Abstract
Most of the increased protein degradation in muscle atrophy caused by starvation and denervation is due to activation of a non-lysosomal ATP-dependent proteolytic process. To determine whether expression of the ubiquitin-proteasome-dependent pathway is activated in atrophying muscles, we measured the levels of mRNA for ubiquitin (Ub) and proteasome subunits, and Ub content. After rats had been deprived of food for 1 or 2 days, the concentration of the two polyubiquitin (polyUb) transcripts increased 2-4-fold in the pale extensor digitorum longus muscle and 1-2.5-fold in the red soleus, whereas total muscle RNA and total mRNA content fell by 50%. After denervation of the soleus, there was a progressive 2-3-fold increase in polyUb mRNA for 1-3 days, whereas total RNA content fell. On starvation or denervation, Ub concentration in the muscles also rose by 60-90%. During starvation, polyUb mRNA levels also increased in heart, but not in liver, kidney, spleen, fat, brain or testes. Although the polyUb gene is a heat-shock gene that is induced in muscles under certain stressful conditions, the muscles of starving rats or after denervation did not express other heat-shock genes. On starvation or denervation, mRNA for several proteasome subunits (C-1, C-3, C-5, C-8 and C-9) also increased 2-4-fold in the atrophying muscles. When the food-deprived animals were re-fed, levels of Ub and proteasome mRNA in their muscles returned to control values within 1 day. In contrast, no change occurred in the levels of muscle mRNAs encoding cathepsin L, cathepsin D and calpain 1 on denervation or food deprivation. Thus polyUb and proteasome mRNAs increased in atrophying muscles in co-ordination with activation of the ATP-dependent proteolytic process.
Full text
PDF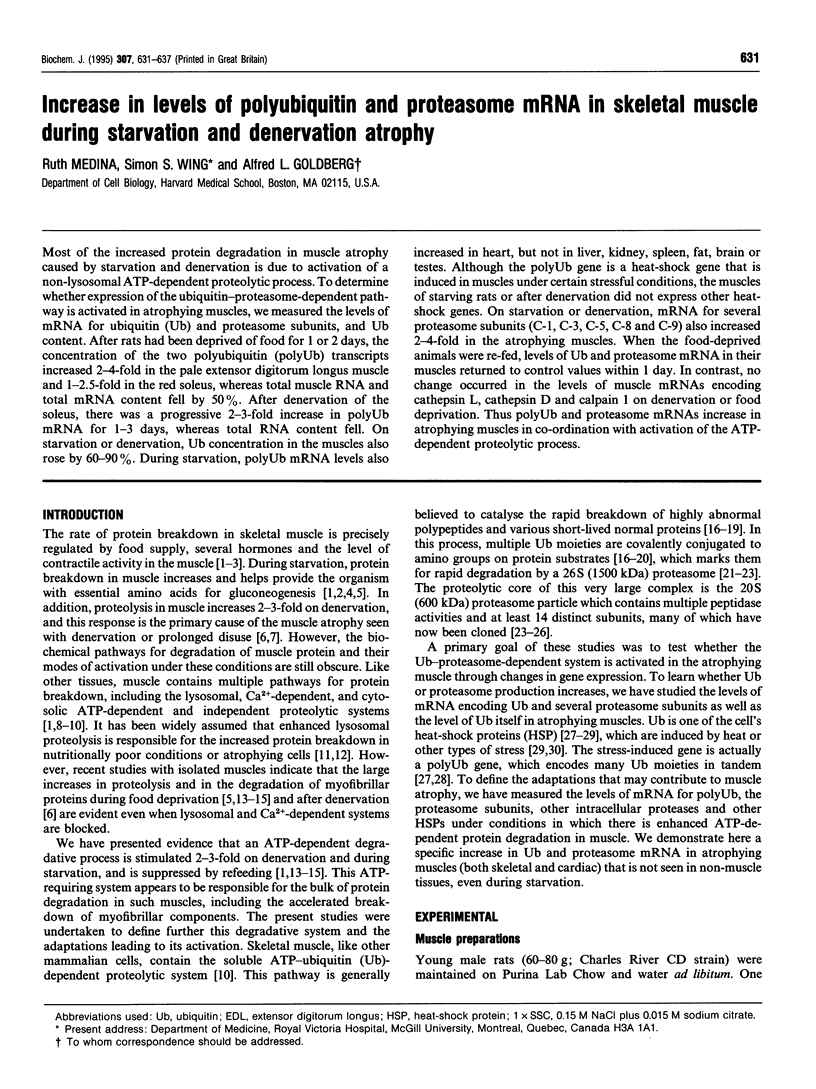
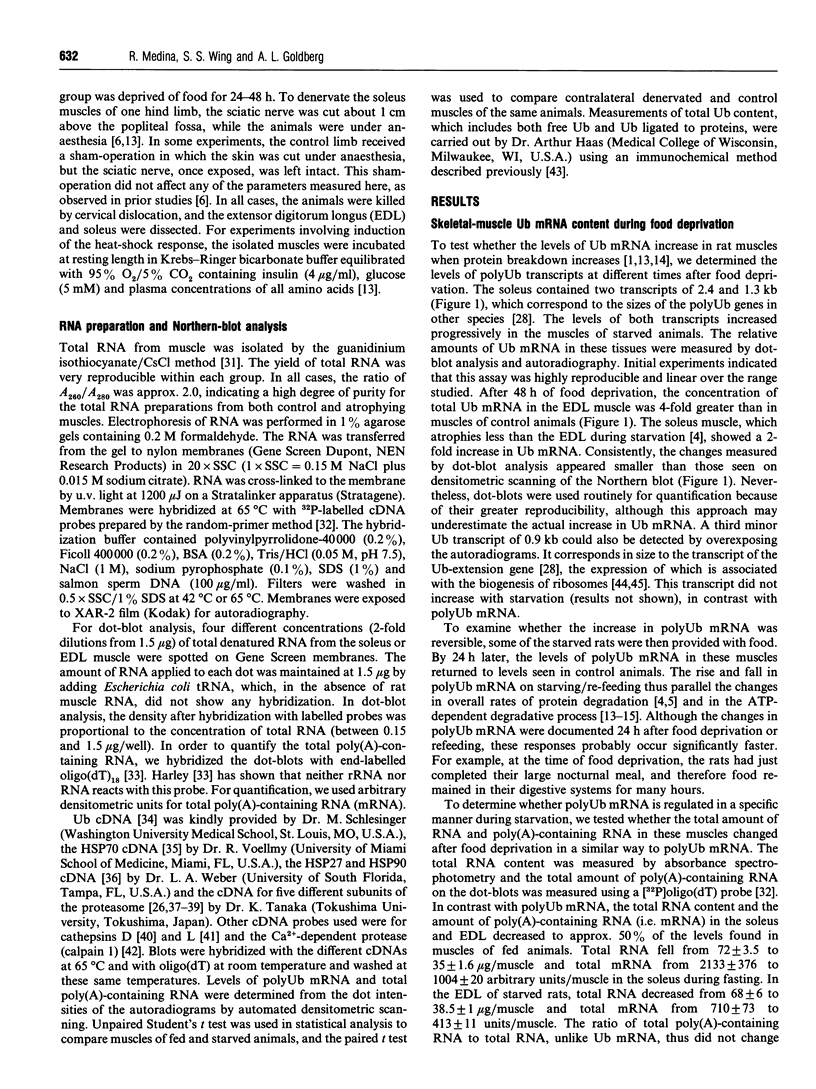
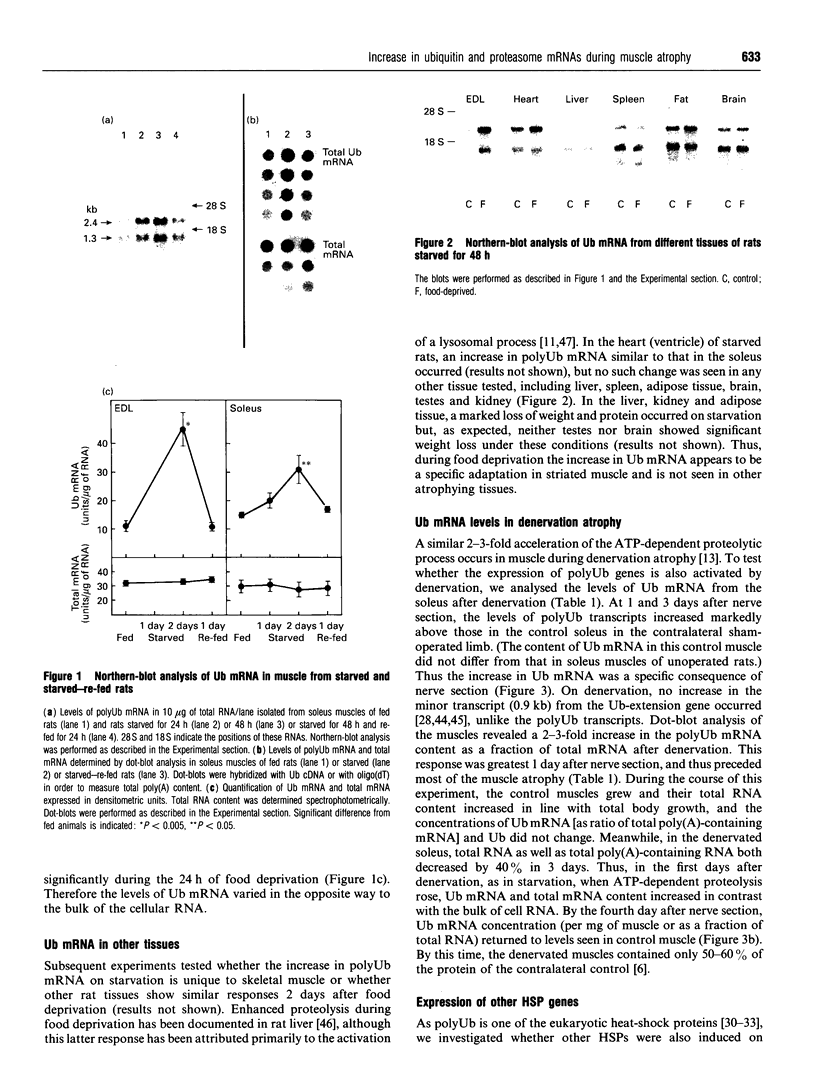
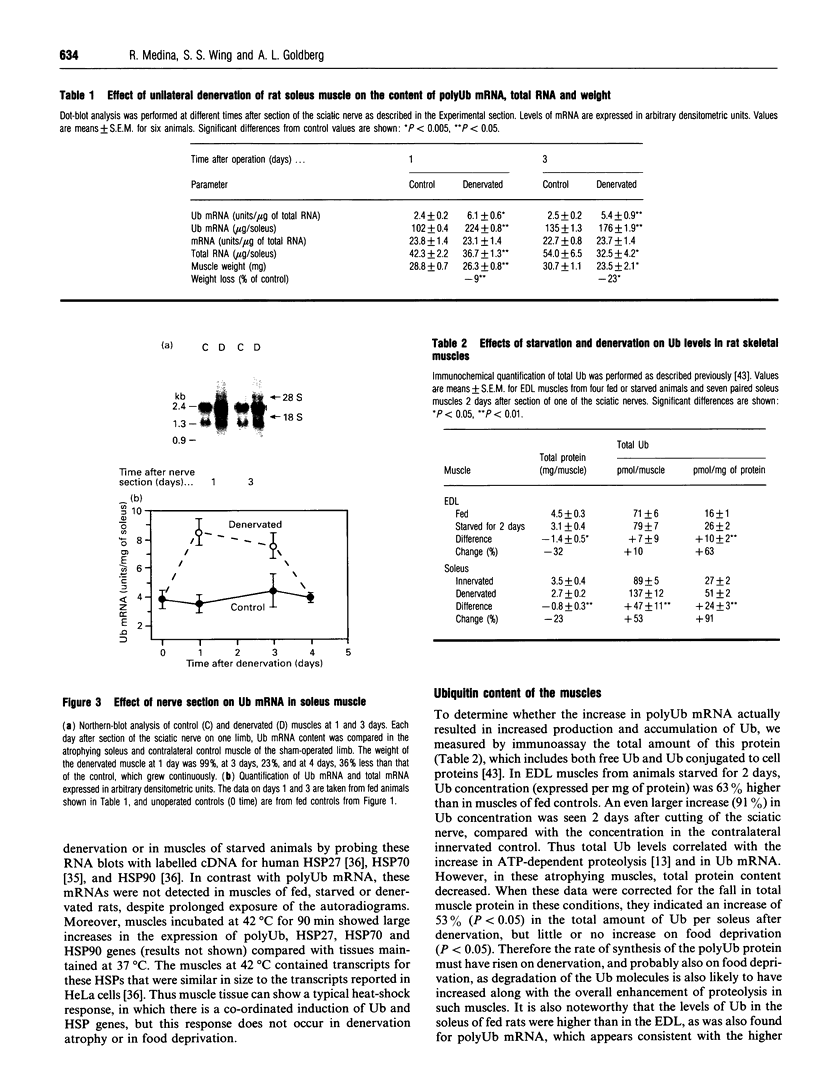
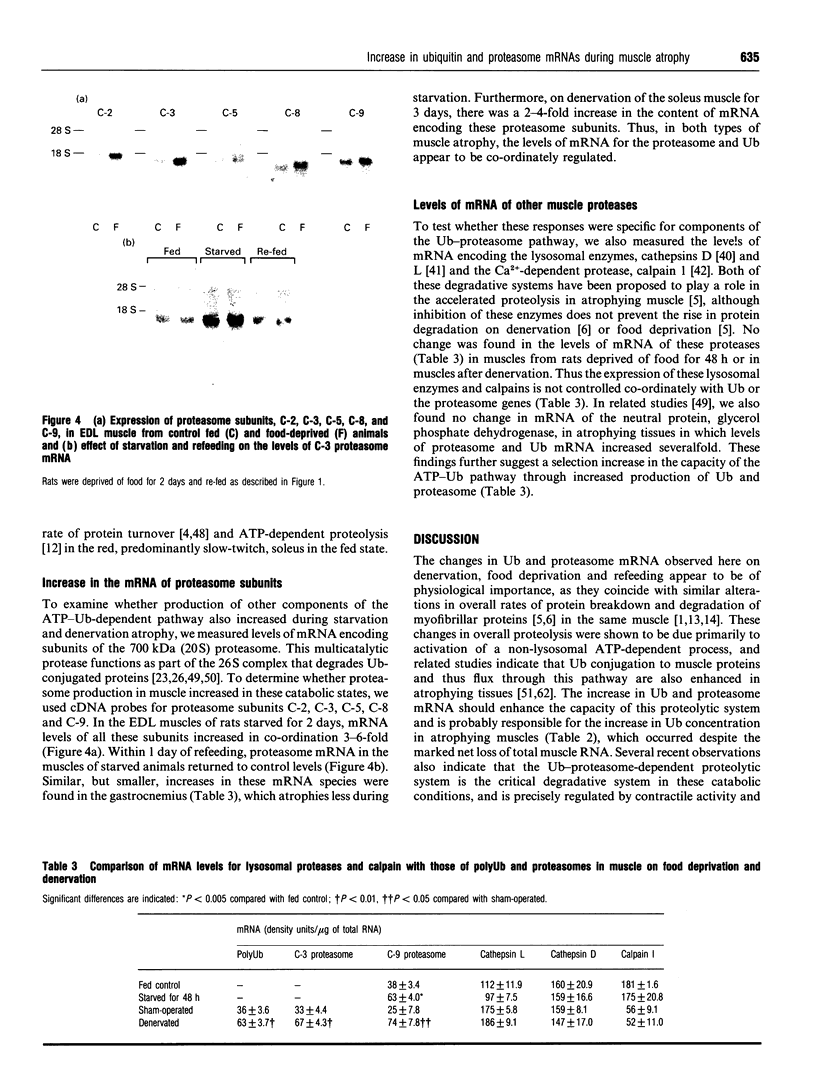
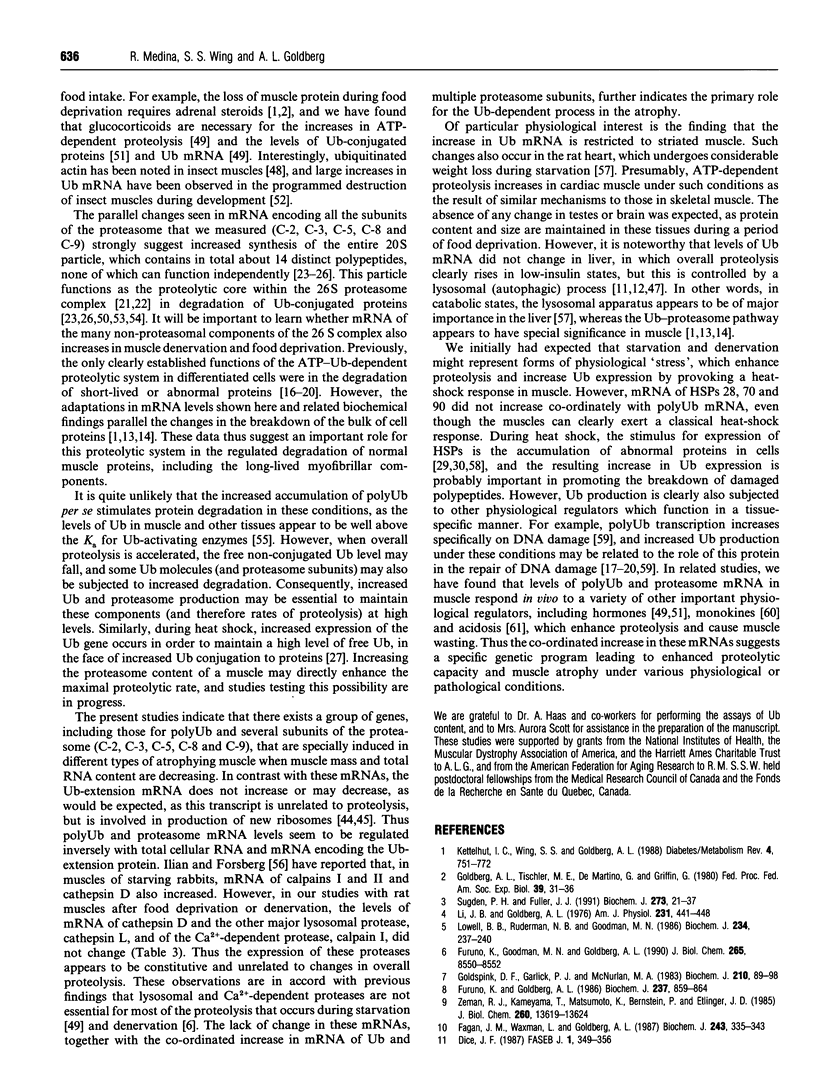
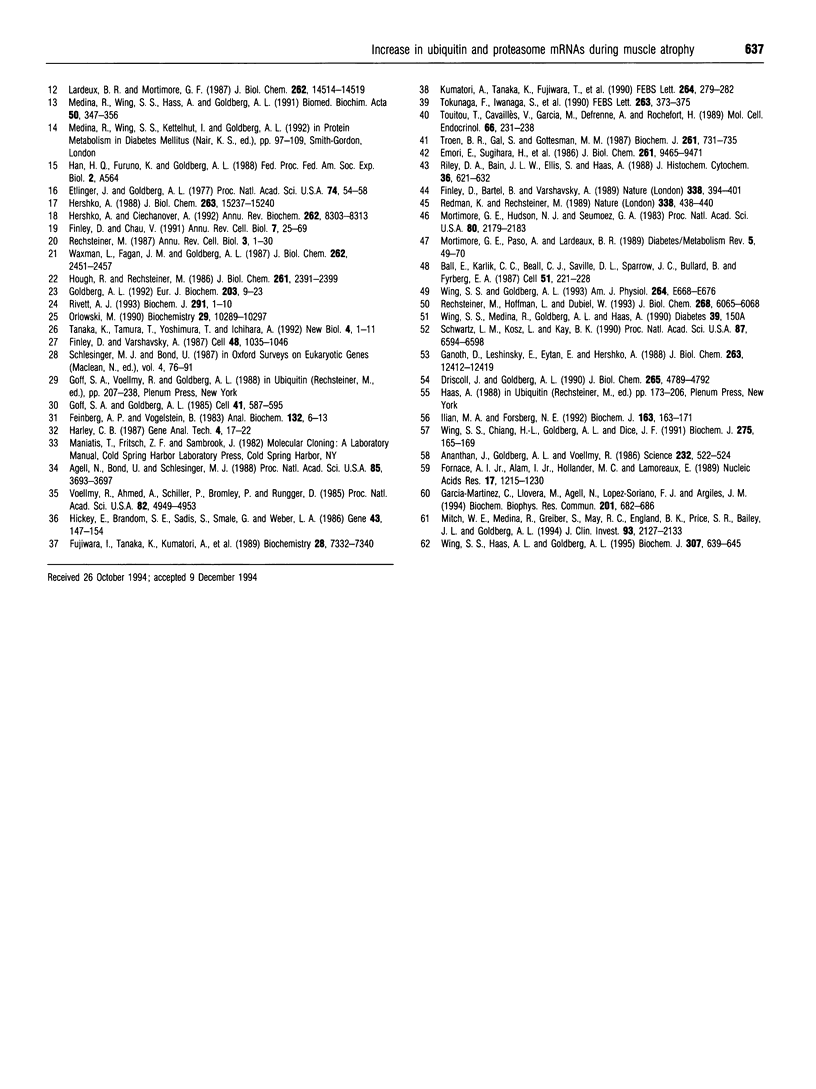
Images in this article
Selected References
These references are in PubMed. This may not be the complete list of references from this article.
- Agell N., Bond U., Schlesinger M. J. In vitro proteolytic processing of a diubiquitin and a truncated diubiquitin formed from in vitro-generated mRNAs. Proc Natl Acad Sci U S A. 1988 Jun;85(11):3693–3697. doi: 10.1073/pnas.85.11.3693. [DOI] [PMC free article] [PubMed] [Google Scholar]
- Ananthan J., Goldberg A. L., Voellmy R. Abnormal proteins serve as eukaryotic stress signals and trigger the activation of heat shock genes. Science. 1986 Apr 25;232(4749):522–524. doi: 10.1126/science.3083508. [DOI] [PubMed] [Google Scholar]
- Ball E., Karlik C. C., Beall C. J., Saville D. L., Sparrow J. C., Bullard B., Fyrberg E. A. Arthrin, a myofibrillar protein of insect flight muscle, is an actin-ubiquitin conjugate. Cell. 1987 Oct 23;51(2):221–228. doi: 10.1016/0092-8674(87)90149-8. [DOI] [PubMed] [Google Scholar]
- Catterall W. A. A Nobel Prize for single channel recording. New Biol. 1992 Jan;4(1):1–3. [PubMed] [Google Scholar]
- Dice J. F. Molecular determinants of protein half-lives in eukaryotic cells. FASEB J. 1987 Nov;1(5):349–357. doi: 10.1096/fasebj.1.5.2824267. [DOI] [PubMed] [Google Scholar]
- Driscoll J., Goldberg A. L. The proteasome (multicatalytic protease) is a component of the 1500-kDa proteolytic complex which degrades ubiquitin-conjugated proteins. J Biol Chem. 1990 Mar 25;265(9):4789–4792. [PubMed] [Google Scholar]
- Emori Y., Kawasaki H., Sugihara H., Imajoh S., Kawashima S., Suzuki K. Isolation and sequence analyses of cDNA clones for the large subunits of two isozymes of rabbit calcium-dependent protease. J Biol Chem. 1986 Jul 15;261(20):9465–9471. [PubMed] [Google Scholar]
- Etlinger J. D., Goldberg A. L. A soluble ATP-dependent proteolytic system responsible for the degradation of abnormal proteins in reticulocytes. Proc Natl Acad Sci U S A. 1977 Jan;74(1):54–58. doi: 10.1073/pnas.74.1.54. [DOI] [PMC free article] [PubMed] [Google Scholar]
- Fagan J. M., Waxman L., Goldberg A. L. Skeletal muscle and liver contain a soluble ATP + ubiquitin-dependent proteolytic system. Biochem J. 1987 Apr 15;243(2):335–343. doi: 10.1042/bj2430335. [DOI] [PMC free article] [PubMed] [Google Scholar]
- Feinberg A. P., Vogelstein B. A technique for radiolabeling DNA restriction endonuclease fragments to high specific activity. Anal Biochem. 1983 Jul 1;132(1):6–13. doi: 10.1016/0003-2697(83)90418-9. [DOI] [PubMed] [Google Scholar]
- Finley D., Bartel B., Varshavsky A. The tails of ubiquitin precursors are ribosomal proteins whose fusion to ubiquitin facilitates ribosome biogenesis. Nature. 1989 Mar 30;338(6214):394–401. doi: 10.1038/338394a0. [DOI] [PubMed] [Google Scholar]
- Finley D., Chau V. Ubiquitination. Annu Rev Cell Biol. 1991;7:25–69. doi: 10.1146/annurev.cb.07.110191.000325. [DOI] [PubMed] [Google Scholar]
- Finley D., Ozkaynak E., Varshavsky A. The yeast polyubiquitin gene is essential for resistance to high temperatures, starvation, and other stresses. Cell. 1987 Mar 27;48(6):1035–1046. doi: 10.1016/0092-8674(87)90711-2. [DOI] [PubMed] [Google Scholar]
- Fornace A. J., Jr, Alamo I., Jr, Hollander M. C., Lamoreaux E. Ubiquitin mRNA is a major stress-induced transcript in mammalian cells. Nucleic Acids Res. 1989 Feb 11;17(3):1215–1230. doi: 10.1093/nar/17.3.1215. [DOI] [PMC free article] [PubMed] [Google Scholar]
- Fujiwara T., Tanaka K., Kumatori A., Shin S., Yoshimura T., Ichihara A., Tokunaga F., Aruga R., Iwanaga S., Kakizuka A. Molecular cloning of cDNA for proteasomes (multicatalytic proteinase complexes) from rat liver: primary structure of the largest component (C2). Biochemistry. 1989 Sep 5;28(18):7332–7340. doi: 10.1021/bi00444a028. [DOI] [PubMed] [Google Scholar]
- Furuno K., Goldberg A. L. The activation of protein degradation in muscle by Ca2+ or muscle injury does not involve a lysosomal mechanism. Biochem J. 1986 Aug 1;237(3):859–864. doi: 10.1042/bj2370859. [DOI] [PMC free article] [PubMed] [Google Scholar]
- Furuno K., Goodman M. N., Goldberg A. L. Role of different proteolytic systems in the degradation of muscle proteins during denervation atrophy. J Biol Chem. 1990 May 25;265(15):8550–8557. [PubMed] [Google Scholar]
- Ganoth D., Leshinsky E., Eytan E., Hershko A. A multicomponent system that degrades proteins conjugated to ubiquitin. Resolution of factors and evidence for ATP-dependent complex formation. J Biol Chem. 1988 Sep 5;263(25):12412–12419. [PubMed] [Google Scholar]
- García-Martínez C., Llovera M., Agell N., López-Soriano F. J., Argilés J. M. Ubiquitin gene expression in skeletal muscle is increased by tumour necrosis factor-alpha. Biochem Biophys Res Commun. 1994 Jun 15;201(2):682–686. doi: 10.1006/bbrc.1994.1754. [DOI] [PubMed] [Google Scholar]
- Goff S. A., Goldberg A. L. Production of abnormal proteins in E. coli stimulates transcription of lon and other heat shock genes. Cell. 1985 Jun;41(2):587–595. doi: 10.1016/s0092-8674(85)80031-3. [DOI] [PubMed] [Google Scholar]
- Goldberg A. L. The mechanism and functions of ATP-dependent proteases in bacterial and animal cells. Eur J Biochem. 1992 Jan 15;203(1-2):9–23. doi: 10.1111/j.1432-1033.1992.tb19822.x. [DOI] [PubMed] [Google Scholar]
- Goldberg A. L., Tischler M., DeMartino G., Griffin G. Hormonal regulation of protein degradation and synthesis in skeletal muscle. Fed Proc. 1980 Jan;39(1):31–36. [PubMed] [Google Scholar]
- Goldspink D. F., Garlick P. J., McNurlan M. A. Protein turnover measured in vivo and in vitro in muscles undergoing compensatory growth and subsequent denervation atrophy. Biochem J. 1983 Jan 15;210(1):89–98. doi: 10.1042/bj2100089. [DOI] [PMC free article] [PubMed] [Google Scholar]
- Harley C. B. Hybridization of oligo(dT) to RNA on nitrocellulose. Gene Anal Tech. 1987 Mar-Apr;4(2):17–22. doi: 10.1016/0735-0651(87)90013-6. [DOI] [PubMed] [Google Scholar]
- Hershko A. Ubiquitin-mediated protein degradation. J Biol Chem. 1988 Oct 25;263(30):15237–15240. [PubMed] [Google Scholar]
- Hickey E., Brandon S. E., Sadis S., Smale G., Weber L. A. Molecular cloning of sequences encoding the human heat-shock proteins and their expression during hyperthermia. Gene. 1986;43(1-2):147–154. doi: 10.1016/0378-1119(86)90018-1. [DOI] [PubMed] [Google Scholar]
- Hough R., Rechsteiner M. Ubiquitin-lysozyme conjugates. Purification and susceptibility to proteolysis. J Biol Chem. 1986 Feb 15;261(5):2391–2399. [PubMed] [Google Scholar]
- Ilian M. A., Forsberg N. E. Gene expression of calpains and their specific endogenous inhibitor, calpastatin, in skeletal muscle of fed and fasted rabbits. Biochem J. 1992 Oct 1;287(Pt 1):163–171. doi: 10.1042/bj2870163. [DOI] [PMC free article] [PubMed] [Google Scholar]
- Kettelhut I. C., Wing S. S., Goldberg A. L. Endocrine regulation of protein breakdown in skeletal muscle. Diabetes Metab Rev. 1988 Dec;4(8):751–772. doi: 10.1002/dmr.5610040805. [DOI] [PubMed] [Google Scholar]
- Kumatori A., Tanaka K., Tamura T., Fujiwara T., Ichihara A., Tokunaga F., Onikura A., Iwanaga S. cDNA cloning and sequencing of component C9 of proteasomes from rat hepatoma cells. FEBS Lett. 1990 May 21;264(2):279–282. doi: 10.1016/0014-5793(90)80267-m. [DOI] [PubMed] [Google Scholar]
- Lardeux B. R., Mortimore G. E. Amino acid and hormonal control of macromolecular turnover in perfused rat liver. Evidence for selective autophagy. J Biol Chem. 1987 Oct 25;262(30):14514–14519. [PubMed] [Google Scholar]
- Li J. B., Goldberg A. L. Effects of food deprivation on protein synthesis and degradation in rat skeletal muscles. Am J Physiol. 1976 Aug;231(2):441–448. doi: 10.1152/ajplegacy.1976.231.2.441. [DOI] [PubMed] [Google Scholar]
- Lowell B. B., Ruderman N. B., Goodman M. N. Evidence that lysosomes are not involved in the degradation of myofibrillar proteins in rat skeletal muscle. Biochem J. 1986 Feb 15;234(1):237–240. doi: 10.1042/bj2340237. [DOI] [PMC free article] [PubMed] [Google Scholar]
- Medina R., Wing S. S., Haas A., Goldberg A. L. Activation of the ubiquitin-ATP-dependent proteolytic system in skeletal muscle during fasting and denervation atrophy. Biomed Biochim Acta. 1991;50(4-6):347–356. [PubMed] [Google Scholar]
- Mitch W. E., Medina R., Grieber S., May R. C., England B. K., Price S. R., Bailey J. L., Goldberg A. L. Metabolic acidosis stimulates muscle protein degradation by activating the adenosine triphosphate-dependent pathway involving ubiquitin and proteasomes. J Clin Invest. 1994 May;93(5):2127–2133. doi: 10.1172/JCI117208. [DOI] [PMC free article] [PubMed] [Google Scholar]
- Mortimore G. E., Hutson N. J., Surmacz C. A. Quantitative correlation between proteolysis and macro- and microautophagy in mouse hepatocytes during starvation and refeeding. Proc Natl Acad Sci U S A. 1983 Apr;80(8):2179–2183. doi: 10.1073/pnas.80.8.2179. [DOI] [PMC free article] [PubMed] [Google Scholar]
- Mortimore G. E., Pösö A. R., Lardeux B. R. Mechanism and regulation of protein degradation in liver. Diabetes Metab Rev. 1989 Feb;5(1):49–70. doi: 10.1002/dmr.5610050105. [DOI] [PubMed] [Google Scholar]
- Orlowski M. The multicatalytic proteinase complex, a major extralysosomal proteolytic system. Biochemistry. 1990 Nov 13;29(45):10289–10297. doi: 10.1021/bi00497a001. [DOI] [PubMed] [Google Scholar]
- Rechsteiner M., Hoffman L., Dubiel W. The multicatalytic and 26 S proteases. J Biol Chem. 1993 Mar 25;268(9):6065–6068. [PubMed] [Google Scholar]
- Rechsteiner M. Ubiquitin-mediated pathways for intracellular proteolysis. Annu Rev Cell Biol. 1987;3:1–30. doi: 10.1146/annurev.cb.03.110187.000245. [DOI] [PubMed] [Google Scholar]
- Redman K. L., Rechsteiner M. Identification of the long ubiquitin extension as ribosomal protein S27a. Nature. 1989 Mar 30;338(6214):438–440. doi: 10.1038/338438a0. [DOI] [PubMed] [Google Scholar]
- Riley D. A., Bain J. L., Ellis S., Haas A. L. Quantitation and immunocytochemical localization of ubiquitin conjugates within rat red and white skeletal muscles. J Histochem Cytochem. 1988 Jun;36(6):621–632. doi: 10.1177/36.6.2835410. [DOI] [PubMed] [Google Scholar]
- Rivett A. J. Proteasomes: multicatalytic proteinase complexes. Biochem J. 1993 Apr 1;291(Pt 1):1–10. doi: 10.1042/bj2910001. [DOI] [PMC free article] [PubMed] [Google Scholar]
- Schwartz L. M., Kosz L., Kay B. K. Gene activation is required for developmentally programmed cell death. Proc Natl Acad Sci U S A. 1990 Sep;87(17):6594–6598. doi: 10.1073/pnas.87.17.6594. [DOI] [PMC free article] [PubMed] [Google Scholar]
- Sugden P. H., Fuller S. J. Regulation of protein turnover in skeletal and cardiac muscle. Biochem J. 1991 Jan 1;273(Pt 1):21–37. doi: 10.1042/bj2730021. [DOI] [PMC free article] [PubMed] [Google Scholar]
- Tokunaga F., Aruga R., Iwanaga S., Tanaka K., Ichihara A., Takao T., Shimonishi Y. The NH2-terminal residues of rat liver proteasome (multicatalytic proteinase complex) subunits, C2, C3 and C8, are N alpha-acetylated. FEBS Lett. 1990 Apr 24;263(2):373–375. doi: 10.1016/0014-5793(90)81417-m. [DOI] [PubMed] [Google Scholar]
- Touitou I., Cavaillès V., Garcia M., Defrenne A., Rochefort H. Differential regulation of cathepsin D by sex steroids in mammary cancer and uterine cells. Mol Cell Endocrinol. 1989 Oct;66(2):231–238. doi: 10.1016/0303-7207(89)90035-x. [DOI] [PubMed] [Google Scholar]
- Troen B. R., Gal S., Gottesman M. M. Sequence and expression of the cDNA for MEP (major excreted protein), a transformation-regulated secreted cathepsin. Biochem J. 1987 Sep 15;246(3):731–735. doi: 10.1042/bj2460731. [DOI] [PMC free article] [PubMed] [Google Scholar]
- Voellmy R., Ahmed A., Schiller P., Bromley P., Rungger D. Isolation and functional analysis of a human 70,000-dalton heat shock protein gene segment. Proc Natl Acad Sci U S A. 1985 Aug;82(15):4949–4953. doi: 10.1073/pnas.82.15.4949. [DOI] [PMC free article] [PubMed] [Google Scholar]
- Waxman L., Fagan J. M., Goldberg A. L. Demonstration of two distinct high molecular weight proteases in rabbit reticulocytes, one of which degrades ubiquitin conjugates. J Biol Chem. 1987 Feb 25;262(6):2451–2457. [PubMed] [Google Scholar]
- Wing S. S., Chiang H. L., Goldberg A. L., Dice J. F. Proteins containing peptide sequences related to Lys-Phe-Glu-Arg-Gln are selectively depleted in liver and heart, but not skeletal muscle, of fasted rats. Biochem J. 1991 Apr 1;275(Pt 1):165–169. doi: 10.1042/bj2750165. [DOI] [PMC free article] [PubMed] [Google Scholar]
- Wing S. S., Goldberg A. L. Glucocorticoids activate the ATP-ubiquitin-dependent proteolytic system in skeletal muscle during fasting. Am J Physiol. 1993 Apr;264(4 Pt 1):E668–E676. doi: 10.1152/ajpendo.1993.264.4.E668. [DOI] [PubMed] [Google Scholar]
- Wing S. S., Haas A. L., Goldberg A. L. Increase in ubiquitin-protein conjugates concomitant with the increase in proteolysis in rat skeletal muscle during starvation and atrophy denervation. Biochem J. 1995 May 1;307(Pt 3):639–645. doi: 10.1042/bj3070639. [DOI] [PMC free article] [PubMed] [Google Scholar]
- Zeman R. J., Kameyama T., Matsumoto K., Bernstein P., Etlinger J. D. Regulation of protein degradation in muscle by calcium. Evidence for enhanced nonlysosomal proteolysis associated with elevated cytosolic calcium. J Biol Chem. 1985 Nov 5;260(25):13619–13624. [PubMed] [Google Scholar]