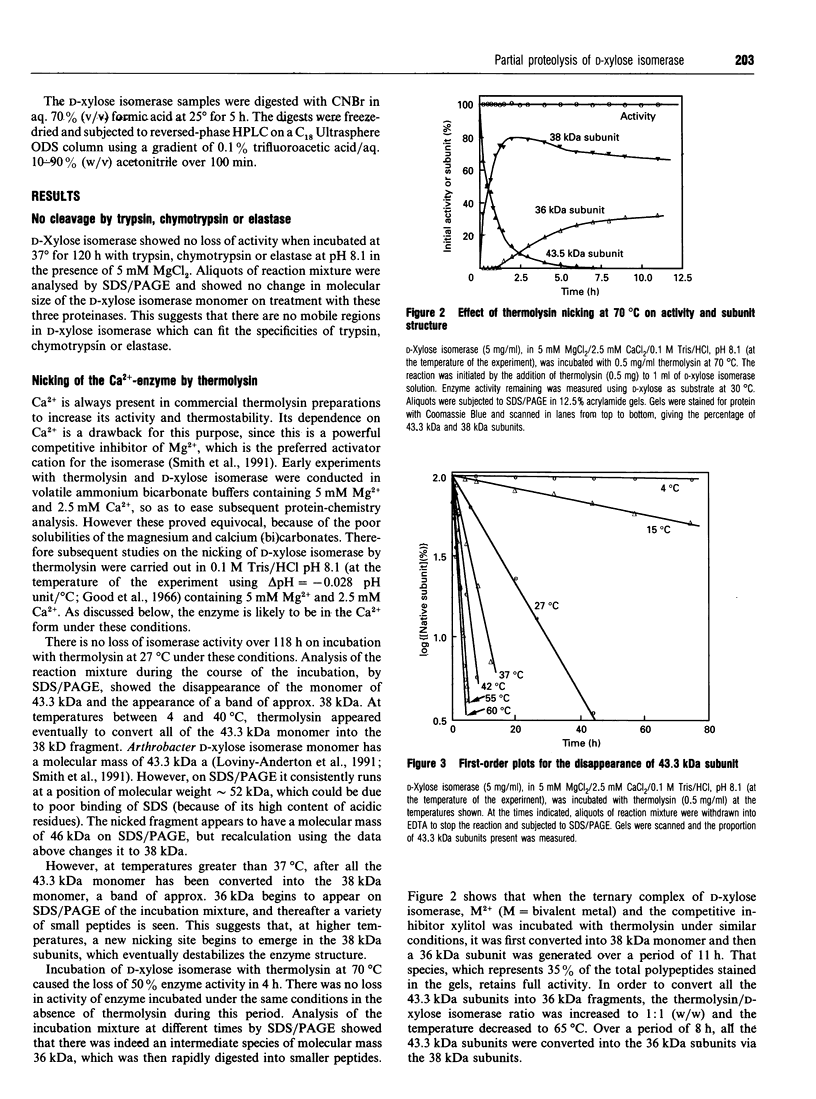
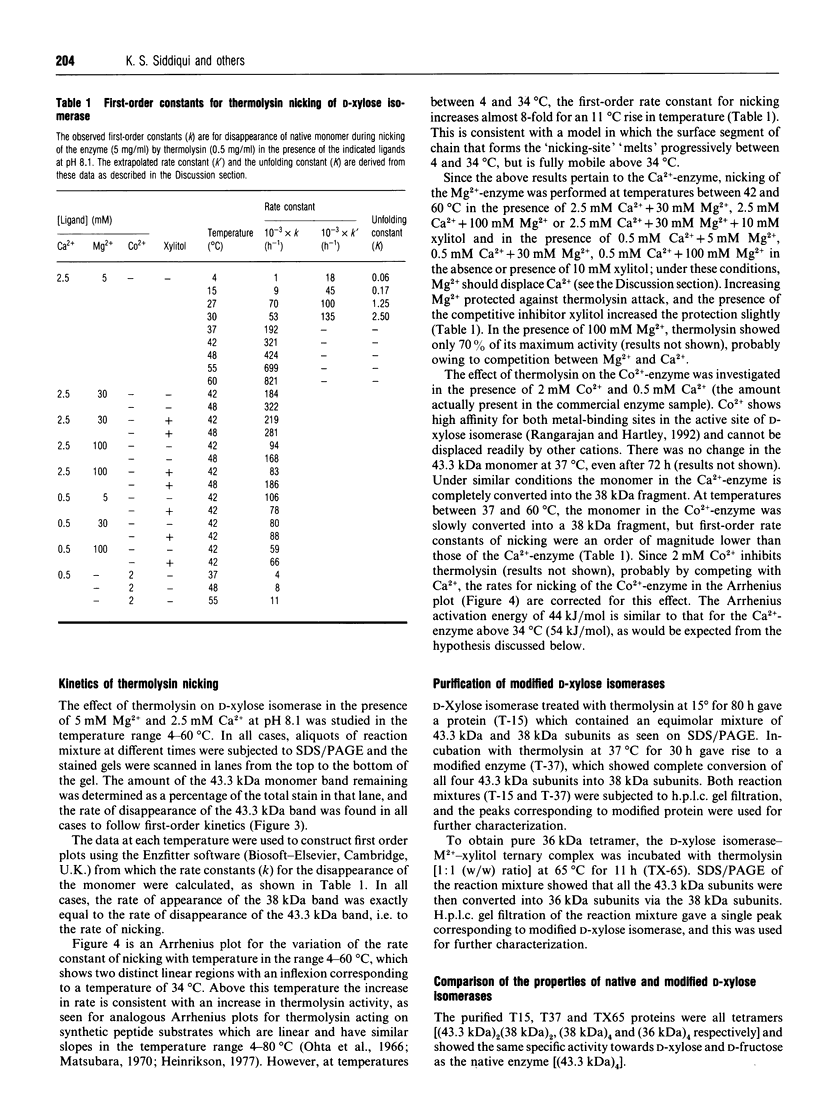
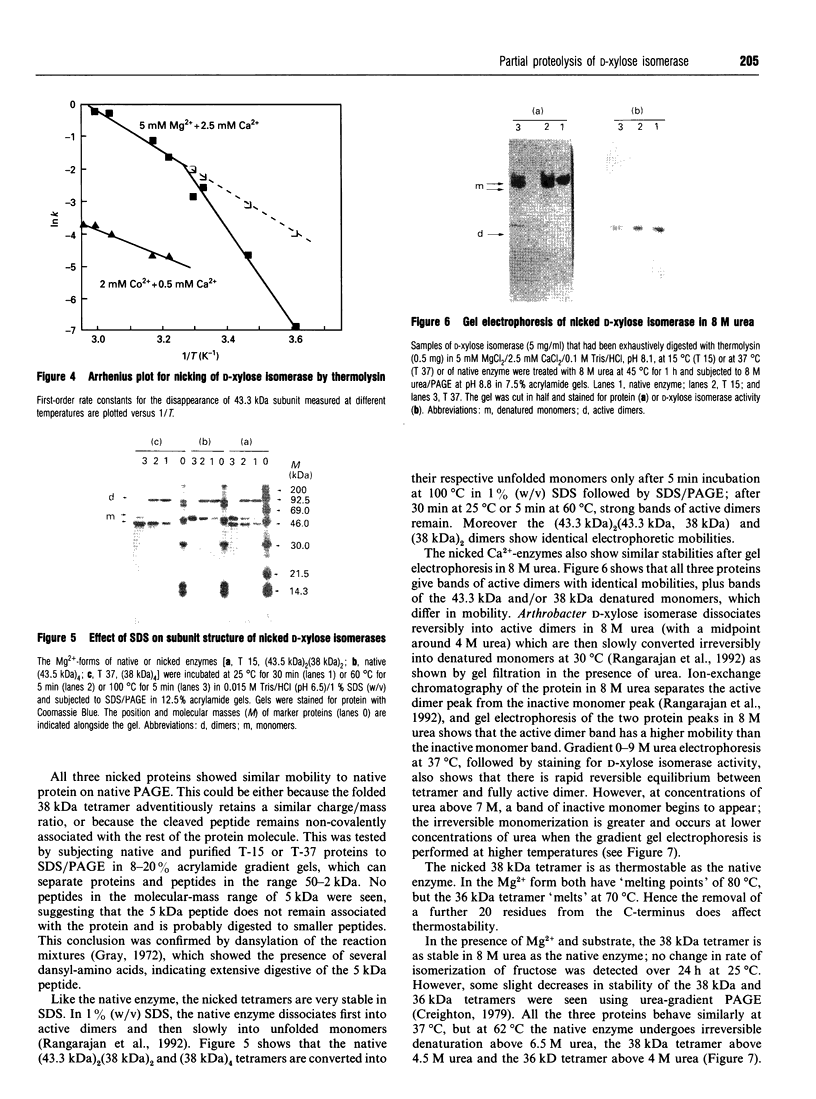
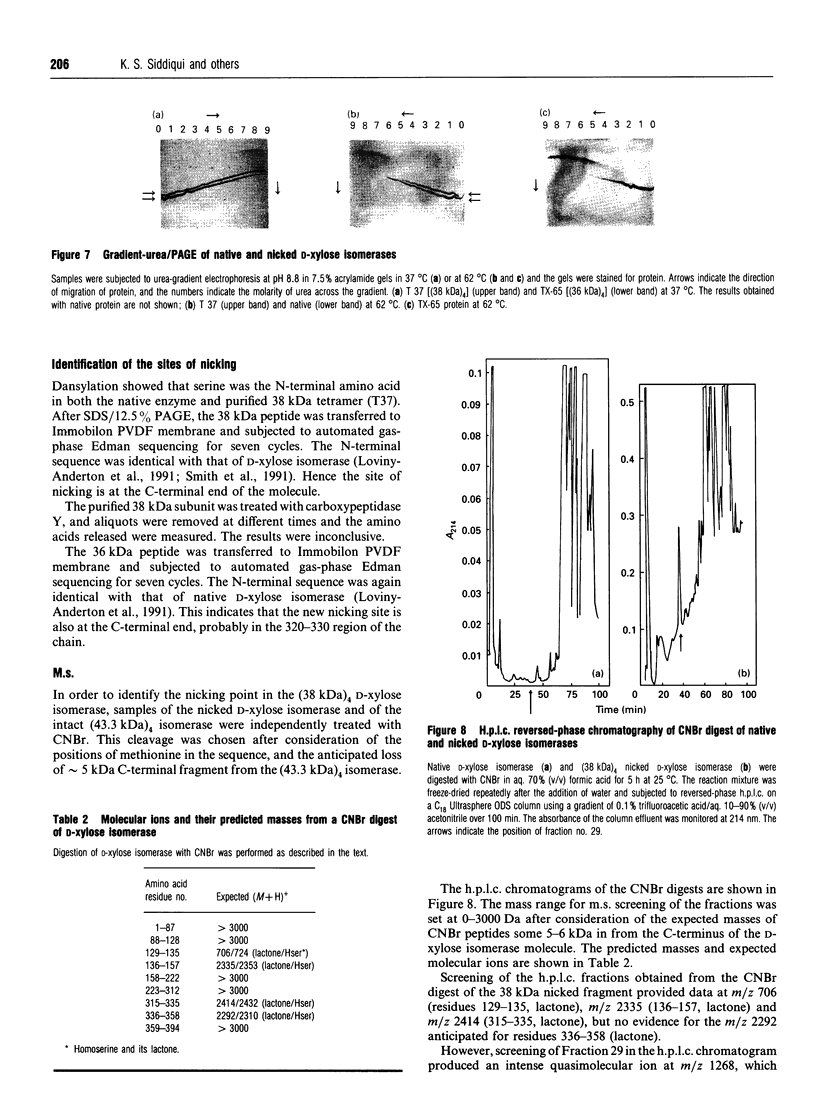
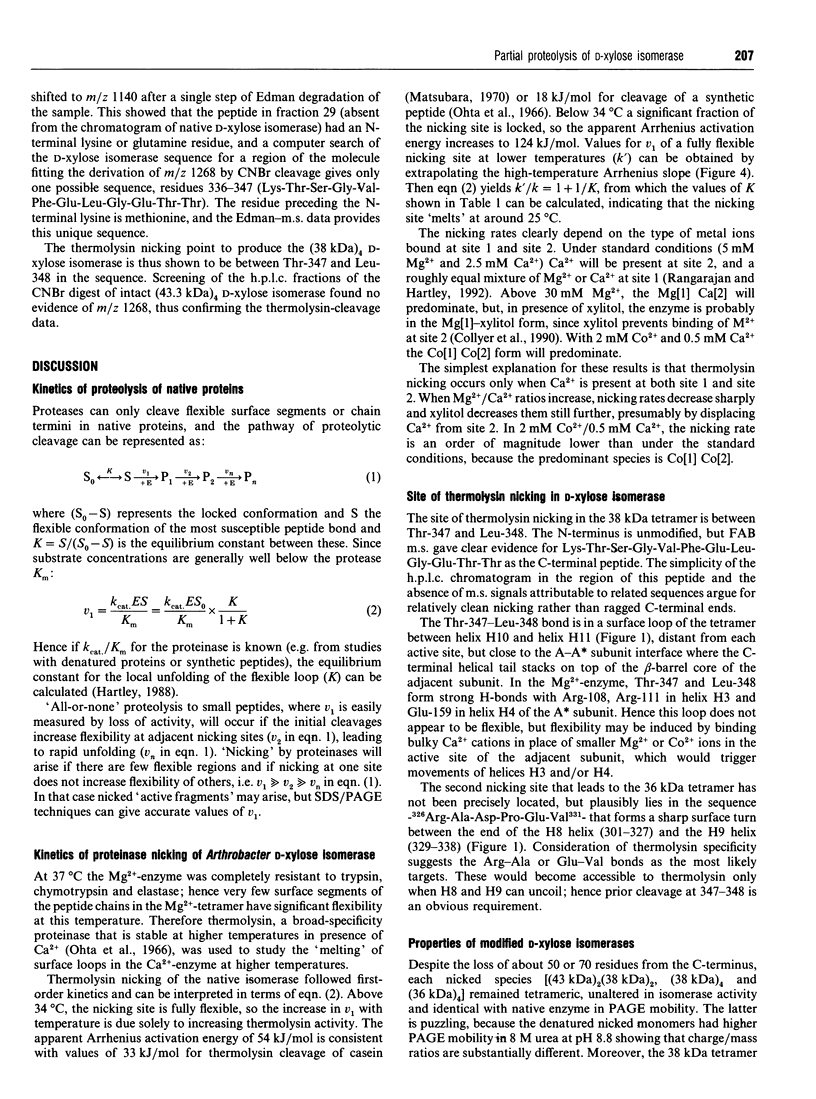
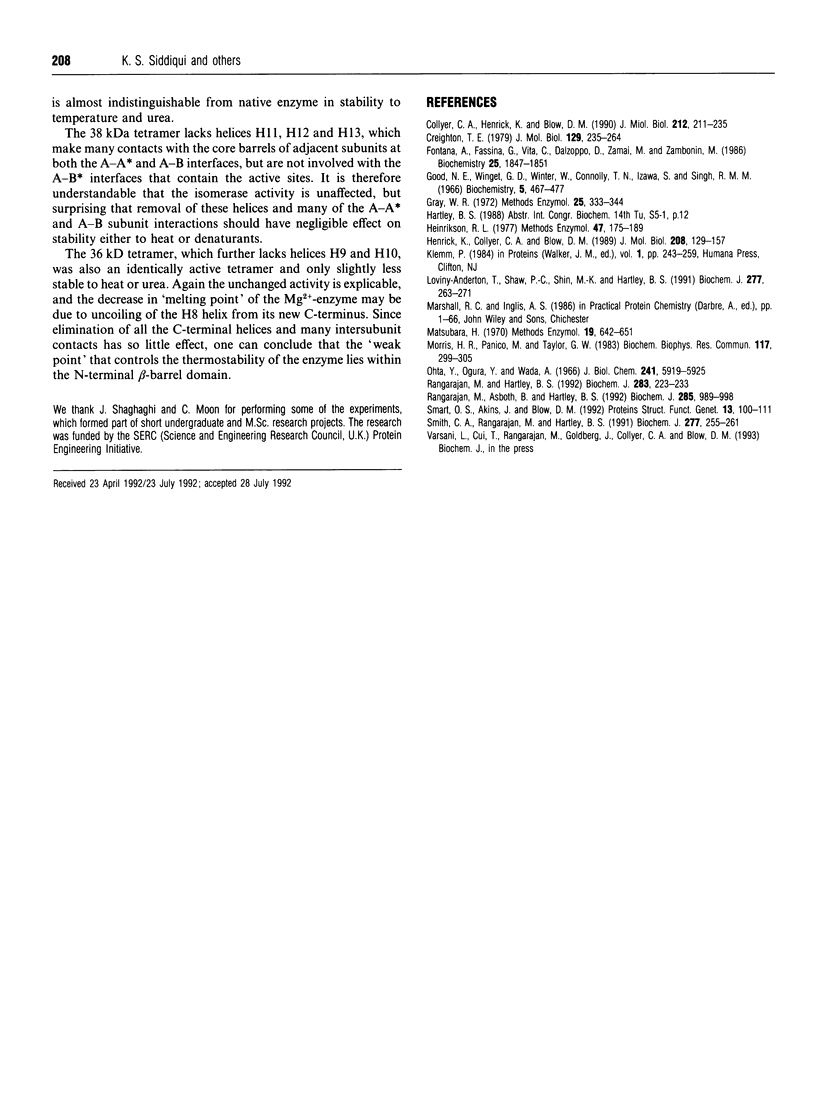
Images in this article
Selected References
These references are in PubMed. This may not be the complete list of references from this article.
- Collyer C. A., Henrick K., Blow D. M. Mechanism for aldose-ketose interconversion by D-xylose isomerase involving ring opening followed by a 1,2-hydride shift. J Mol Biol. 1990 Mar 5;212(1):211–235. doi: 10.1016/0022-2836(90)90316-E. [DOI] [PubMed] [Google Scholar]
- Creighton T. E. Electrophoretic analysis of the unfolding of proteins by urea. J Mol Biol. 1979 Apr 5;129(2):235–264. doi: 10.1016/0022-2836(79)90279-1. [DOI] [PubMed] [Google Scholar]
- Fontana A., Fassina G., Vita C., Dalzoppo D., Zamai M., Zambonin M. Correlation between sites of limited proteolysis and segmental mobility in thermolysin. Biochemistry. 1986 Apr 22;25(8):1847–1851. doi: 10.1021/bi00356a001. [DOI] [PubMed] [Google Scholar]
- Good N. E., Winget G. D., Winter W., Connolly T. N., Izawa S., Singh R. M. Hydrogen ion buffers for biological research. Biochemistry. 1966 Feb;5(2):467–477. doi: 10.1021/bi00866a011. [DOI] [PubMed] [Google Scholar]
- Heinrikson R. L. Applications of thermolysin in protein structural analysis. Methods Enzymol. 1977;47:175–189. doi: 10.1016/0076-6879(77)47022-8. [DOI] [PubMed] [Google Scholar]
- Henrick K., Collyer C. A., Blow D. M. Structures of D-xylose isomerase from Arthrobacter strain B3728 containing the inhibitors xylitol and D-sorbitol at 2.5 A and 2.3 A resolution, respectively. J Mol Biol. 1989 Jul 5;208(1):129–157. doi: 10.1016/0022-2836(89)90092-2. [DOI] [PubMed] [Google Scholar]
- Loviny-Anderton T., Shaw P. C., Shin M. K., Hartley B. S. D-Xylose (D-glucose) isomerase from Arthrobacter strain N.R.R.L. B3728. Gene cloning, sequence and expression. Biochem J. 1991 Jul 1;277(Pt 1):263–271. doi: 10.1042/bj2770263. [DOI] [PMC free article] [PubMed] [Google Scholar]
- Morris H. R., Panico M., Taylor G. W. FAB-mapping of recombinant-DNA protein products. Biochem Biophys Res Commun. 1983 Nov 30;117(1):299–305. doi: 10.1016/0006-291x(83)91575-9. [DOI] [PubMed] [Google Scholar]
- Ohta Y., Ogura Y., Wada A. Thermostable protease from thermophilic bacteria. I. Thermostability, physiocochemical properties, and amino acid composition. J Biol Chem. 1966 Dec 25;241(24):5919–5925. [PubMed] [Google Scholar]
- Rangarajan M., Hartley B. S. Mechanism of D-fructose isomerization by Arthrobacter D-xylose isomerase. Biochem J. 1992 Apr 1;283(Pt 1):223–233. doi: 10.1042/bj2830223. [DOI] [PMC free article] [PubMed] [Google Scholar]
- Smart O. S., Akins J., Blow D. M. Molecular mechanics simulations of a conformational rearrangement of D-xylose in the active site of D-xylose isomerase. Proteins. 1992 Apr;13(2):100–111. doi: 10.1002/prot.340130203. [DOI] [PubMed] [Google Scholar]
- Smith C. A., Rangarajan M., Hartley B. S. D-Xylose (D-glucose) isomerase from Arthrobacter strain N.R.R.L. B3728. Purification and properties. Biochem J. 1991 Jul 1;277(Pt 1):255–261. doi: 10.1042/bj2770255. [DOI] [PMC free article] [PubMed] [Google Scholar]