Abstract
1. The ability of myo-inositol polyphosphates to inhibit iron-catalysed hydroxyl radical formation was studied in a hypoxanthine/xanthine oxidase system [Graf, Empson and Eaton (1987) J. Biol. Chem. 262, 11647-11650]. Fe3+ present in the assay reagents supported some radical formation, and a standard assay, with 5 microM Fe3+ added, was used to investigate the specificity of compounds which could inhibit radical generation. 2. InsP6 (phytic acid) was able to inhibit radical formation in this assay completely. In this respect it was similar to the effects of the high affinity Fe3+ chelator Desferral, and dissimilar to the effects of EDTA which, even at high concentrations, still allowed detectable radical formation to take place. 3. The six isomers of InsP5 were purified from an alkaline hydrolysate of InsP6 (four of them as two enantiomeric mixtures), and they were compared with InsP6 in this assay. Ins(1,2,3,4,6)P5 and D/L-Ins(1,2,3,4,5)P5 were similar to InsP6 in that they caused a complete inhibition of iron-catalysed radical formation at > 30 microM. Ins(1,3,4,5,6)P5 and D/L-Ins(1,2,4,5,6)P5, however, were markedly less potent than InsP6, and did not inhibit radical formation completely; even when Ins(1,3,4,5,6)P5 was added up to 600 microM, significant radical formation was still detected. Thus InsP5s lacking 2 or 1/3 phosphates are in this respect qualitatively different from InsP6 and the other InsP5s. 4. scyllo-Inositol hexakisphosphate was also tested, and although it caused a greater inhibition than Ins(1,3,4,5,6)P5, it too still allowed detectable free radical formation even at 600 microM. 5. We conclude that the 1,2,3 (equatorial-axial-equatorial) phosphate grouping in InsP6 has a conformation that uniquely provides a specific interaction with iron to inhibit totally its ability to catalyse hydroxyl radical formation; we suggest that a physiological function of InsP6 might be to act as a 'safe' binding site for iron during its transport through the cytosol or cellular organelles.
Full text
PDF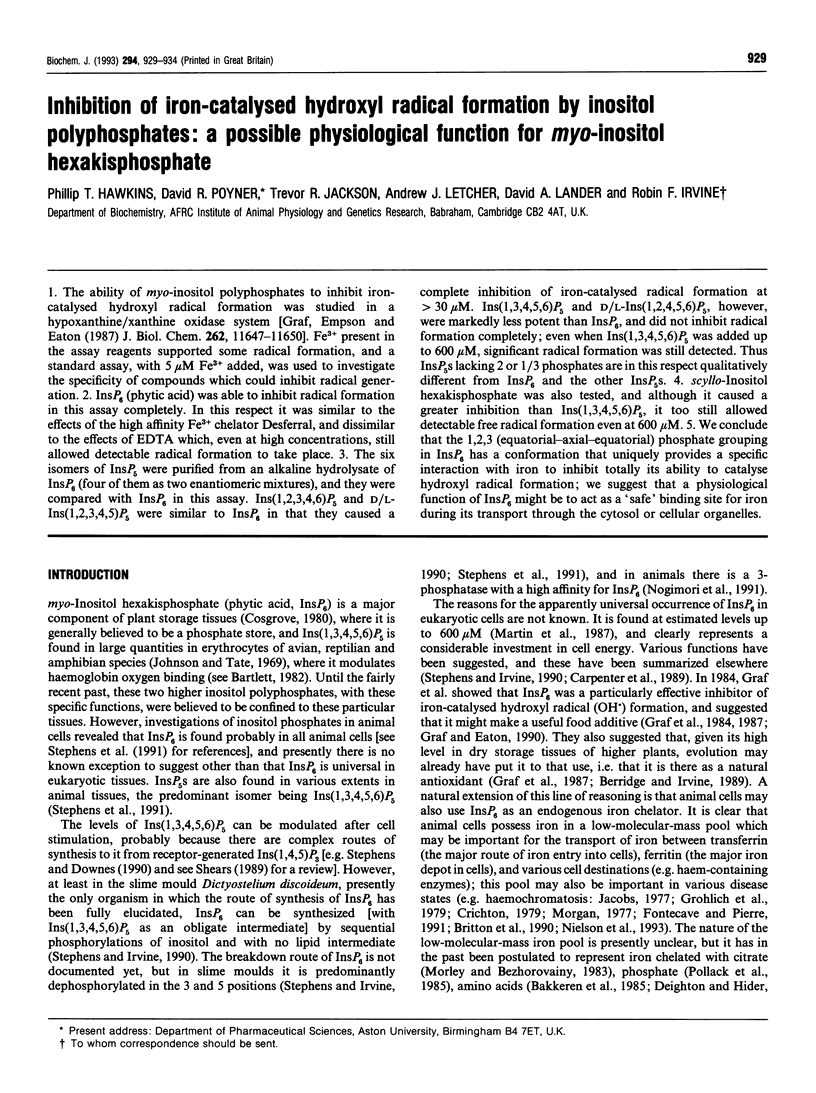
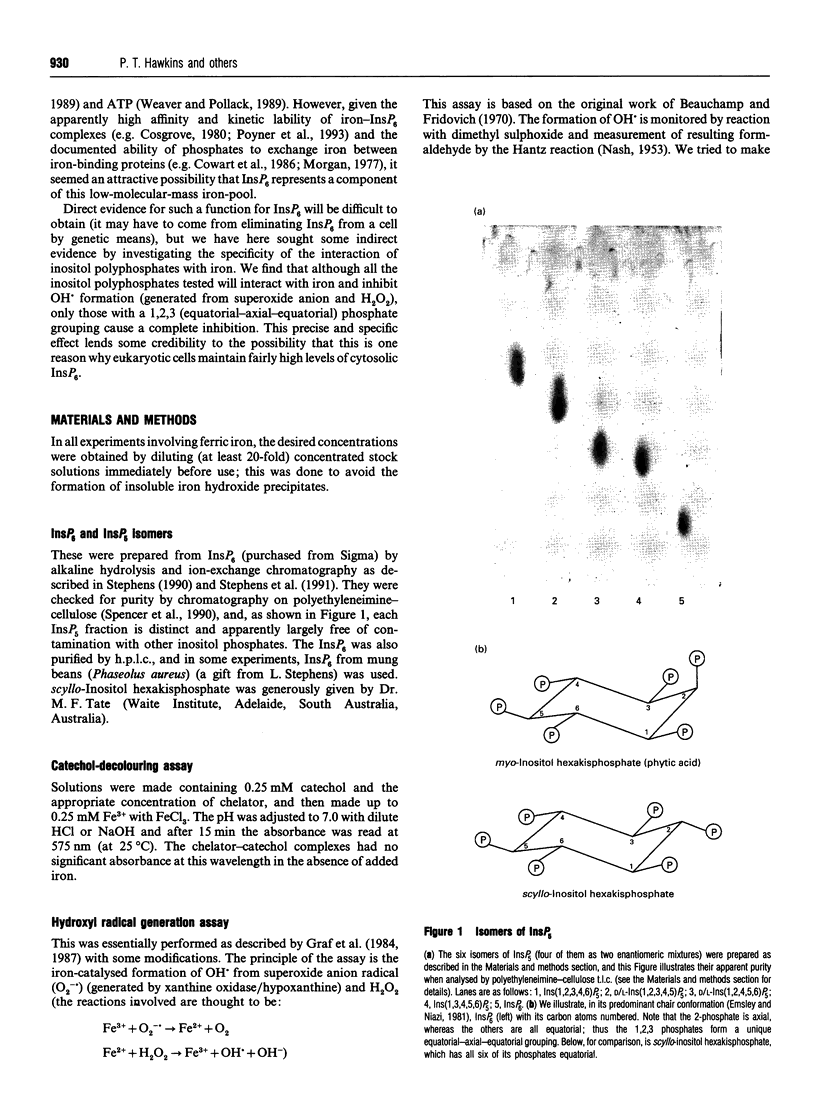
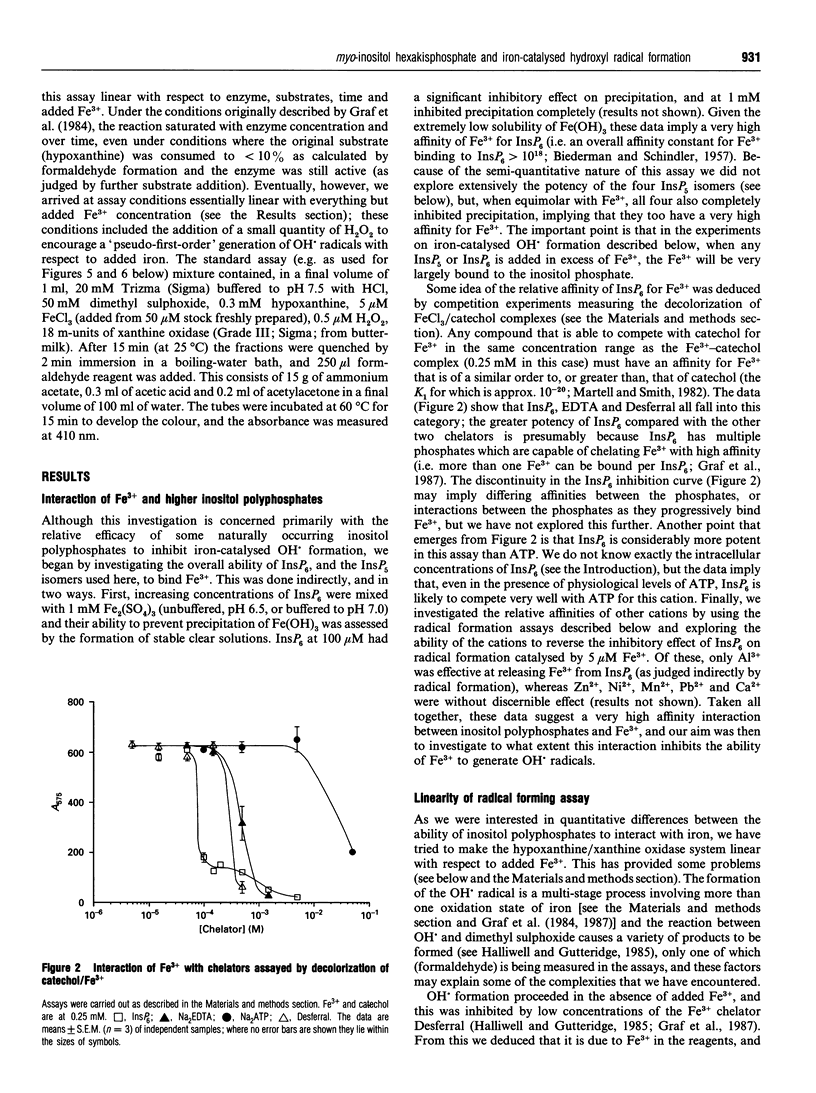
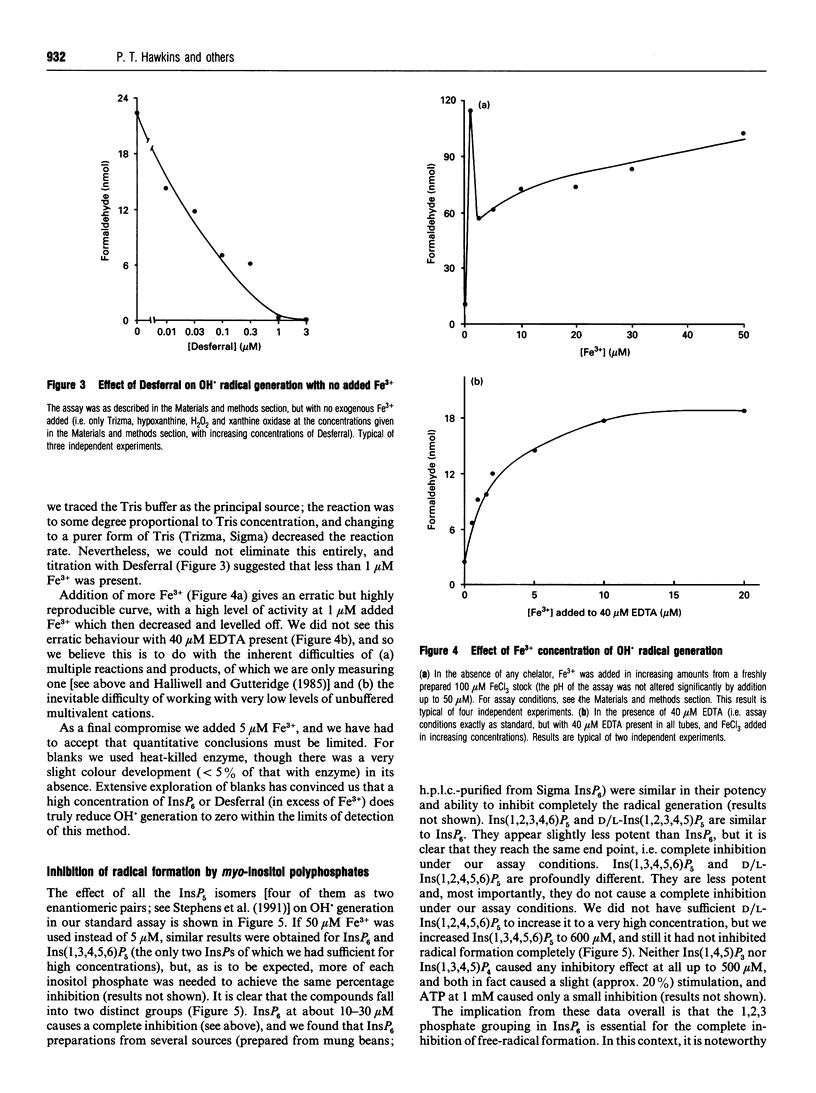
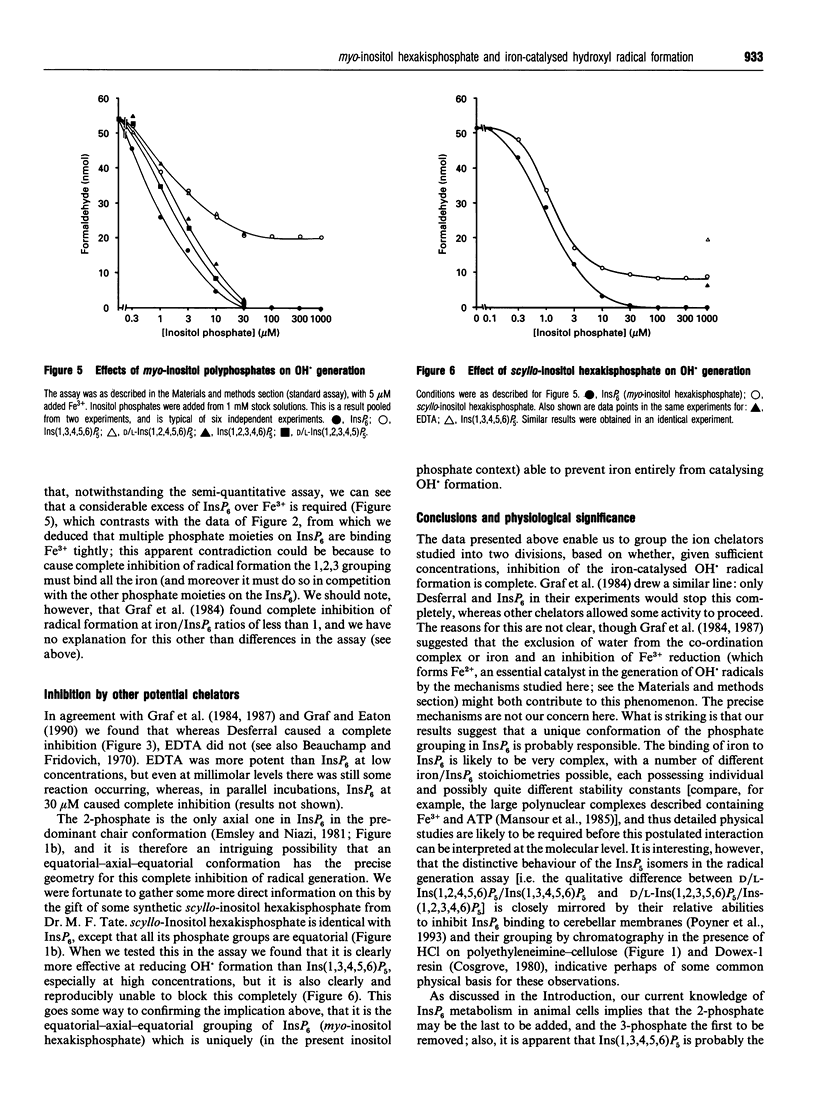
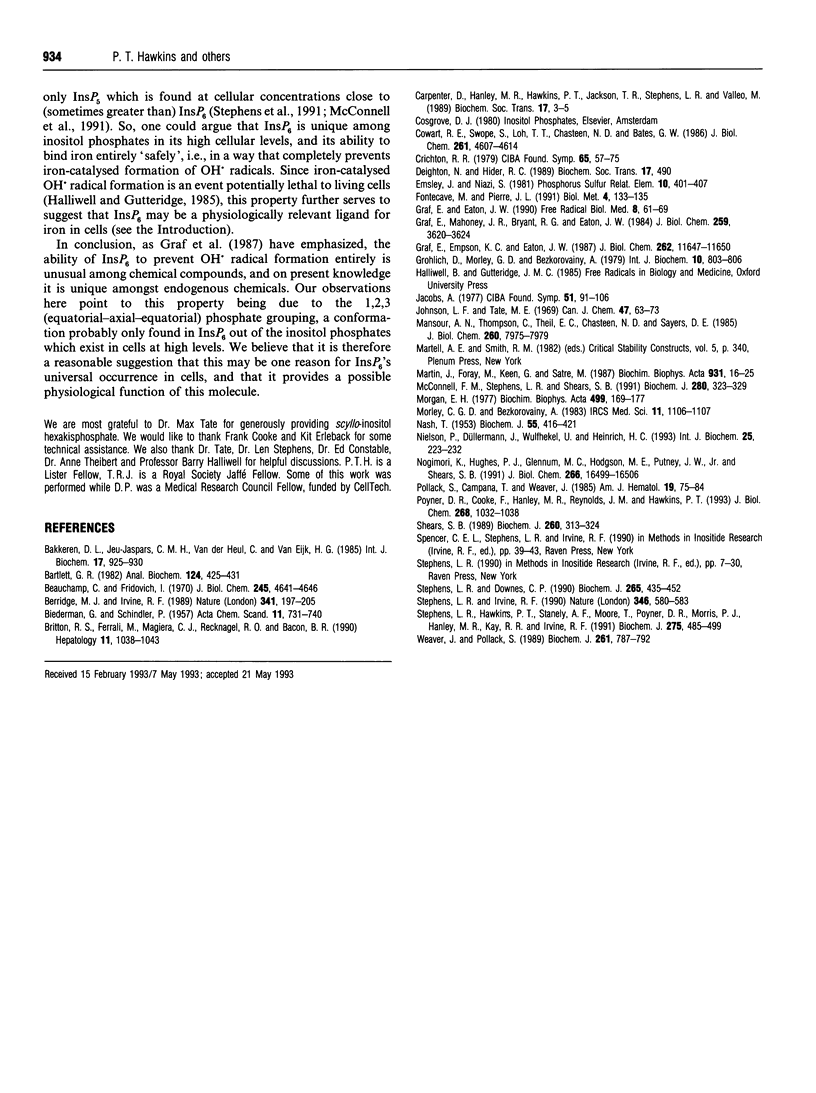
Images in this article
Selected References
These references are in PubMed. This may not be the complete list of references from this article.
- Bakkeren D. L., de Jeu-Jaspars C. M., van der Heul C., van Eijk H. G. Analysis of iron-binding components in the low molecular weight fraction of rat reticulocyte cytosol. Int J Biochem. 1985;17(8):925–930. doi: 10.1016/0020-711x(85)90177-6. [DOI] [PubMed] [Google Scholar]
- Bartlett G. R. Isolation and assay of red-cell inositol polyphosphates. Anal Biochem. 1982 Aug;124(2):425–431. doi: 10.1016/0003-2697(82)90060-4. [DOI] [PubMed] [Google Scholar]
- Beauchamp C., Fridovich I. A mechanism for the production of ethylene from methional. The generation of the hydroxyl radical by xanthine oxidase. J Biol Chem. 1970 Sep 25;245(18):4641–4646. [PubMed] [Google Scholar]
- Berridge M. J., Irvine R. F. Inositol phosphates and cell signalling. Nature. 1989 Sep 21;341(6239):197–205. doi: 10.1038/341197a0. [DOI] [PubMed] [Google Scholar]
- Britton R. S., Ferrali M., Magiera C. J., Recknagel R. O., Bacon B. R. Increased prooxidant action of hepatic cytosolic low-molecular-weight iron in experimental iron overload. Hepatology. 1990 Jun;11(6):1038–1043. doi: 10.1002/hep.1840110620. [DOI] [PubMed] [Google Scholar]
- Carpenter D., Hanley M. R., Hawkins P. T., Jackson T. R., Stephens L. R., Vallejo M. The metabolism and functions of inositol pentakisphosphate and inositol hexakisphosphate. Biochem Soc Trans. 1989 Feb;17(1):3–5. doi: 10.1042/bst0170003. [DOI] [PubMed] [Google Scholar]
- Cowart R. E., Swope S., Loh T. T., Chasteen N. D., Bates G. W. The exchange of Fe3+ between pyrophosphate and transferrin. Probing the nature of an intermediate complex with stopped flow kinetics, rapid multimixing, and electron paramagnetic resonance spectroscopy. J Biol Chem. 1986 Apr 5;261(10):4607–4614. [PubMed] [Google Scholar]
- Crichton R. R. Interactions between iron metabolism and oxygen activation. Ciba Found Symp. 1978 Jun 6;(65):57–76. doi: 10.1002/9780470715413.ch5. [DOI] [PubMed] [Google Scholar]
- Fontecave M., Pierre J. L. Iron metabolism: the low-molecular-mass iron pool. Biol Met. 1991;4(3):133–135. doi: 10.1007/BF01141302. [DOI] [PubMed] [Google Scholar]
- Graf E., Eaton J. W. Antioxidant functions of phytic acid. Free Radic Biol Med. 1990;8(1):61–69. doi: 10.1016/0891-5849(90)90146-a. [DOI] [PubMed] [Google Scholar]
- Graf E., Empson K. L., Eaton J. W. Phytic acid. A natural antioxidant. J Biol Chem. 1987 Aug 25;262(24):11647–11650. [PubMed] [Google Scholar]
- Graf E., Mahoney J. R., Bryant R. G., Eaton J. W. Iron-catalyzed hydroxyl radical formation. Stringent requirement for free iron coordination site. J Biol Chem. 1984 Mar 25;259(6):3620–3624. [PubMed] [Google Scholar]
- Herzberg G. R. Purification and properties of liver phosphofructokinase from normal and obese mice. Int J Biochem. 1979;10(10):803–806. doi: 10.1016/0020-711x(79)90052-1. [DOI] [PubMed] [Google Scholar]
- Jacobs A. An intracellular transit iron pool. Ciba Found Symp. 1976 Dec 7;(51):91–106. doi: 10.1002/9780470720325.ch5. [DOI] [PubMed] [Google Scholar]
- Mansour A. N., Thompson C., Theil E. C., Chasteen N. D., Sayers D. E. Fe(III).ATP complexes. Models for ferritin and other polynuclear iron complexes with phosphate. J Biol Chem. 1985 Jul 5;260(13):7975–7979. [PubMed] [Google Scholar]
- Martin J. B., Foray M. F., Klein G., Satre M. Identification of inositol hexaphosphate in 31P-NMR spectra of Dictyostelium discoideum amoebae. Relevance to intracellular pH determination. Biochim Biophys Acta. 1987 Oct 22;931(1):16–25. doi: 10.1016/0167-4889(87)90045-0. [DOI] [PubMed] [Google Scholar]
- McConnell F. M., Stephens L. R., Shears S. B. Multiple isomers of inositol pentakisphosphate in Epstein-Barr-virus- transformed (T5-1) B-lymphocytes. Identification of inositol 1,3,4,5,6-pentakisphosphate, D-inositol 1,2,4,5,6-pentakisphosphate and L-inositol 1,2,4,5,6-pentakisphosphate. Biochem J. 1991 Dec 1;280(Pt 2):323–329. doi: 10.1042/bj2800323. [DOI] [PMC free article] [PubMed] [Google Scholar]
- Morgan E. H. Iron exchange between transferrin molecules mediated by phosphate compounds and other cell metabolites. Biochim Biophys Acta. 1977 Aug 25;499(1):169–177. doi: 10.1016/0304-4165(77)90239-2. [DOI] [PubMed] [Google Scholar]
- NASH T. The colorimetric estimation of formaldehyde by means of the Hantzsch reaction. Biochem J. 1953 Oct;55(3):416–421. doi: 10.1042/bj0550416. [DOI] [PMC free article] [PubMed] [Google Scholar]
- Nielsen P., Düllmann J., Wulfhekel U., Heinrich H. C. Non-transferrin-bound-iron in serum and low-molecular-weight-iron in the liver of dietary iron-loaded rats. Int J Biochem. 1993 Feb;25(2):223–232. doi: 10.1016/0020-711x(93)90010-c. [DOI] [PubMed] [Google Scholar]
- Nogimori K., Hughes P. J., Glennon M. C., Hodgson M. E., Putney J. W., Jr, Shears S. B. Purification of an inositol (1,3,4,5)-tetrakisphosphate 3-phosphatase activity from rat liver and the evaluation of its substrate specificity. J Biol Chem. 1991 Sep 5;266(25):16499–16506. [PubMed] [Google Scholar]
- Pollack S., Campana T., Weaver J. Low molecular weight iron in guinea pig reticulocytes. Am J Hematol. 1985 May;19(1):75–84. doi: 10.1002/ajh.2830190110. [DOI] [PubMed] [Google Scholar]
- Poyner D. R., Cooke F., Hanley M. R., Reynolds D. J., Hawkins P. T. Characterization of metal ion-induced [3H]inositol hexakisphosphate binding to rat cerebellar membranes. J Biol Chem. 1993 Jan 15;268(2):1032–1038. [PubMed] [Google Scholar]
- Shears S. B. Metabolism of the inositol phosphates produced upon receptor activation. Biochem J. 1989 Jun 1;260(2):313–324. doi: 10.1042/bj2600313. [DOI] [PMC free article] [PubMed] [Google Scholar]
- Stephens L. R., Downes C. P. Product-precursor relationships amongst inositol polyphosphates. Incorporation of [32P]Pi into myo-inositol 1,3,4,6-tetrakisphosphate, myo-inositol 1,3,4,5-tetrakisphosphate, myo-inositol 3,4,5,6-tetrakisphosphate and myo-inositol 1,3,4,5,6-pentakisphosphate in intact avian erythrocytes. Biochem J. 1990 Jan 15;265(2):435–452. doi: 10.1042/bj2650435. [DOI] [PMC free article] [PubMed] [Google Scholar]
- Stephens L. R., Hawkins P. T., Stanley A. F., Moore T., Poyner D. R., Morris P. J., Hanley M. R., Kay R. R., Irvine R. F. myo-inositol pentakisphosphates. Structure, biological occurrence and phosphorylation to myo-inositol hexakisphosphate. Biochem J. 1991 Apr 15;275(Pt 2):485–499. doi: 10.1042/bj2750485. [DOI] [PMC free article] [PubMed] [Google Scholar]
- Stephens L. R., Irvine R. F. Stepwise phosphorylation of myo-inositol leading to myo-inositol hexakisphosphate in Dictyostelium. Nature. 1990 Aug 9;346(6284):580–583. doi: 10.1038/346580a0. [DOI] [PubMed] [Google Scholar]
- Weaver J., Pollack S. Low-Mr iron isolated from guinea pig reticulocytes as AMP-Fe and ATP-Fe complexes. Biochem J. 1989 Aug 1;261(3):787–792. doi: 10.1042/bj2610787. [DOI] [PMC free article] [PubMed] [Google Scholar]