Abstract
Calmodulin-dependent protein phosphatase has been proposed to be an important phosphotyrosyl-protein phosphatase. The ability of the enzyme to attack autophosphorylated insulin receptor was examined and compared with the known ability of the enzyme to act on autophosphorylated epidermal-growth-factor (EGF) receptor. Purified calmodulin-dependent protein phosphatase was shown to catalyse the complete dephosphorylation of phosphotyrosyl-(insulin receptor). When compared at similar concentrations, 32P-labelled EGF receptor was dephosphorylated at greater than 3 times the rate of 32P-labelled insulin receptor; both dephosphorylations exhibited similar dependence on metal ions and calmodulin. Native phosphotyrosyl-protein phosphatases in cell extracts were also characterized. With rat liver, heart or brain, most (75%) of the native phosphatase activity against both 32P-labelled insulin and EGF receptors was recovered in the particulate fraction of the cell, with only 25% in the soluble fraction. This subcellular distribution contrasts with results of previous studies using artificial substrates, which found most of the phosphotyrosyl-protein phosphatase activity in the soluble fraction of the cell. Properties of particulate and soluble phosphatase activity against 32P-labelled insulin and EGF receptors are reported. The contribution of calmodulin-dependent protein phosphatase activity to phosphotyrosyl-protein phosphatase activity in cell fractions was determined by utilizing the unique metal-ion dependence of calmodulin-dependent protein phosphatase. Whereas Ni2+ (1 mM) markedly activated the calmodulin-dependent protein phosphatase, it was found to inhibit potently both particulate and soluble phosphotyrosyl-protein phosphatase activity. In fractions from rat liver, brain and heart, total phosphotyrosyl-protein phosphatase activity against both 32P-labelled receptors was inhibited by 99.5 +/- 6% (mean +/- S.E.M., 30 observations) by Ni2+. Results of Ni2+ inhibition studies were confirmed by other methods. It is concluded that in cell extracts phosphotyrosyl-protein phosphatases other than calmodulin-dependent protein phosphatase are the major phosphotyrosyl-(insulin receptor) and -(EGF receptor) phosphatases.
Full text
PDF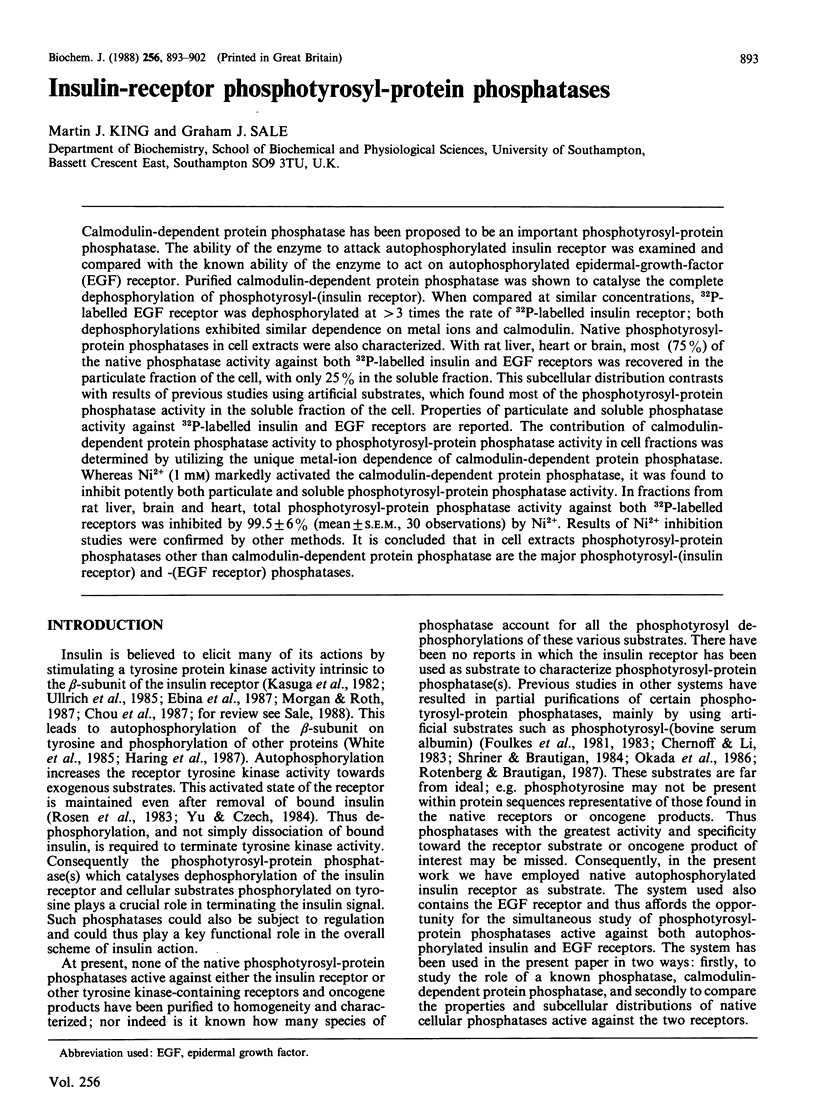
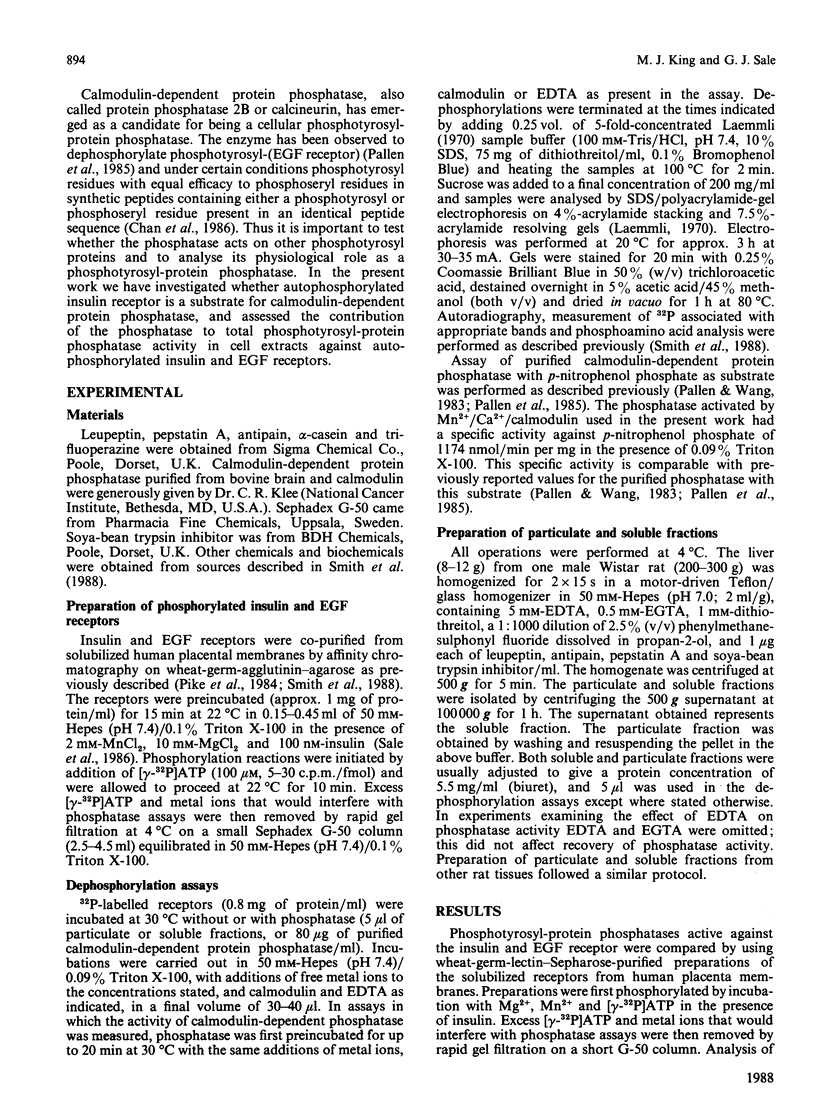
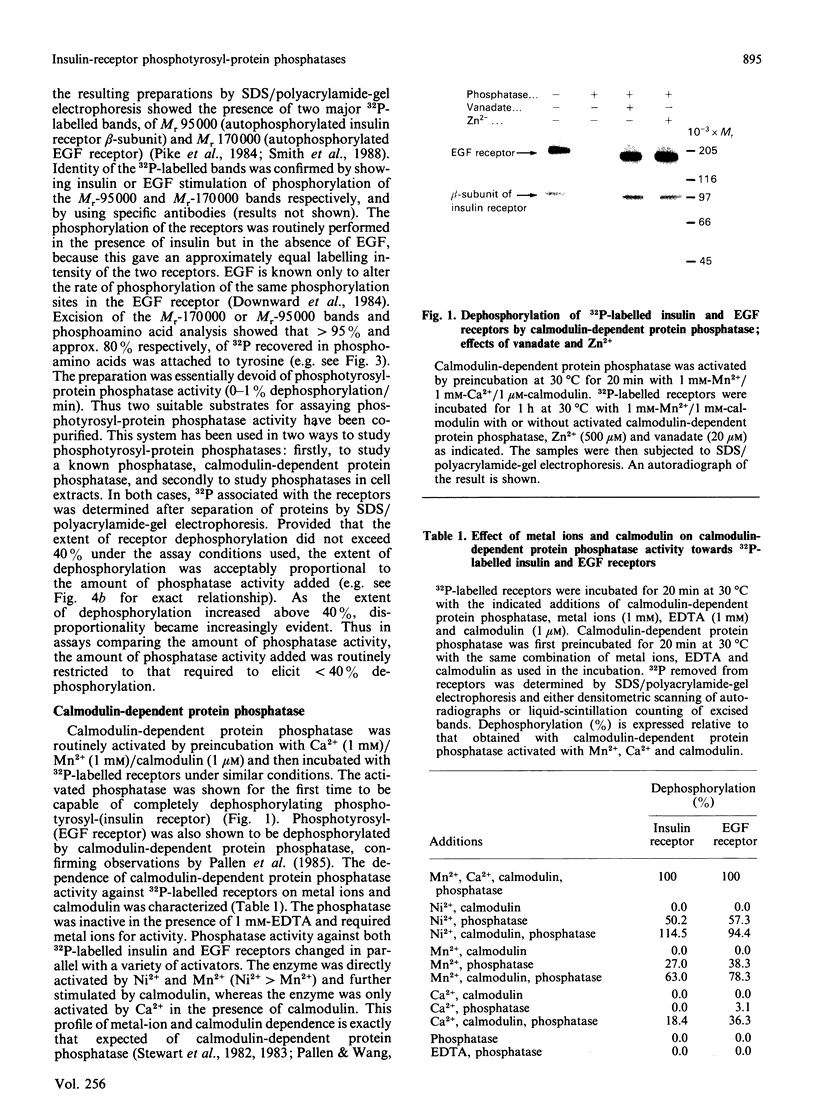
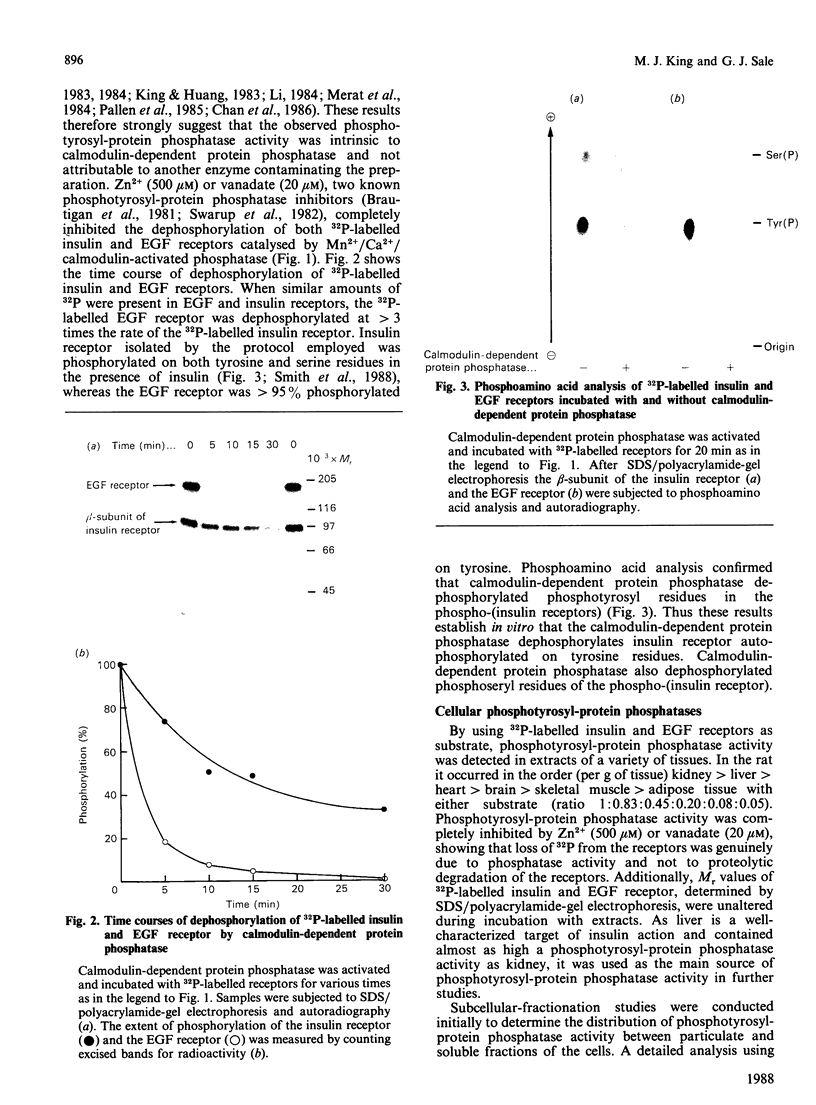
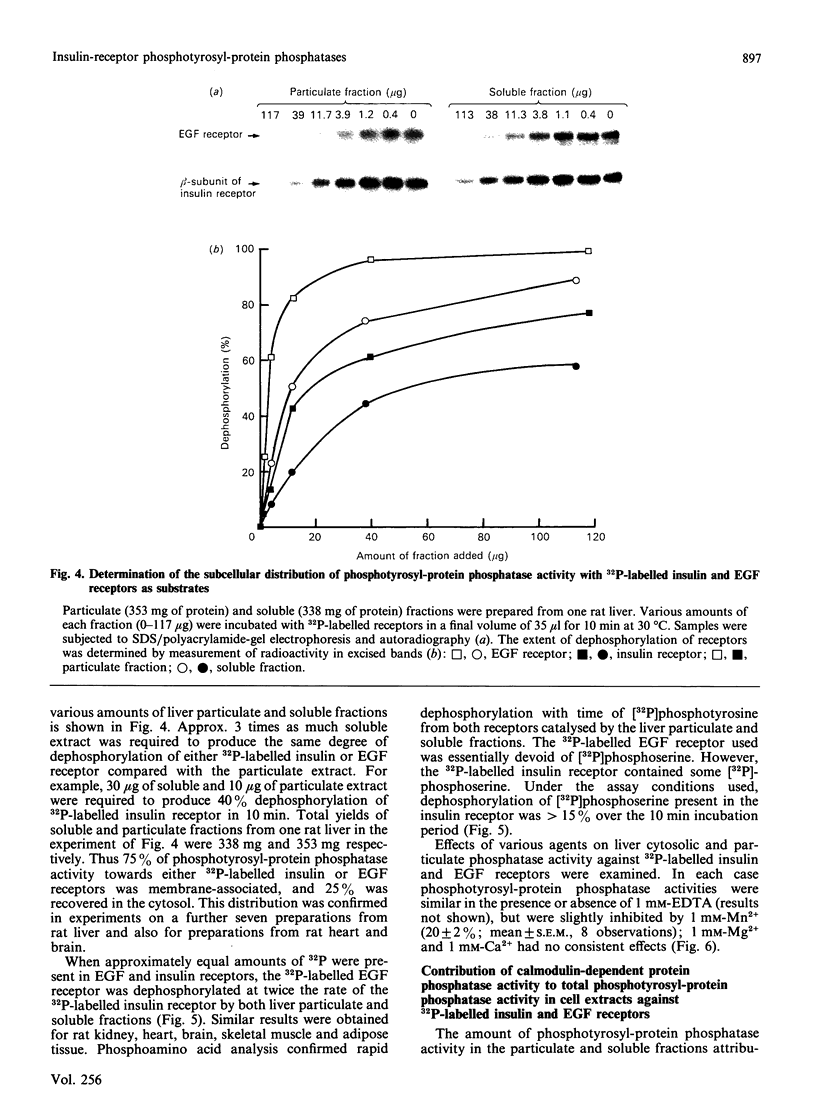
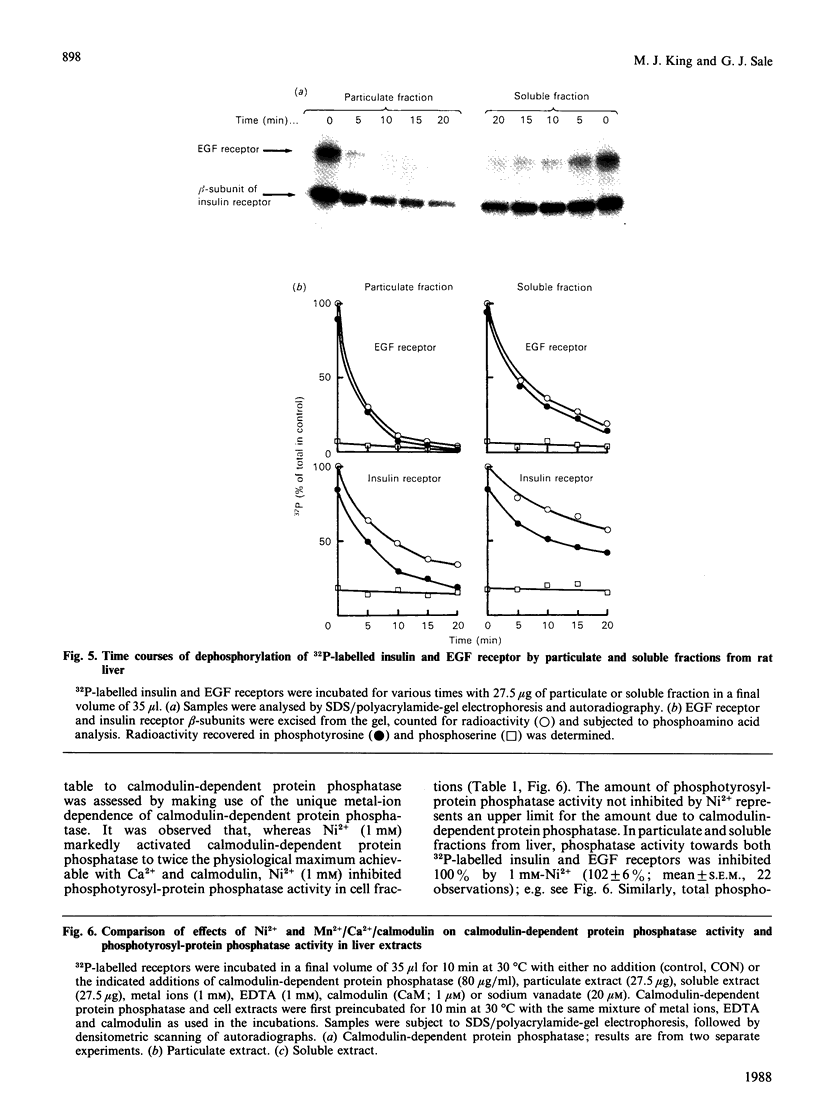
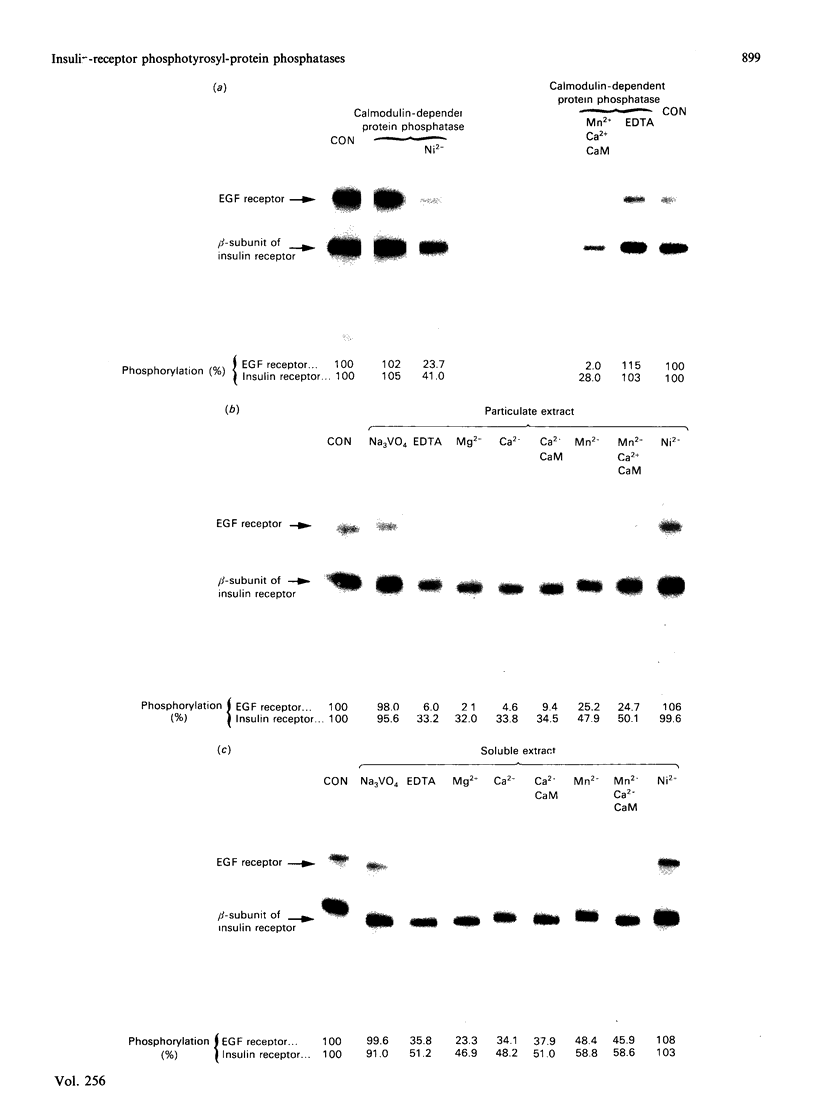
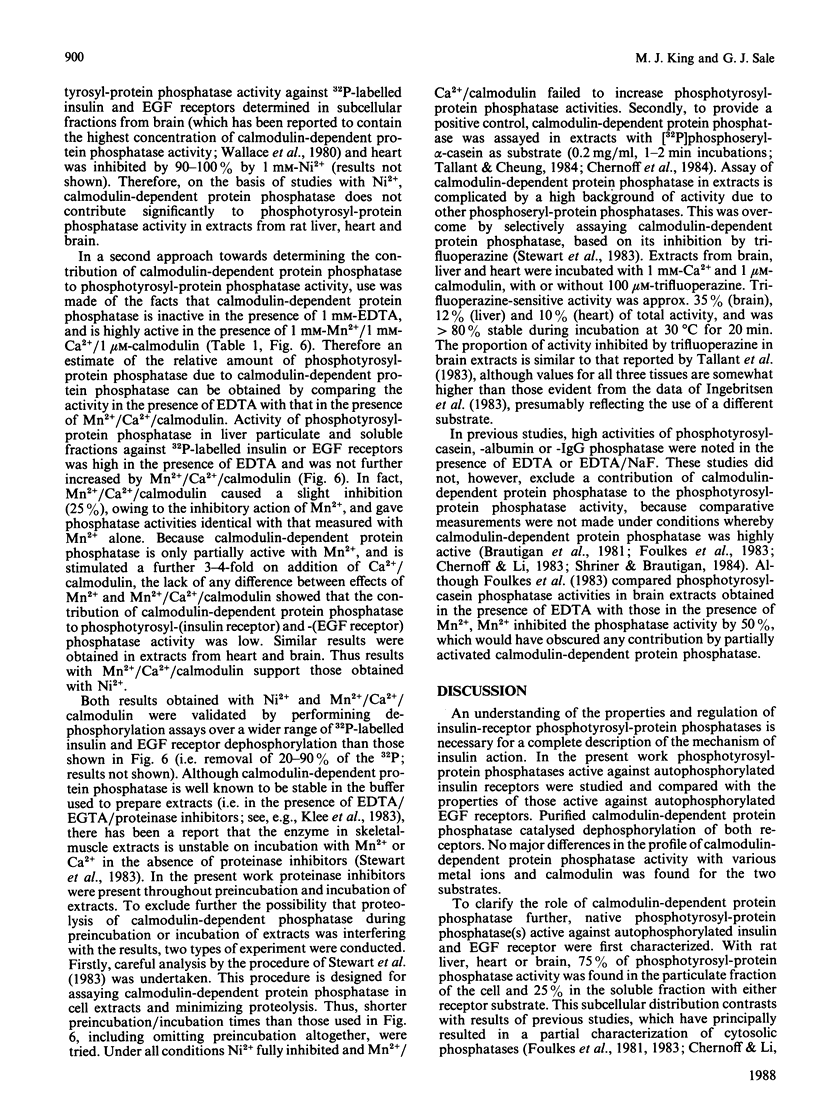
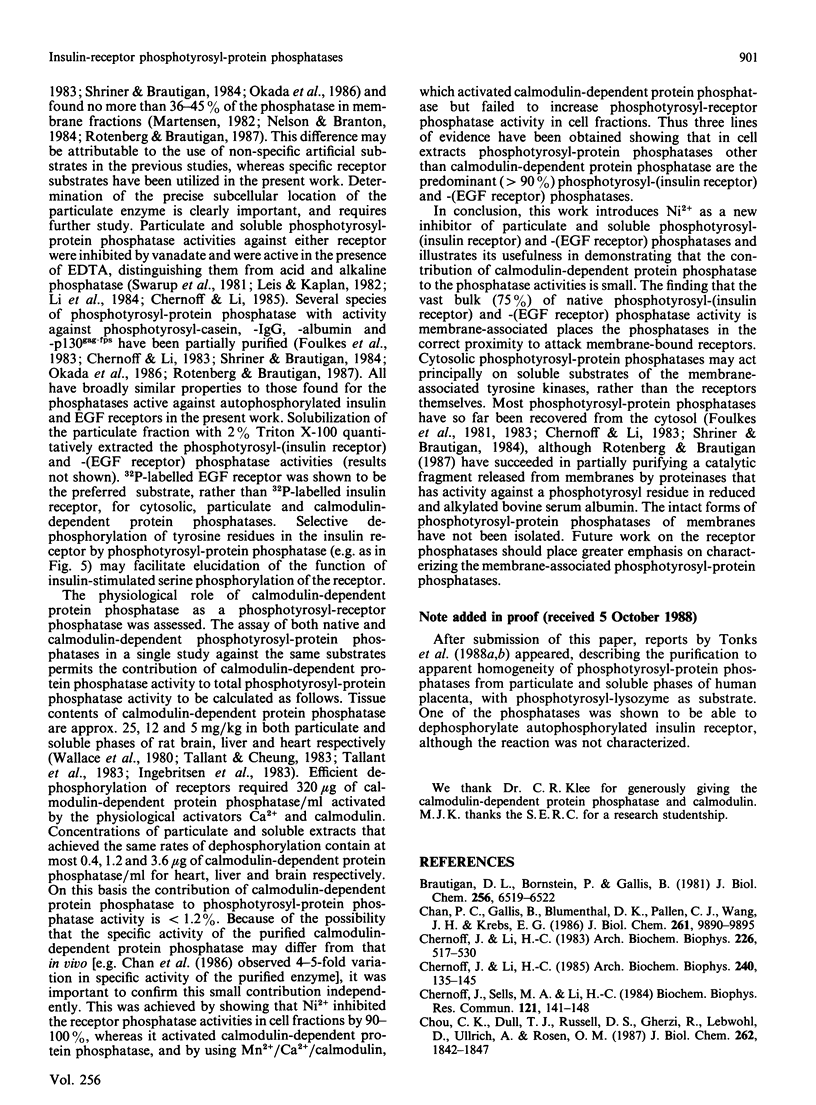
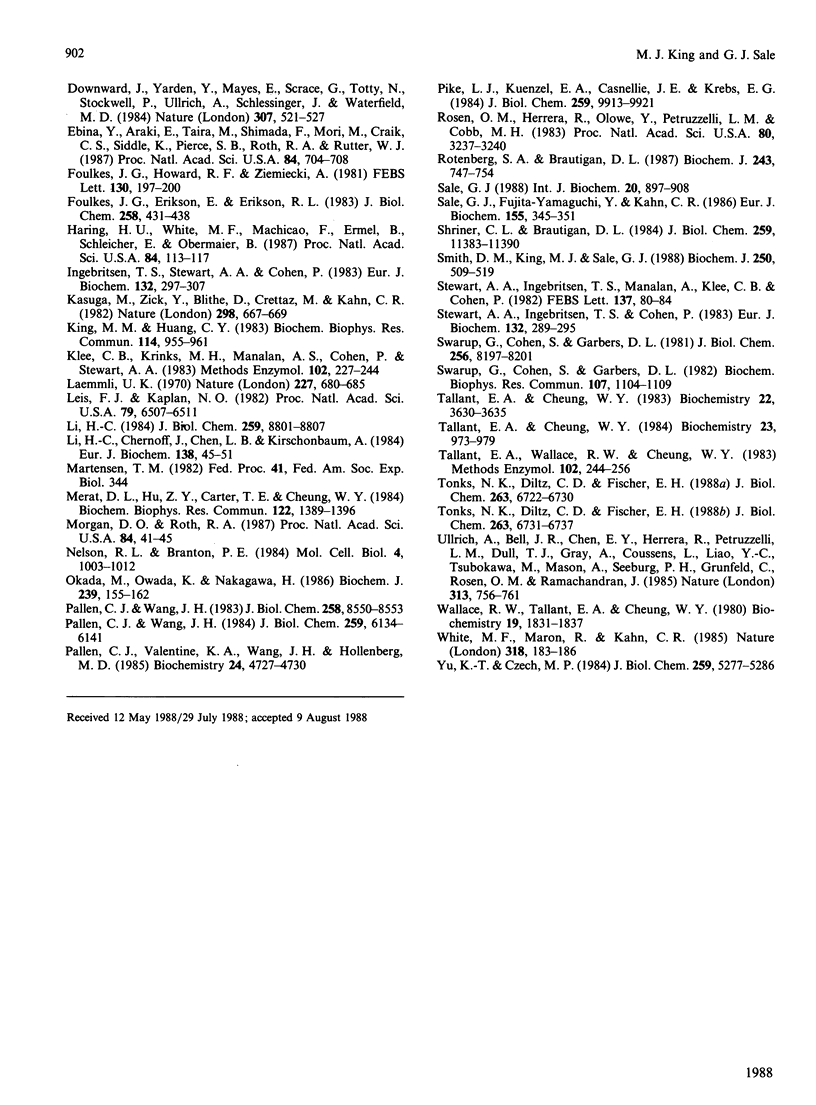
Images in this article
Selected References
These references are in PubMed. This may not be the complete list of references from this article.
- Brautigan D. L., Bornstein P., Gallis B. Phosphotyrosyl-protein phosphatase. Specific inhibition by Zn. J Biol Chem. 1981 Jul 10;256(13):6519–6522. [PubMed] [Google Scholar]
- Chan C. P., Gallis B., Blumenthal D. K., Pallen C. J., Wang J. H., Krebs E. G. Characterization of the phosphotyrosyl protein phosphatase activity of calmodulin-dependent protein phosphatase. J Biol Chem. 1986 Jul 25;261(21):9890–9895. [PubMed] [Google Scholar]
- Chernoff J., Li H. C. A major phosphotyrosyl-protein phosphatase from bovine heart is associated with a low-molecular-weight acid phosphatase. Arch Biochem Biophys. 1985 Jul;240(1):135–145. doi: 10.1016/0003-9861(85)90016-5. [DOI] [PubMed] [Google Scholar]
- Chernoff J., Li H. C. Multiple forms of phosphotyrosyl- and phosphoseryl-protein phosphatase from cardiac muscle: partial purification and characterization of an EDTA-stimulated phosphotyrosyl-protein phosphatase. Arch Biochem Biophys. 1983 Oct 15;226(2):517–530. doi: 10.1016/0003-9861(83)90321-1. [DOI] [PubMed] [Google Scholar]
- Chernoff J., Sells M. A., Li H. C. Characterization of phosphotyrosyl-protein phosphatase activity associated with calcineurin. Biochem Biophys Res Commun. 1984 May 31;121(1):141–148. doi: 10.1016/0006-291x(84)90698-3. [DOI] [PubMed] [Google Scholar]
- Chou C. K., Dull T. J., Russell D. S., Gherzi R., Lebwohl D., Ullrich A., Rosen O. M. Human insulin receptors mutated at the ATP-binding site lack protein tyrosine kinase activity and fail to mediate postreceptor effects of insulin. J Biol Chem. 1987 Feb 5;262(4):1842–1847. [PubMed] [Google Scholar]
- Downward J., Yarden Y., Mayes E., Scrace G., Totty N., Stockwell P., Ullrich A., Schlessinger J., Waterfield M. D. Close similarity of epidermal growth factor receptor and v-erb-B oncogene protein sequences. Nature. 1984 Feb 9;307(5951):521–527. doi: 10.1038/307521a0. [DOI] [PubMed] [Google Scholar]
- Ebina Y., Araki E., Taira M., Shimada F., Mori M., Craik C. S., Siddle K., Pierce S. B., Roth R. A., Rutter W. J. Replacement of lysine residue 1030 in the putative ATP-binding region of the insulin receptor abolishes insulin- and antibody-stimulated glucose uptake and receptor kinase activity. Proc Natl Acad Sci U S A. 1987 Feb;84(3):704–708. doi: 10.1073/pnas.84.3.704. [DOI] [PMC free article] [PubMed] [Google Scholar]
- Foulkes J. G., Erikson E., Erikson R. L. Separation of multiple phosphotyrosyl-and phosphoseryl-protein phosphatases from chicken brain. J Biol Chem. 1983 Jan 10;258(1):431–438. [PubMed] [Google Scholar]
- Foulkes J. G., Howard R. F., Ziemiecki A. Detection of a novel mammalian protein phosphatase with activity for phosphotyrosine. FEBS Lett. 1981 Aug 3;130(2):197–200. doi: 10.1016/0014-5793(81)81118-0. [DOI] [PubMed] [Google Scholar]
- Häring H. U., White M. F., Machicao F., Ermel B., Schleicher E., Obermaier B. Insulin rapidly stimulates phosphorylation of a 46-kDa membrane protein on tyrosine residues as well as phosphorylation of several soluble proteins in intact fat cells. Proc Natl Acad Sci U S A. 1987 Jan;84(1):113–117. doi: 10.1073/pnas.84.1.113. [DOI] [PMC free article] [PubMed] [Google Scholar]
- Ingebritsen T. S., Stewart A. A., Cohen P. The protein phosphatases involved in cellular regulation. 6. Measurement of type-1 and type-2 protein phosphatases in extracts of mammalian tissues; an assessment of their physiological roles. Eur J Biochem. 1983 May 2;132(2):297–307. doi: 10.1111/j.1432-1033.1983.tb07362.x. [DOI] [PubMed] [Google Scholar]
- Kasuga M., Zick Y., Blithe D. L., Crettaz M., Kahn C. R. Insulin stimulates tyrosine phosphorylation of the insulin receptor in a cell-free system. Nature. 1982 Aug 12;298(5875):667–669. doi: 10.1038/298667a0. [DOI] [PubMed] [Google Scholar]
- King M. M., Huang C. Y. Activation of calcineurin by nickel ions. Biochem Biophys Res Commun. 1983 Aug 12;114(3):955–961. doi: 10.1016/0006-291x(83)90653-8. [DOI] [PubMed] [Google Scholar]
- Klee C. B., Krinks M. H., Manalan A. S., Cohen P., Stewart A. A. Isolation and characterization of bovine brain calcineurin: a calmodulin-stimulated protein phosphatase. Methods Enzymol. 1983;102:227–244. doi: 10.1016/s0076-6879(83)02024-8. [DOI] [PubMed] [Google Scholar]
- Laemmli U. K. Cleavage of structural proteins during the assembly of the head of bacteriophage T4. Nature. 1970 Aug 15;227(5259):680–685. doi: 10.1038/227680a0. [DOI] [PubMed] [Google Scholar]
- Leis J. F., Kaplan N. O. An acid phosphatase in the plasma membranes of human astrocytoma showing marked specificity toward phosphotyrosine protein. Proc Natl Acad Sci U S A. 1982 Nov;79(21):6507–6511. doi: 10.1073/pnas.79.21.6507. [DOI] [PMC free article] [PubMed] [Google Scholar]
- Li H. C. Activation of brain calcineurin phosphatase towards nonprotein phosphoesters by Ca2+, calmodulin, and Mg2+. J Biol Chem. 1984 Jul 25;259(14):8801–8807. [PubMed] [Google Scholar]
- Li H. C., Chernoff J., Chen L. B., Kirschonbaum A. A phosphotyrosyl-protein phosphatase activity associated with acid phosphatase from human prostate gland. Eur J Biochem. 1984 Jan 2;138(1):45–51. doi: 10.1111/j.1432-1033.1984.tb07879.x. [DOI] [PubMed] [Google Scholar]
- Merat D. L., Hu Z. Y., Carter T. E., Cheung W. Y. Subunit A of calmodulin-dependent protein phosphatase requires Mn2+ for activity. Biochem Biophys Res Commun. 1984 Aug 16;122(3):1389–1396. doi: 10.1016/0006-291x(84)91245-2. [DOI] [PubMed] [Google Scholar]
- Morgan D. O., Roth R. A. Acute insulin action requires insulin receptor kinase activity: introduction of an inhibitory monoclonal antibody into mammalian cells blocks the rapid effects of insulin. Proc Natl Acad Sci U S A. 1987 Jan;84(1):41–45. doi: 10.1073/pnas.84.1.41. [DOI] [PMC free article] [PubMed] [Google Scholar]
- Nelson R. L., Branton P. E. Identification, purification, and characterization of phosphotyrosine-specific protein phosphatases from cultured chicken embryo fibroblasts. Mol Cell Biol. 1984 Jun;4(6):1003–1012. doi: 10.1128/mcb.4.6.1003. [DOI] [PMC free article] [PubMed] [Google Scholar]
- Okada M., Owada K., Nakagawa H. [Phosphotyrosine]protein phosphatase in rat brain. A major [phosphotyrosine]protein phosphatase is a 23 kDa protein distinct from acid phosphatase. Biochem J. 1986 Oct 1;239(1):155–162. doi: 10.1042/bj2390155. [DOI] [PMC free article] [PubMed] [Google Scholar]
- Pallen C. J., Valentine K. A., Wang J. H., Hollenberg M. D. Calcineurin-mediated dephosphorylation of the human placental membrane receptor for epidermal growth factor urogastrone. Biochemistry. 1985 Aug 27;24(18):4727–4730. doi: 10.1021/bi00339a003. [DOI] [PubMed] [Google Scholar]
- Pallen C. J., Wang J. H. Calmodulin-stimulated dephosphorylation of p-nitrophenyl phosphate and free phosphotyrosine by calcineurin. J Biol Chem. 1983 Jul 25;258(14):8550–8553. [PubMed] [Google Scholar]
- Pallen C. J., Wang J. H. Regulation of calcineurin by metal ions. Mechanism of activation by Ni2+ and an enhanced response to Ca2+/calmodulin. J Biol Chem. 1984 May 25;259(10):6134–6141. [PubMed] [Google Scholar]
- Pike L. J., Kuenzel E. A., Casnellie J. E., Krebs E. G. A comparison of the insulin- and epidermal growth factor-stimulated protein kinases from human placenta. J Biol Chem. 1984 Aug 10;259(15):9913–9921. [PubMed] [Google Scholar]
- Rosen O. M., Herrera R., Olowe Y., Petruzzelli L. M., Cobb M. H. Phosphorylation activates the insulin receptor tyrosine protein kinase. Proc Natl Acad Sci U S A. 1983 Jun;80(11):3237–3240. doi: 10.1073/pnas.80.11.3237. [DOI] [PMC free article] [PubMed] [Google Scholar]
- Rotenberg S. A., Brautigan D. L. Membrane protein phosphotyrosine phosphatase in rabbit kidney. Proteolysis activates the enzyme and generates soluble catalytic fragments. Biochem J. 1987 May 1;243(3):747–754. doi: 10.1042/bj2430747. [DOI] [PMC free article] [PubMed] [Google Scholar]
- Sale G. J., Fujita-Yamaguchi Y., Kahn C. R. Characterization of phosphatidylinositol kinase activity associated with the insulin receptor. Eur J Biochem. 1986 Mar 3;155(2):345–351. doi: 10.1111/j.1432-1033.1986.tb09497.x. [DOI] [PubMed] [Google Scholar]
- Sale G. J. Recent progress in our understanding of the mechanism of action of insulin. Int J Biochem. 1988;20(9):897–908. doi: 10.1016/0020-711x(88)90173-5. [DOI] [PubMed] [Google Scholar]
- Shriner C. L., Brautigan D. L. Cytosolic protein phosphotyrosine phosphatases from rabbit kidney. Purification of two distinct enzymes that bind to Zn2+-iminodiacetate agarose. J Biol Chem. 1984 Sep 25;259(18):11383–11390. [PubMed] [Google Scholar]
- Smith D. M., King M. J., Sale G. J. Two systems in vitro that show insulin-stimulated serine kinase activity towards the insulin receptor. Biochem J. 1988 Mar 1;250(2):509–519. doi: 10.1042/bj2500509. [DOI] [PMC free article] [PubMed] [Google Scholar]
- Stewart A. A., Ingebritsen T. S., Cohen P. The protein phosphatases involved in cellular regulation. 5. Purification and properties of a Ca2+/calmodulin-dependent protein phosphatase (2B) from rabbit skeletal muscle. Eur J Biochem. 1983 May 2;132(2):289–295. doi: 10.1111/j.1432-1033.1983.tb07361.x. [DOI] [PubMed] [Google Scholar]
- Stewart A. A., Ingebritsen T. S., Manalan A., Klee C. B., Cohen P. Discovery of a Ca2+- and calmodulin-dependent protein phosphatase: probable identity with calcineurin (CaM-BP80). FEBS Lett. 1982 Jan 11;137(1):80–84. doi: 10.1016/0014-5793(82)80319-0. [DOI] [PubMed] [Google Scholar]
- Swarup G., Cohen S., Garbers D. L. Inhibition of membrane phosphotyrosyl-protein phosphatase activity by vanadate. Biochem Biophys Res Commun. 1982 Aug;107(3):1104–1109. doi: 10.1016/0006-291x(82)90635-0. [DOI] [PubMed] [Google Scholar]
- Swarup G., Cohen S., Garbers D. L. Selective dephosphorylation of proteins containing phosphotyrosine by alkaline phosphatases. J Biol Chem. 1981 Aug 10;256(15):8197–8201. [PubMed] [Google Scholar]
- Tallant E. A., Cheung W. Y. Activation of bovine brain calmodulin-dependent protein phosphatase by limited trypsinization. Biochemistry. 1984 Feb 28;23(5):973–979. doi: 10.1021/bi00300a027. [DOI] [PubMed] [Google Scholar]
- Tallant E. A., Cheung W. Y. Calmodulin-dependent protein phosphatase: a developmental study. Biochemistry. 1983 Jul 19;22(15):3630–3635. doi: 10.1021/bi00284a014. [DOI] [PubMed] [Google Scholar]
- Tallant E. A., Wallace R. W., Cheung W. Y. Purification and radioimmunoassay of calmodulin-dependent protein phosphatase from bovine brain. Methods Enzymol. 1983;102:244–256. doi: 10.1016/s0076-6879(83)02025-x. [DOI] [PubMed] [Google Scholar]
- Tonks N. K., Diltz C. D., Fischer E. H. Characterization of the major protein-tyrosine-phosphatases of human placenta. J Biol Chem. 1988 May 15;263(14):6731–6737. [PubMed] [Google Scholar]
- Tonks N. K., Diltz C. D., Fischer E. H. Purification of the major protein-tyrosine-phosphatases of human placenta. J Biol Chem. 1988 May 15;263(14):6722–6730. [PubMed] [Google Scholar]
- Ullrich A., Bell J. R., Chen E. Y., Herrera R., Petruzzelli L. M., Dull T. J., Gray A., Coussens L., Liao Y. C., Tsubokawa M. Human insulin receptor and its relationship to the tyrosine kinase family of oncogenes. 1985 Feb 28-Mar 6Nature. 313(6005):756–761. doi: 10.1038/313756a0. [DOI] [PubMed] [Google Scholar]
- Wallace R. W., Tallant E. A., Cheung W. Y. High levels of a heat-labile calmodulin-binding protein (CaM-BP80) in bovine neostriatum. Biochemistry. 1980 Apr 29;19(9):1831–1837. doi: 10.1021/bi00550a016. [DOI] [PubMed] [Google Scholar]
- White M. F., Maron R., Kahn C. R. Insulin rapidly stimulates tyrosine phosphorylation of a Mr-185,000 protein in intact cells. Nature. 1985 Nov 14;318(6042):183–186. doi: 10.1038/318183a0. [DOI] [PubMed] [Google Scholar]
- Yu K. T., Czech M. P. Tyrosine phosphorylation of the insulin receptor beta subunit activates the receptor-associated tyrosine kinase activity. J Biol Chem. 1984 Apr 25;259(8):5277–5286. [PubMed] [Google Scholar]