Abstract
IR spectroscopy has been widely applied in the study of photo-activated biological processes such as photosynthesis, but has not been applied to the study of reacting systems which require rapid mixing of aqueous solutions. This has been due in part to the high pressure needed to make an aqueous solution flow rapidly through the 50 microns optical pathlength between the plates in an IR cuvette suitable for use with 2H2O and the high viscosity of the concentrated protein solutions required to generate measurable IR signals. An apparatus, based largely on conventional stopped-flow technology, is described which achieves mixing well within the time-resolved performance (approximately 40 ms) of modern Fourier-transform IR (FTIR) spectrometers, since the dead time of the mixing device is approximately 15 ms. It has proved possible to achieve efficient mixing by using a simple six-jet mixing device. This is probably at least in part because of the high back pressure which develops when aqueous fluid is passed rapidly through the short pathlength of the cuvette and which promotes turbulent flow. Several examples of measurements of the deacylation of acylchymotrypsins are provided which demonstrate the operation of the apparatus in conjunction with a spectrometer capable of scanning at four scans/s. For cinnamoyl-chymotrypsin, isotope-edited spectra have been obtained which show somewhat lower resolution than is achieved by conventional scanning methods, since some smoothing has to be applied to the spectra. Difference spectra of the acylation of chymotrypsin by glycylglycine p-nitrophenyl ester have been obtained by averaging ten stopped-flow shots and show good signal-to-noise ratio without smoothing. It is predicted that this apparatus is likely to find a variety of applications in the study of enzyme-catalysed reactions, since the spectra are relatively rich in structural information, and isotope editing greatly enhances the interpretability of the spectra.
Full text
PDF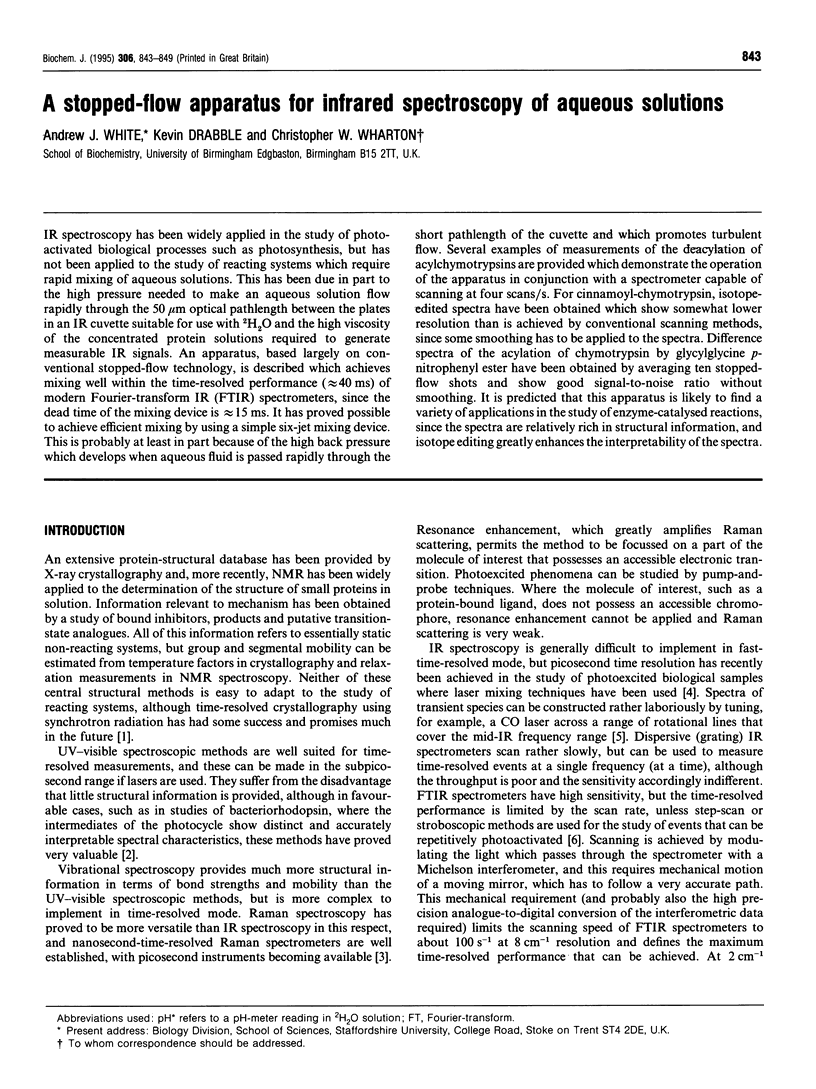
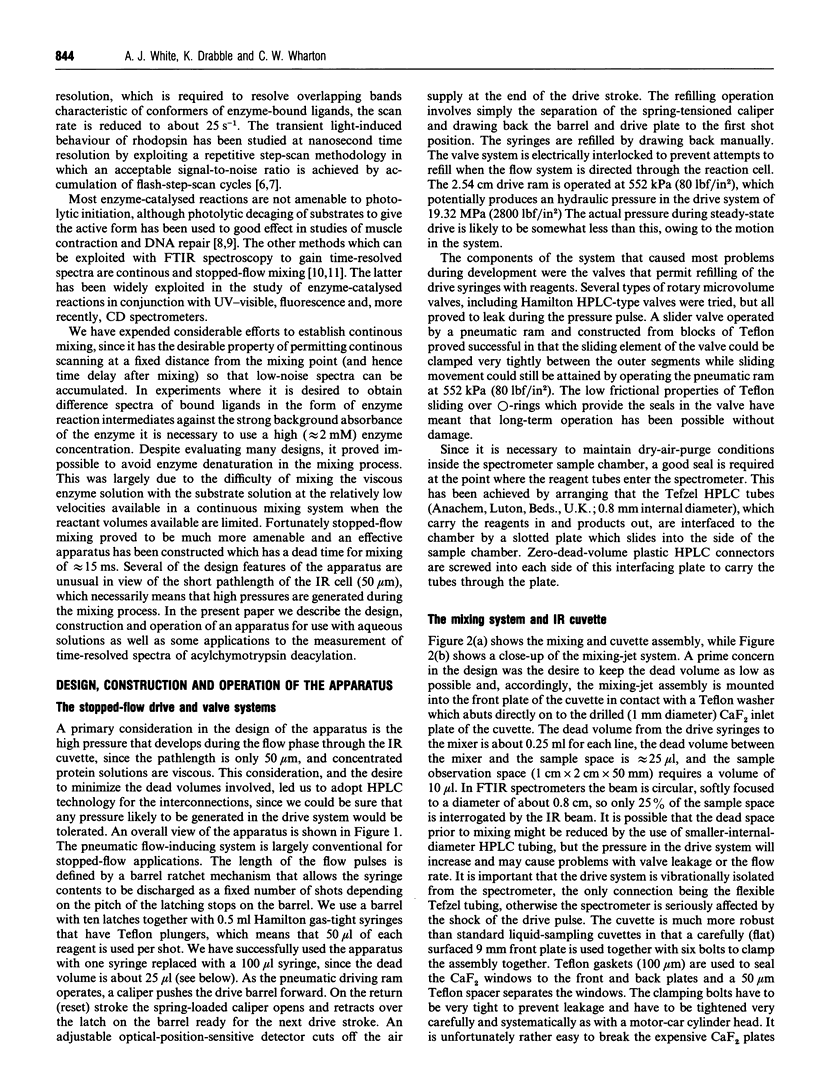
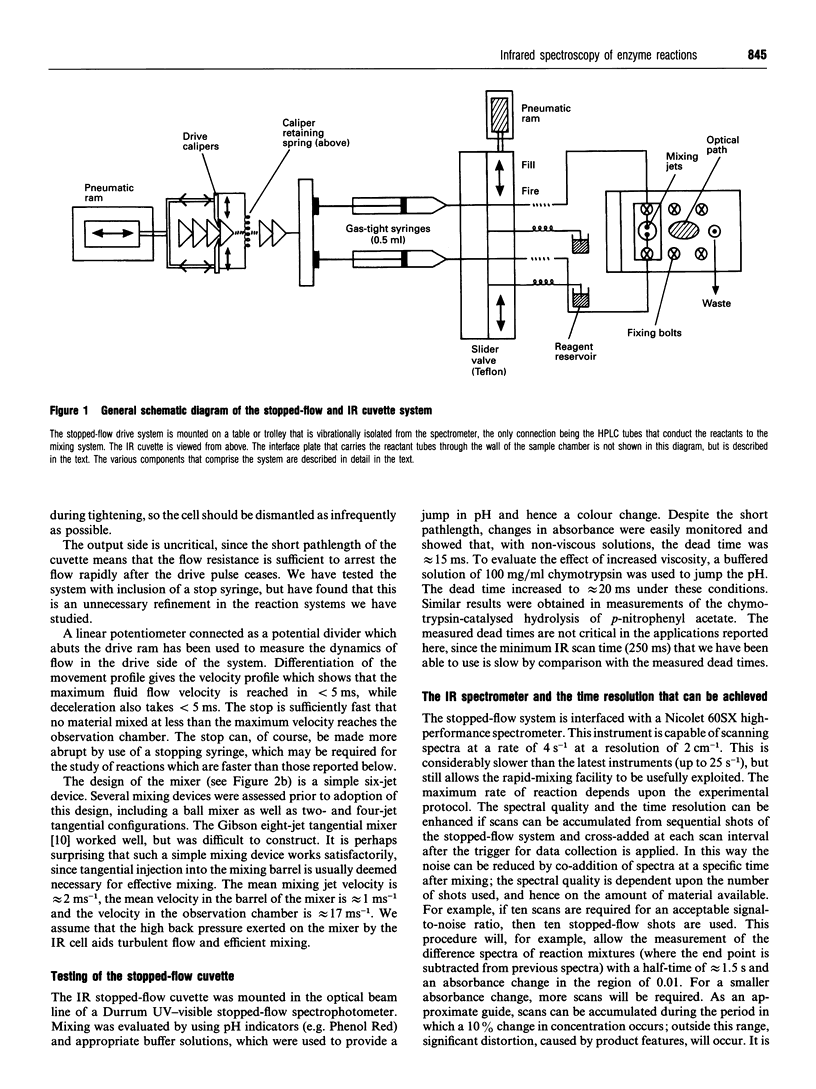
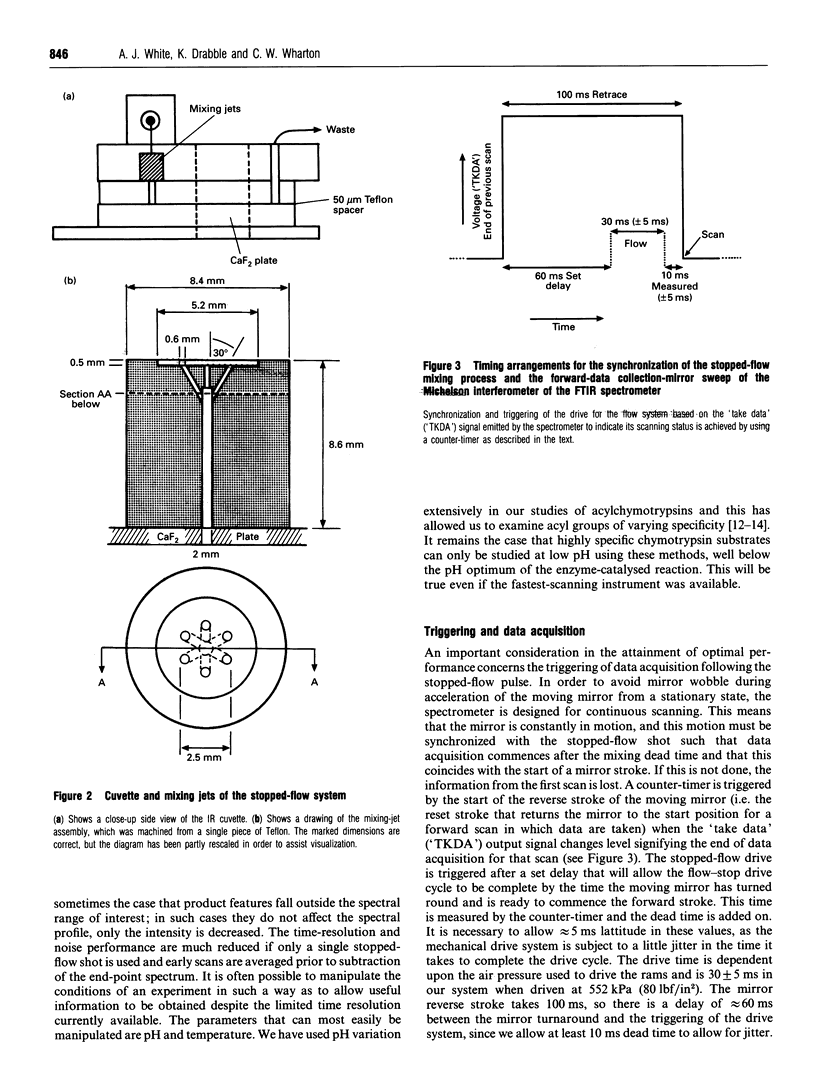
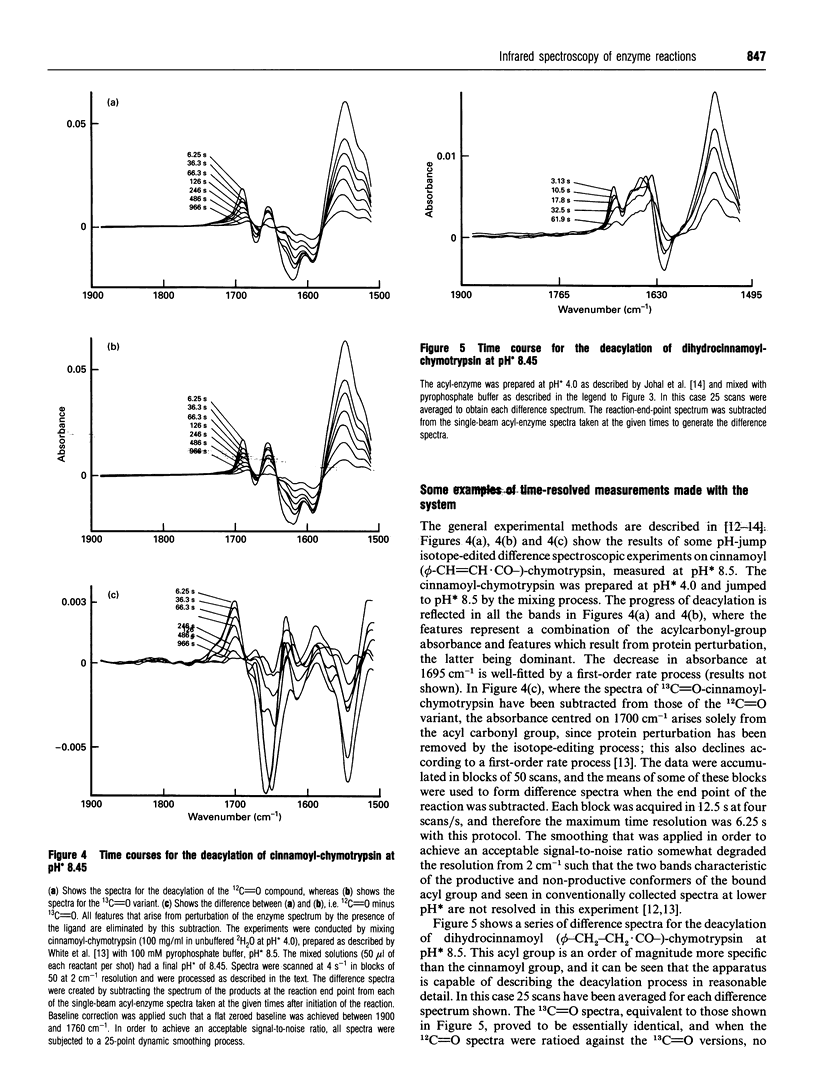
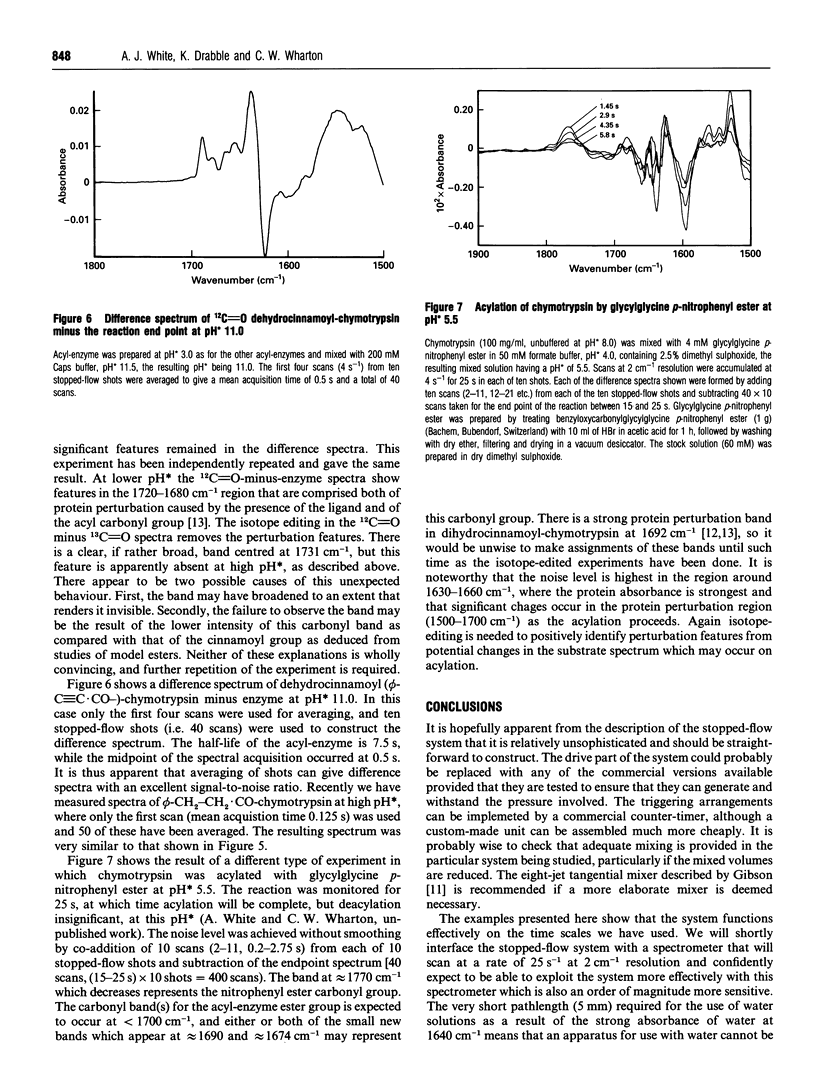
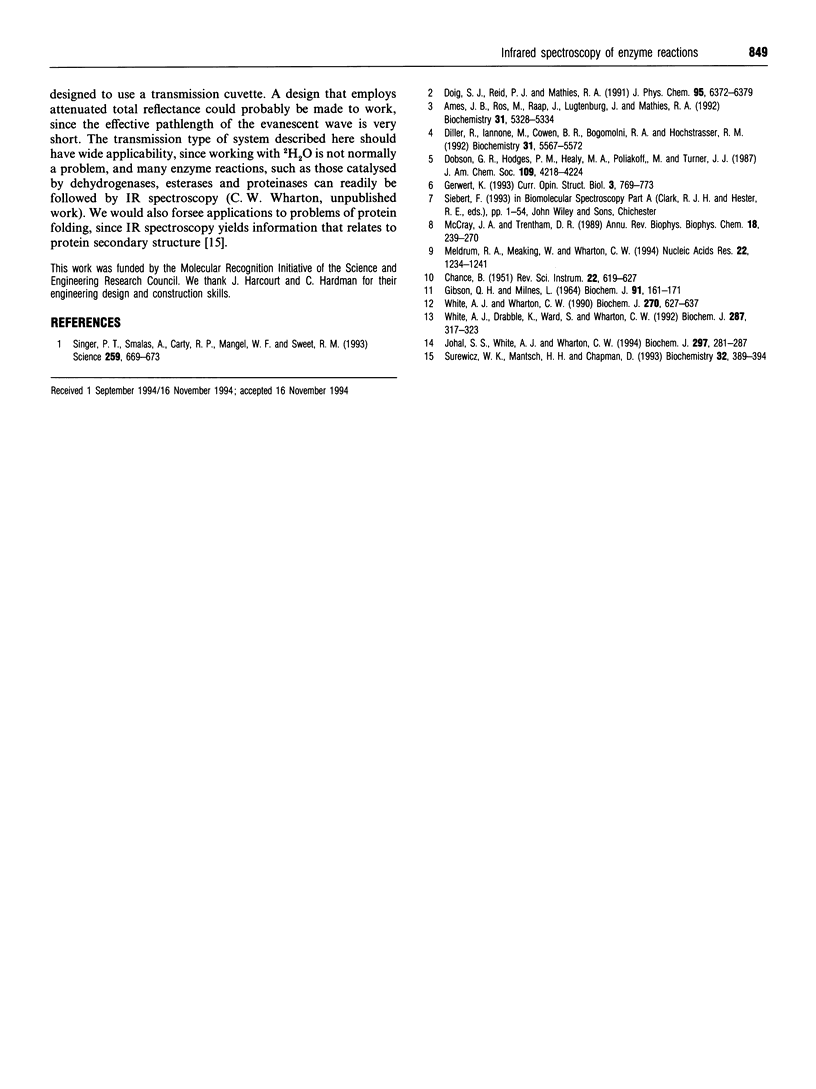
Selected References
These references are in PubMed. This may not be the complete list of references from this article.
- Ames J. B., Ros M., Raap J., Lugtenburg J., Mathies R. A. Time-resolved ultraviolet resonance Raman studies of protein structure: application to bacteriorhodopsin. Biochemistry. 1992 Jun 16;31(23):5328–5334. doi: 10.1021/bi00138a012. [DOI] [PubMed] [Google Scholar]
- Diller R., Iannone M., Cowen B. R., Maiti S., Bogomolni R. A., Hochstrasser R. M. Picosecond dynamics of bacteriorhodopsin, probed by time-resolved infrared spectroscopy. Biochemistry. 1992 Jun 23;31(24):5567–5572. doi: 10.1021/bi00139a020. [DOI] [PubMed] [Google Scholar]
- Gibson Q. H., Milnes L. Apparatus for rapid and sensitive spectrophotometry. Biochem J. 1964 Apr;91(1):161–171. doi: 10.1042/bj0910161. [DOI] [PMC free article] [PubMed] [Google Scholar]
- Johal S. S., White A. J., Wharton C. W. Effect of specificity on ligand conformation in acyl-chymotrypsins. Biochem J. 1994 Jan 15;297(Pt 2):281–287. doi: 10.1042/bj2970281. [DOI] [PMC free article] [PubMed] [Google Scholar]
- McCray J. A., Trentham D. R. Properties and uses of photoreactive caged compounds. Annu Rev Biophys Biophys Chem. 1989;18:239–270. doi: 10.1146/annurev.bb.18.060189.001323. [DOI] [PubMed] [Google Scholar]
- Meldrum R. A., Meaking W. S., Wharton C. W. The kinetics and mechanism of repair of UV induced DNA damage in mammalian cells. The use of 'caged' nucleotides and electroporation to study short time course events in DNA repair. Nucleic Acids Res. 1994 Apr 11;22(7):1234–1241. doi: 10.1093/nar/22.7.1234. [DOI] [PMC free article] [PubMed] [Google Scholar]
- Singer P. T., Smalås A., Carty R. P., Mangel W. F., Sweet R. M. The hydrolytic water molecule in trypsin, revealed by time-resolved Laue crystallography. Science. 1993 Jan 29;259(5095):669–673. doi: 10.1126/science.8430314. [DOI] [PubMed] [Google Scholar]
- Surewicz W. K., Mantsch H. H., Chapman D. Determination of protein secondary structure by Fourier transform infrared spectroscopy: a critical assessment. Biochemistry. 1993 Jan 19;32(2):389–394. doi: 10.1021/bi00053a001. [DOI] [PubMed] [Google Scholar]
- White A. J., Wharton C. W. Hydrogen-bonding in enzyme catalysis. Fourier-transform infrared detection of ground-state electronic strain in acyl-chymotrypsins and analysis of the kinetic consequences. Biochem J. 1990 Sep 15;270(3):627–637. doi: 10.1042/bj2700627. [DOI] [PMC free article] [PubMed] [Google Scholar]