Abstract
When [14C]arachidonoyl-CoA was incubated with crude extracts of rat liver microsomes, [14C]arachidonic acid was incorporated into many proteins, suggesting that modification of these proteins with fatty acid, i.e. acylation, occurred. Using a [14C]arachidonyl-CoA labelling assay, 50 and 53 kDa proteins were purified from rat liver microsomes to near homogeneity by sequential chromatography on Red-Toyopearl, hydroxyapatite, heparin-Toyopearl, Blue-Toyopearl and UDP-hexanolamine-agarose. Acylation of the 50 and 53 kDa proteins occurred in the absence of any other protein, suggesting that these molecules catalyse autoacylation. The acylation was dependent on the length of the incubation period and the concentration of [14C]arachidonoyl-CoA. The 50 and 53 kDa proteins also had acyl-CoA-binding activity; initial rates of acyl-CoA binding and acylation were 0.25 and 0.004 min-1 respectively. The proteins also had weak but distinct acyl-CoA-hydrolysing activity (0.006 min-1). These results suggest that the proteins catalysed the sequential reactions of binding to acyl-CoA, autoacylation, and hydrolysis of fatty acid. N-terminal amino acid sequencing analysis showed these proteins to be UDP-glucuronosyltransferase (UDPGT) isoforms. UDPGT activity was inhibited by arachidonoyl-CoA. These results suggest that binding of acyl-CoA and acylation of UDPGT isoforms regulate the enzyme activities, implying a possible novel function for fatty acyl-CoA in glucuronidation, which is involved in the metabolism of drugs, steroids and bilirubin.
Full text
PDF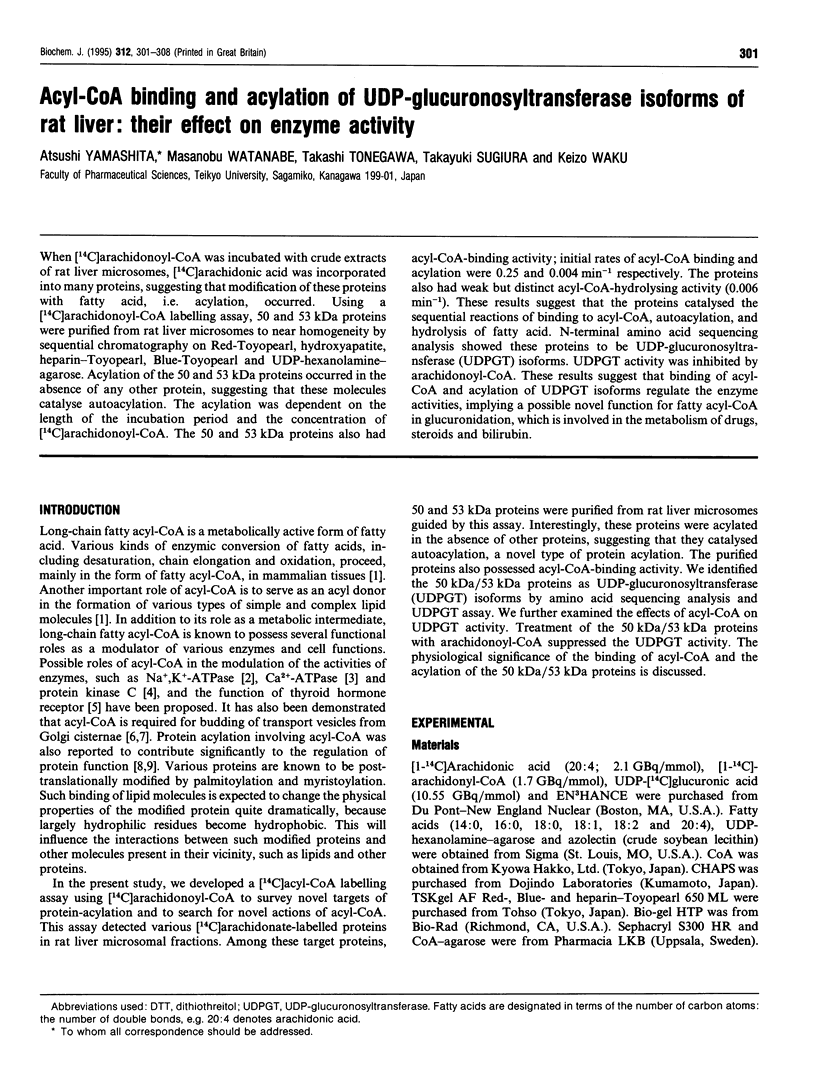
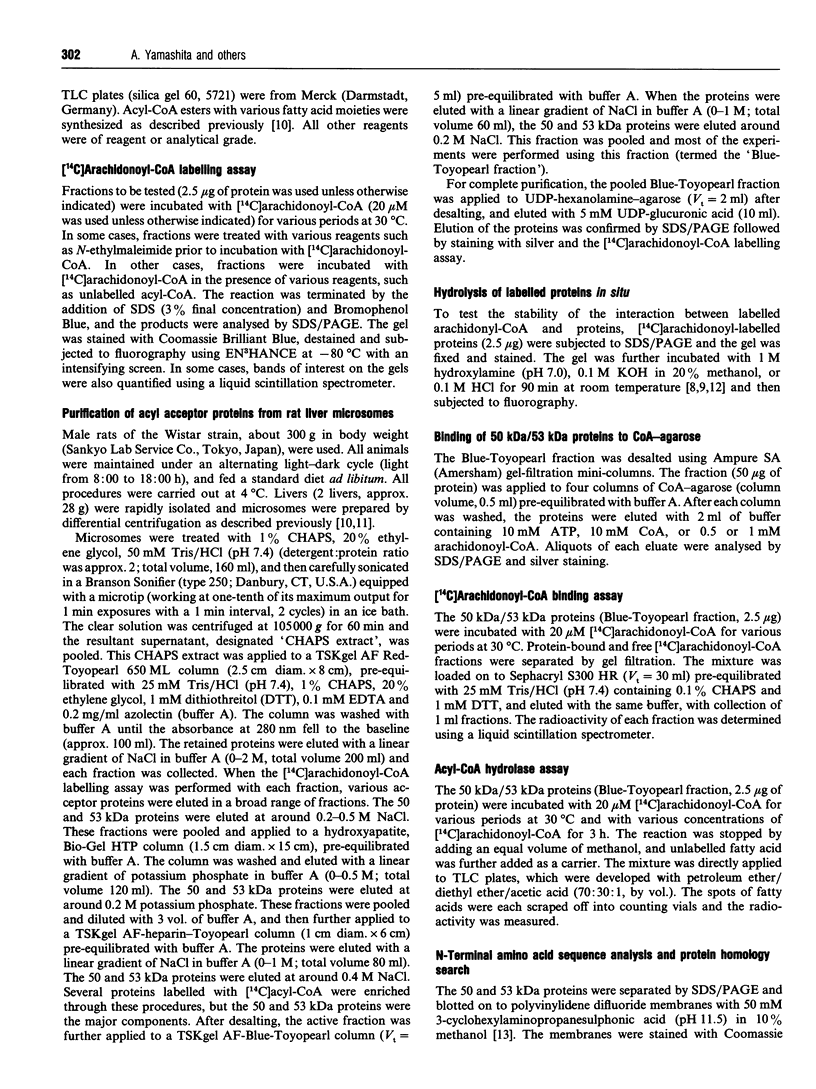
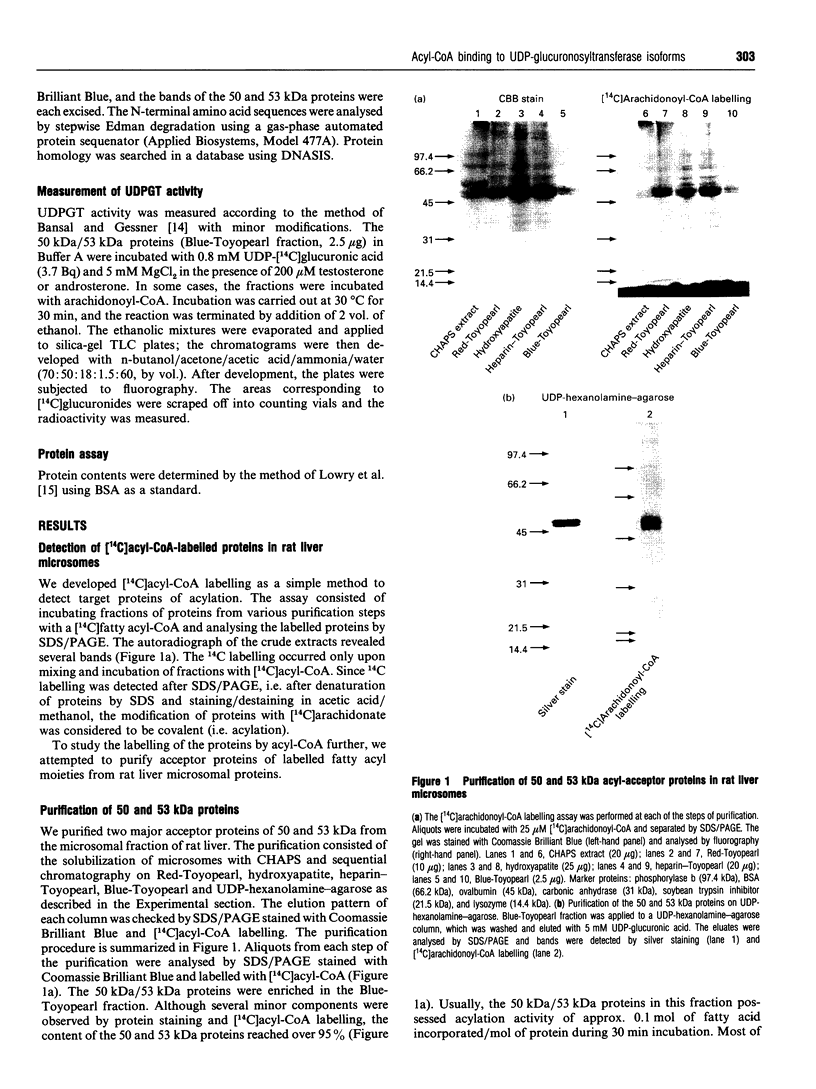
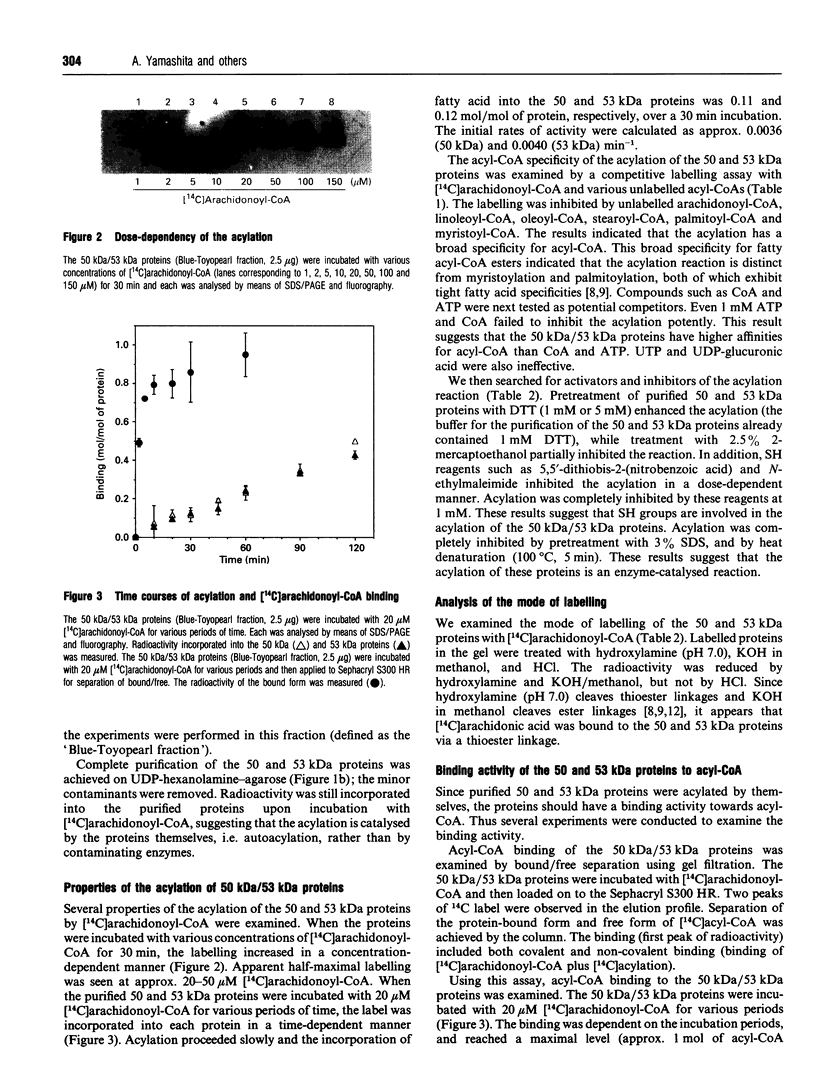
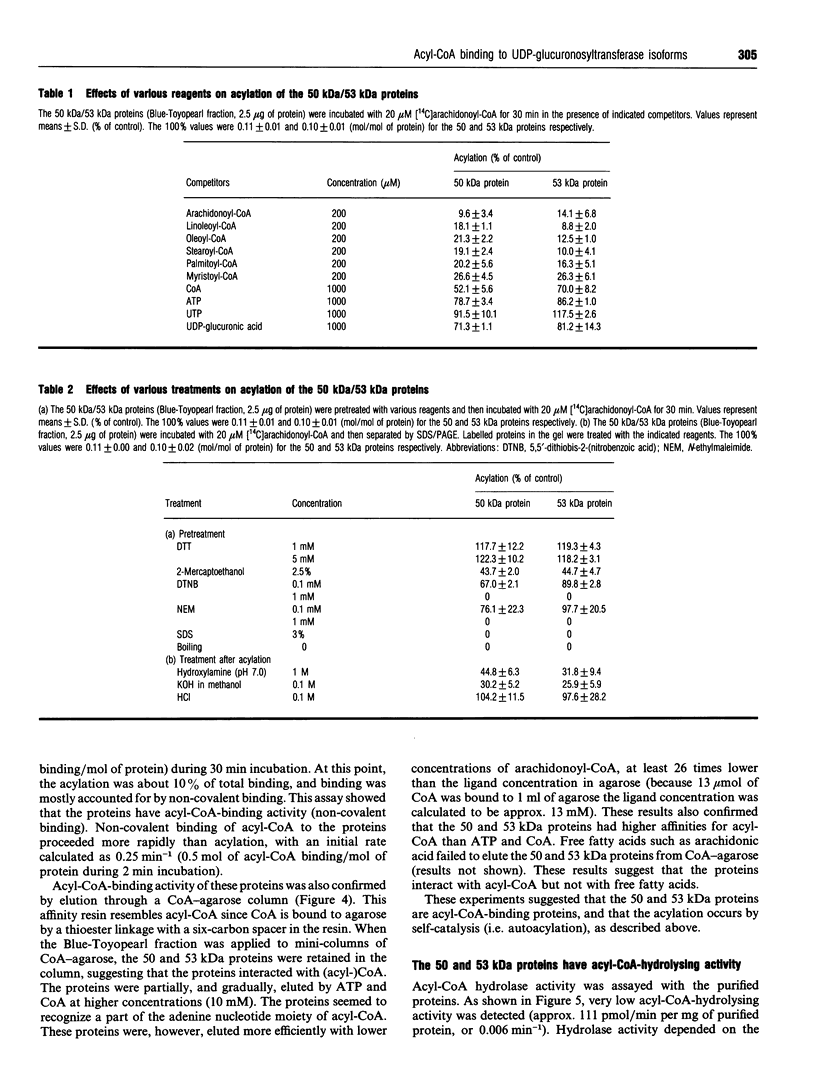
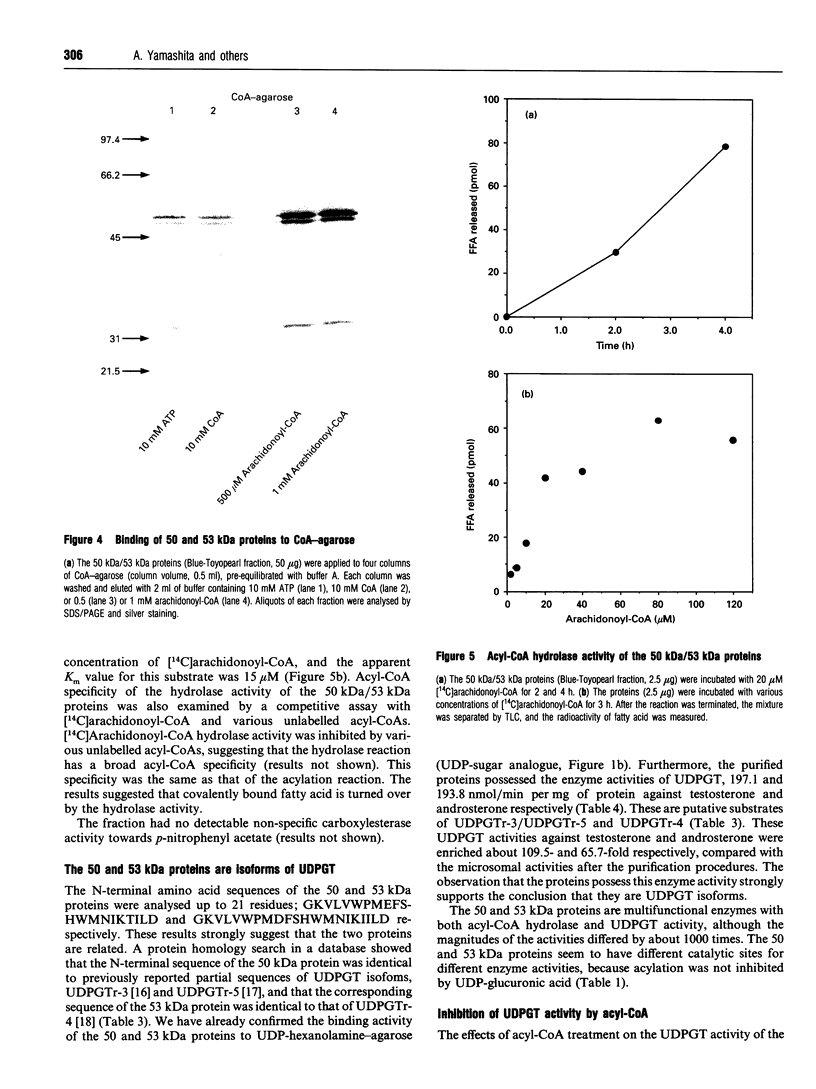
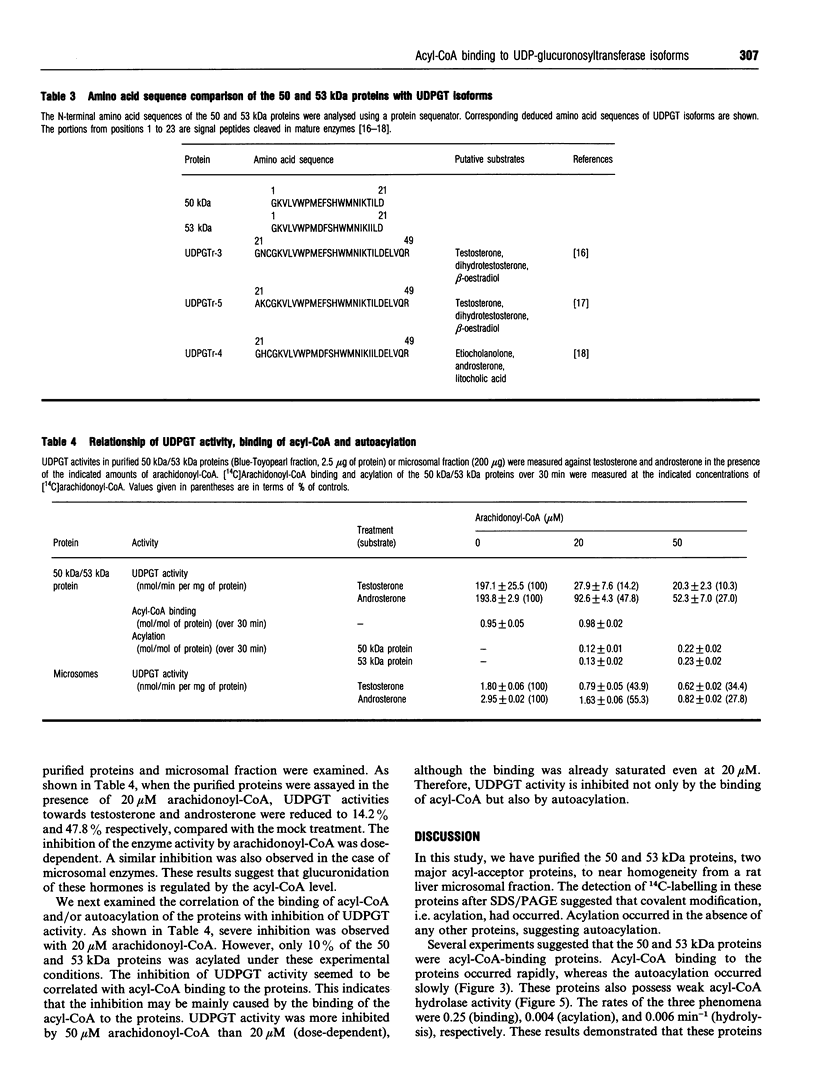
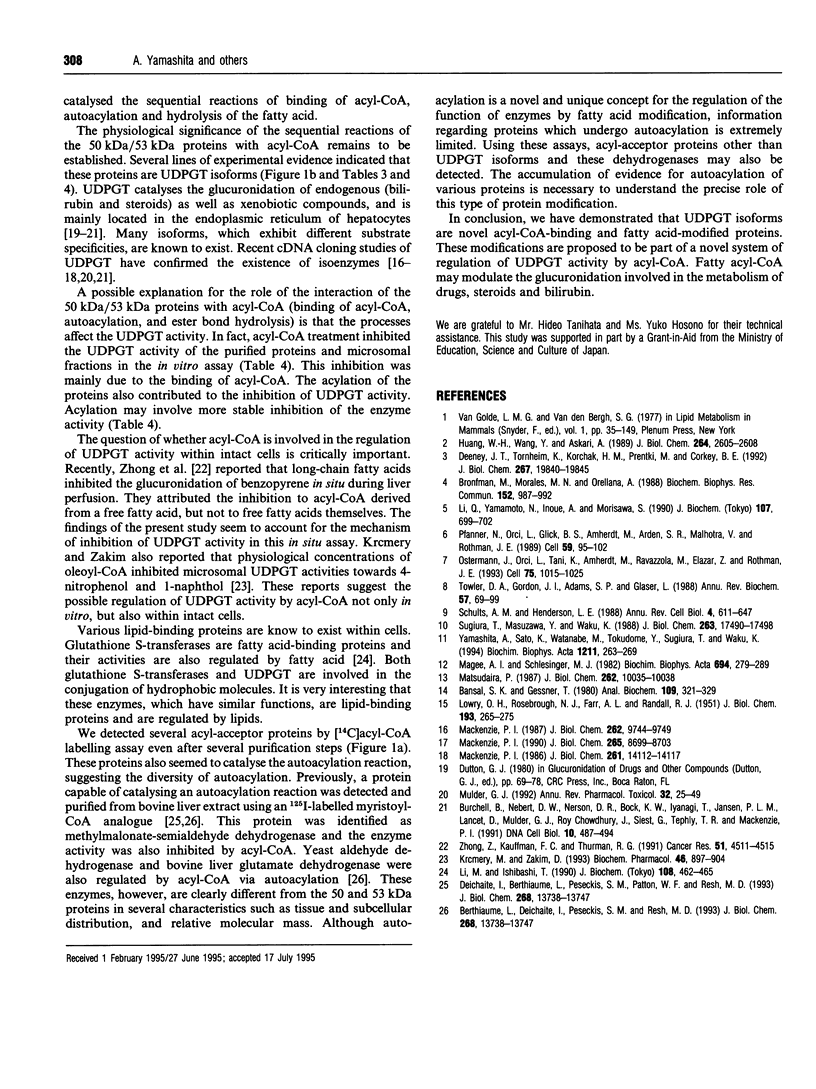
Images in this article
Selected References
These references are in PubMed. This may not be the complete list of references from this article.
- Bansal S. K., Gessner T. A unified method for the assay of uridine diphosphoglucuronyltransferase activities toward various aglycones using uridine diphospho[U-14C]glucuronic acid. Anal Biochem. 1980 Dec;109(2):321–329. doi: 10.1016/0003-2697(80)90655-7. [DOI] [PubMed] [Google Scholar]
- Bronfman M., Morales M. N., Orellana A. Diacylglycerol activation of protein kinase C is modulated by long-chain acyl-CoA. Biochem Biophys Res Commun. 1988 May 16;152(3):987–992. doi: 10.1016/s0006-291x(88)80381-4. [DOI] [PubMed] [Google Scholar]
- Burchell B., Nebert D. W., Nelson D. R., Bock K. W., Iyanagi T., Jansen P. L., Lancet D., Mulder G. J., Chowdhury J. R., Siest G. The UDP glucuronosyltransferase gene superfamily: suggested nomenclature based on evolutionary divergence. DNA Cell Biol. 1991 Sep;10(7):487–494. doi: 10.1089/dna.1991.10.487. [DOI] [PubMed] [Google Scholar]
- Deeney J. T., Tornheim K., Korchak H. M., Prentki M., Corkey B. E. Acyl-CoA esters modulate intracellular Ca2+ handling by permeabilized clonal pancreatic beta-cells. J Biol Chem. 1992 Oct 5;267(28):19840–19845. [PubMed] [Google Scholar]
- Deichaite I., Berthiaume L., Peseckis S. M., Patton W. F., Resh M. D. Novel use of an iodo-myristyl-CoA analog identifies a semialdehyde dehydrogenase in bovine liver. J Biol Chem. 1993 Jun 25;268(18):13738–13747. [PubMed] [Google Scholar]
- Huang W. H., Wang Y., Askari A. Mechanism of the control of (Na+ + K+)-ATPase by long-chain acyl coenzyme A. J Biol Chem. 1989 Feb 15;264(5):2605–2608. [PubMed] [Google Scholar]
- Krcmery M., Zakim D. Effects of oleoyl-CoA on the activity and functional state of UDP-glucuronosyltransferase. Biochem Pharmacol. 1993 Sep 1;46(5):897–904. doi: 10.1016/0006-2952(93)90500-v. [DOI] [PubMed] [Google Scholar]
- LOWRY O. H., ROSEBROUGH N. J., FARR A. L., RANDALL R. J. Protein measurement with the Folin phenol reagent. J Biol Chem. 1951 Nov;193(1):265–275. [PubMed] [Google Scholar]
- Li M., Ishibashi T. Evidence for identifying fatty acids in rat liver glutathione S-transferase and its possible involvement in the secondary structure. J Biochem. 1990 Sep;108(3):462–465. doi: 10.1093/oxfordjournals.jbchem.a123222. [DOI] [PubMed] [Google Scholar]
- Li Q. L., Yamamoto N., Inoue A., Morisawa S. Fatty acyl-CoAs are potent inhibitors of the nuclear thyroid hormone receptor in vitro. J Biochem. 1990 May;107(5):699–702. doi: 10.1093/oxfordjournals.jbchem.a123111. [DOI] [PubMed] [Google Scholar]
- Mackenzie P. I. Rat liver UDP-glucuronosyltransferase. Identification of cDNAs encoding two enzymes which glucuronidate testosterone, dihydrotestosterone, and beta-estradiol. J Biol Chem. 1987 Jul 15;262(20):9744–9749. [PubMed] [Google Scholar]
- Mackenzie P. I. Rat liver UDP-glucuronosyltransferase. cDNA sequence and expression of a form glucuronidating 3-hydroxyandrogens. J Biol Chem. 1986 Oct 25;261(30):14112–14117. [PubMed] [Google Scholar]
- Mackenzie P. I. The cDNA sequence and expression of a variant 17 beta-hydroxysteroid UDP-glucuronosyltransferase. J Biol Chem. 1990 May 25;265(15):8699–8703. [PubMed] [Google Scholar]
- Magee A. I., Schlesinger M. J. Fatty acid acylation of eucaryotic cell membrane proteins. Biochim Biophys Acta. 1982 Nov 30;694(3):279–289. doi: 10.1016/0304-4157(82)90008-9. [DOI] [PMC free article] [PubMed] [Google Scholar]
- Matsudaira P. Sequence from picomole quantities of proteins electroblotted onto polyvinylidene difluoride membranes. J Biol Chem. 1987 Jul 25;262(21):10035–10038. [PubMed] [Google Scholar]
- Mulder G. J. Glucuronidation and its role in regulation of biological activity of drugs. Annu Rev Pharmacol Toxicol. 1992;32:25–49. doi: 10.1146/annurev.pa.32.040192.000325. [DOI] [PubMed] [Google Scholar]
- Ostermann J., Orci L., Tani K., Amherdt M., Ravazzola M., Elazar Z., Rothman J. E. Stepwise assembly of functionally active transport vesicles. Cell. 1993 Dec 3;75(5):1015–1025. doi: 10.1016/0092-8674(93)90545-2. [DOI] [PubMed] [Google Scholar]
- Pfanner N., Orci L., Glick B. S., Amherdt M., Arden S. R., Malhotra V., Rothman J. E. Fatty acyl-coenzyme A is required for budding of transport vesicles from Golgi cisternae. Cell. 1989 Oct 6;59(1):95–102. doi: 10.1016/0092-8674(89)90872-6. [DOI] [PubMed] [Google Scholar]
- Schultz A. M., Henderson L. E., Oroszlan S. Fatty acylation of proteins. Annu Rev Cell Biol. 1988;4:611–647. doi: 10.1146/annurev.cb.04.110188.003143. [DOI] [PubMed] [Google Scholar]
- Sugiura T., Masuzawa Y., Waku K. Coenzyme A-dependent transacylation system in rabbit liver microsomes. J Biol Chem. 1988 Nov 25;263(33):17490–17498. [PubMed] [Google Scholar]
- Towler D. A., Gordon J. I., Adams S. P., Glaser L. The biology and enzymology of eukaryotic protein acylation. Annu Rev Biochem. 1988;57:69–99. doi: 10.1146/annurev.bi.57.070188.000441. [DOI] [PubMed] [Google Scholar]
- Yamashita A., Sato K., Watanabe M., Tokudome Y., Sugiura T., Waku K. Induction of coenzyme A-dependent transacylation activity in rat liver microsomes by administration of clofibrate. Biochim Biophys Acta. 1994 Mar 24;1211(3):263–269. doi: 10.1016/0005-2760(94)90149-x. [DOI] [PubMed] [Google Scholar]
- Zhong Z., Kauffman F. C., Thurman R. G. Inhibition of glucuronidation of benzo(a)pyrene phenols by long-chain fatty acids. Cancer Res. 1991 Sep 1;51(17):4511–4515. [PubMed] [Google Scholar]