Abstract
Roles for ubiquitin (an 8.5 kDa polypeptide) involve its conjugation to proteins as a signal to initiate degradation and as a stress protein. We investigated ubiquitin conjugation and ubiquitin-dependent proteolytic activities in cultured bovine lens epithelial cells (BLECs) upon oxidative challenge. A 44% decrease in intracellular glutathione confirmed oxidative stress upon incubation with 1 mM H2O2. After 30 min incubation, endogenous high-molecular-mass ubiquitin conjugates decreased 73%, and intracellular proteolysis decreased about 50%. In the supernatants of the oxidatively treated BLECs, the ability to form high-molecular-mass ubiquitin conjugates with exogenous 125I-labelled ubiquitin decreased 28%, and ATP-dependent degradation of oxidized alpha-crystallin decreased 36%. When the H2O2-treated BLECs were allowed to recover for 60 min, intracellular proteolysis returned to the level of control cells. There was also a subsequent transient enhancement of intracellular proteolysis and a simultaneous recovery of endogenous high-molecular-mass ubiquitin conjugates. In parallel cell-free experiments, conjugating activity with exogenous 125I-labelled ubiquitin and ATP-dependent degradation of oxidized alpha-crystallin increased 35% and 72% respectively compared with non-oxidatively treated BLECs. ATP-independent proteolysis showed little response to exposure or removal of H2O2. These results indicate that (1) the rate of intracellular proteolysis in BLECs is associated with the level of endogenous high-molecular-mass ubiquitin conjugates and (2) oxidative stress may inactivate the ubiquitin conjugation activity with coordinate depression of proteolytic capability. Enhancement in ubiquitin conjugation and proteolytic activities during recovery from oxidative stress may be important in removal of damaged proteins and restoration of normal function of BLECs. The inactivation of ubiquitin-dependent proteolysis by oxidation may be involved in the accumulation of altered proteins and other adverse sequelae in the oxidatively challenged aging lens.
Full text
PDF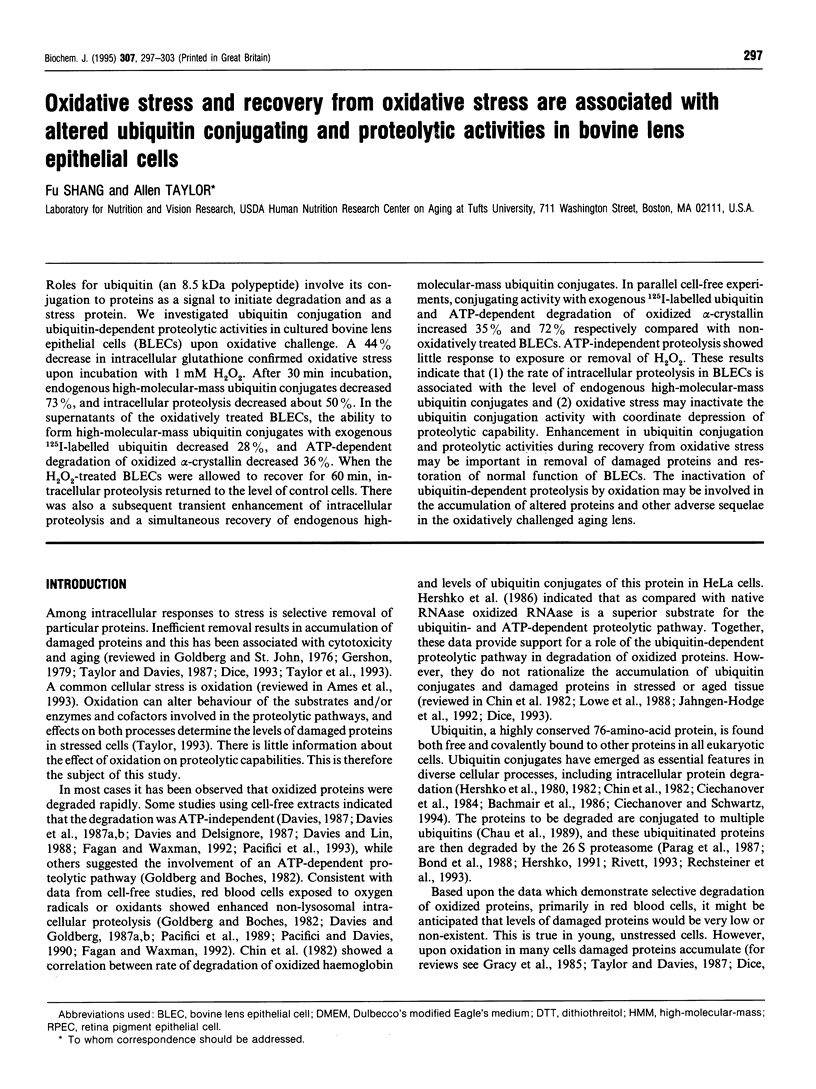
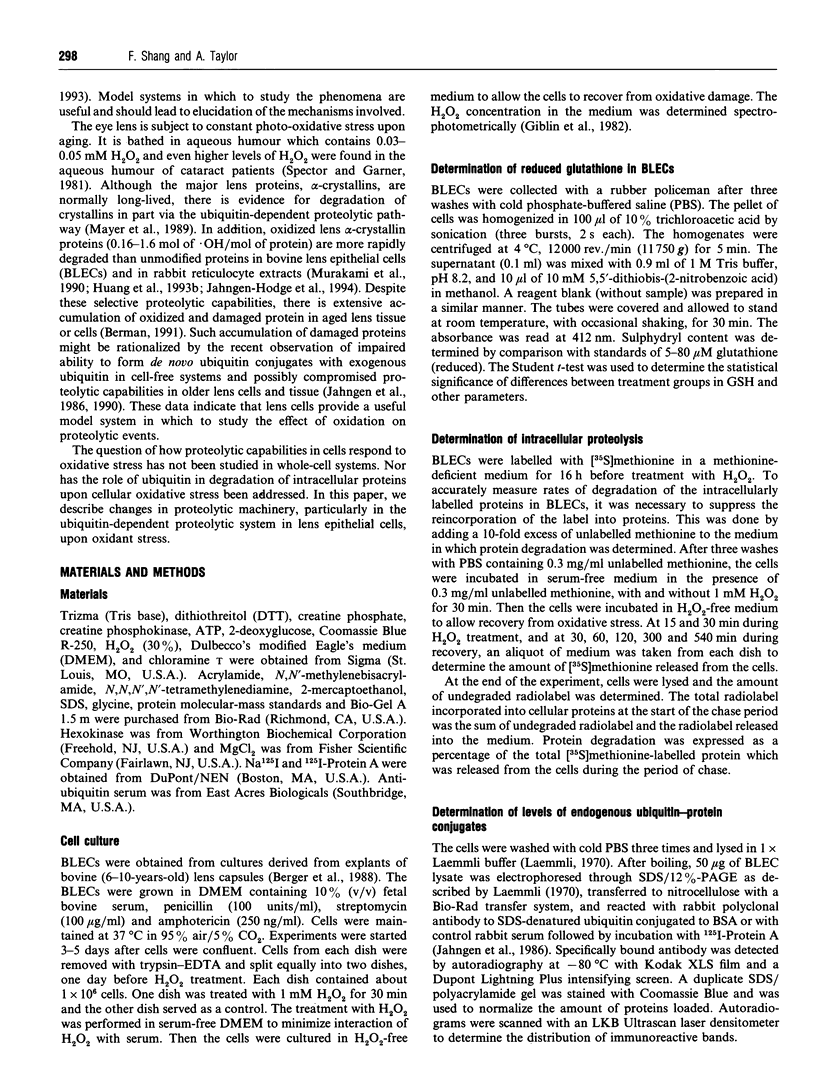
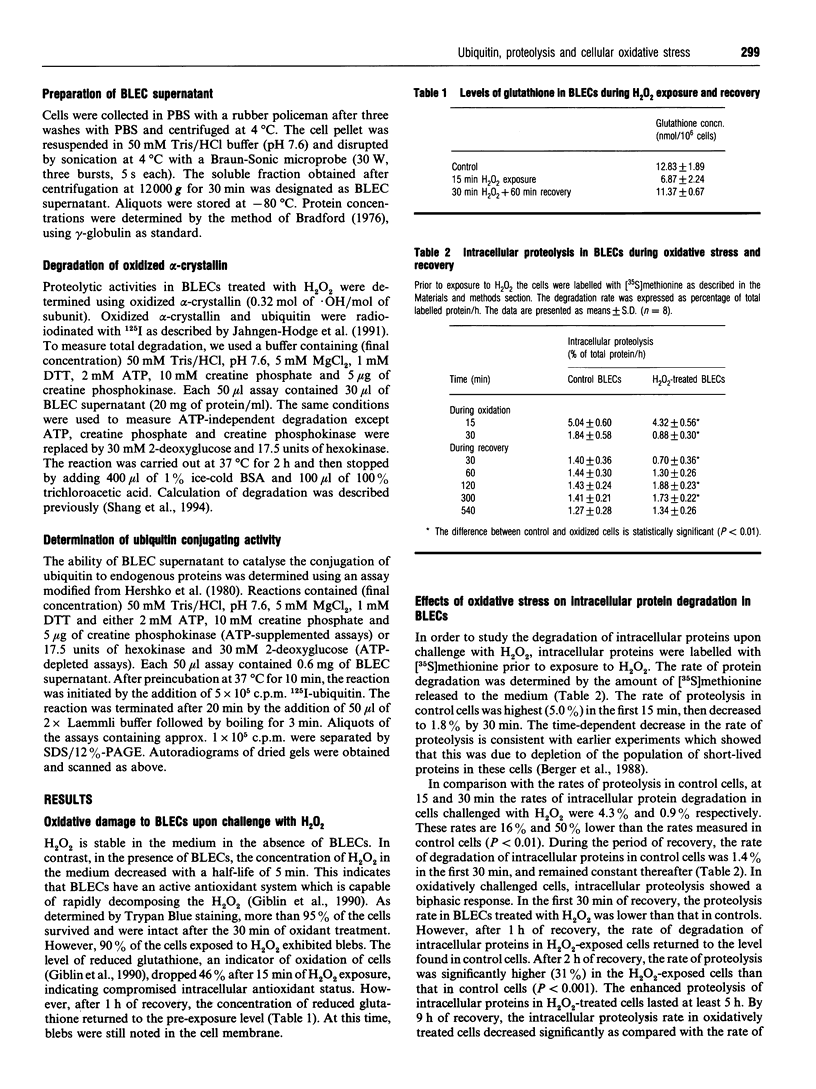
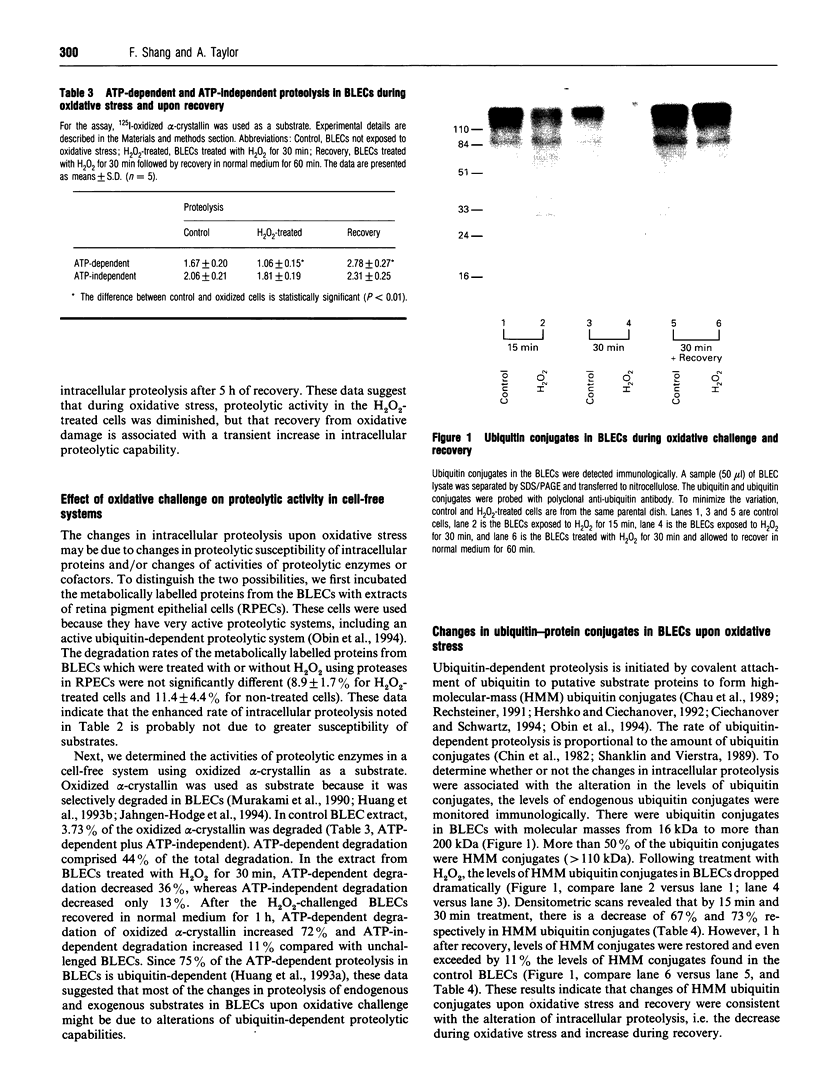
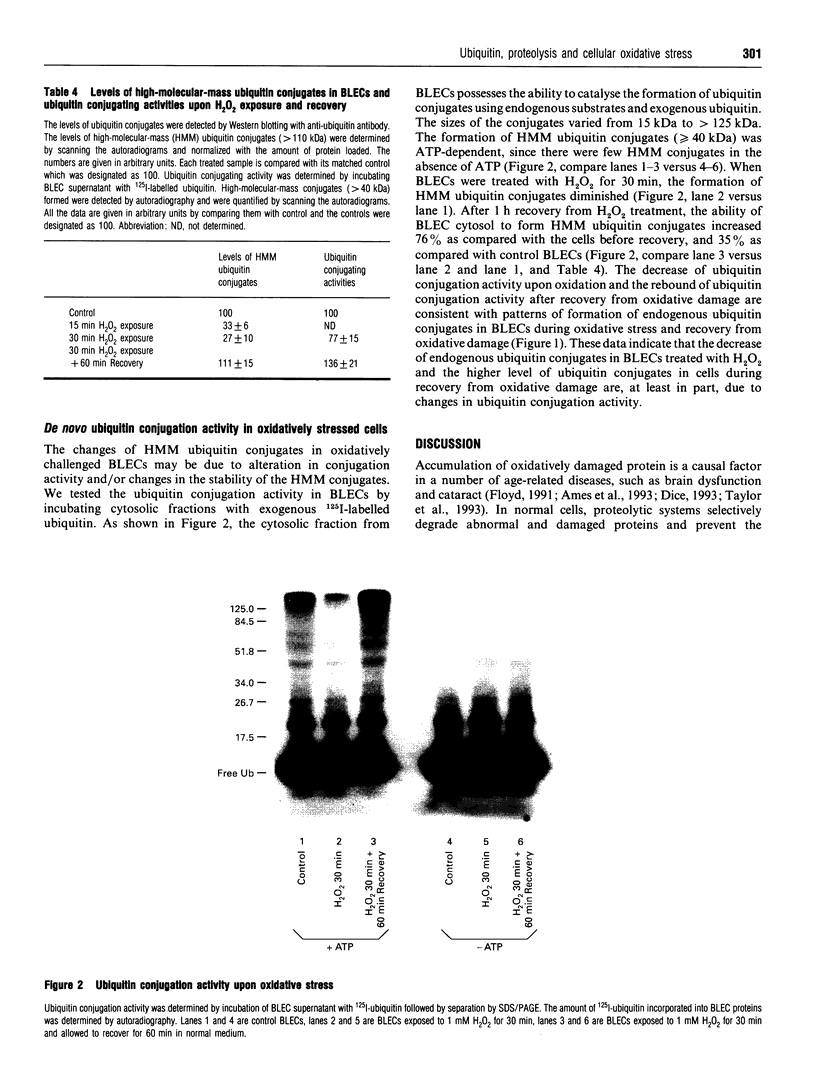
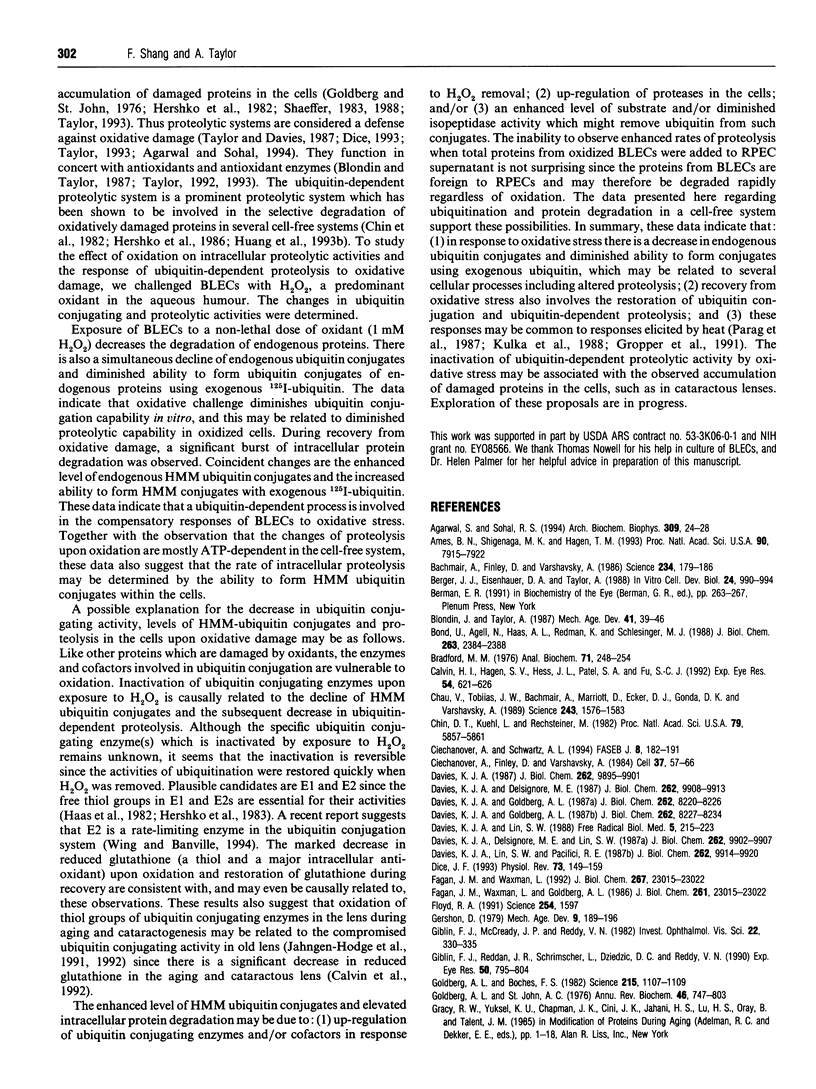
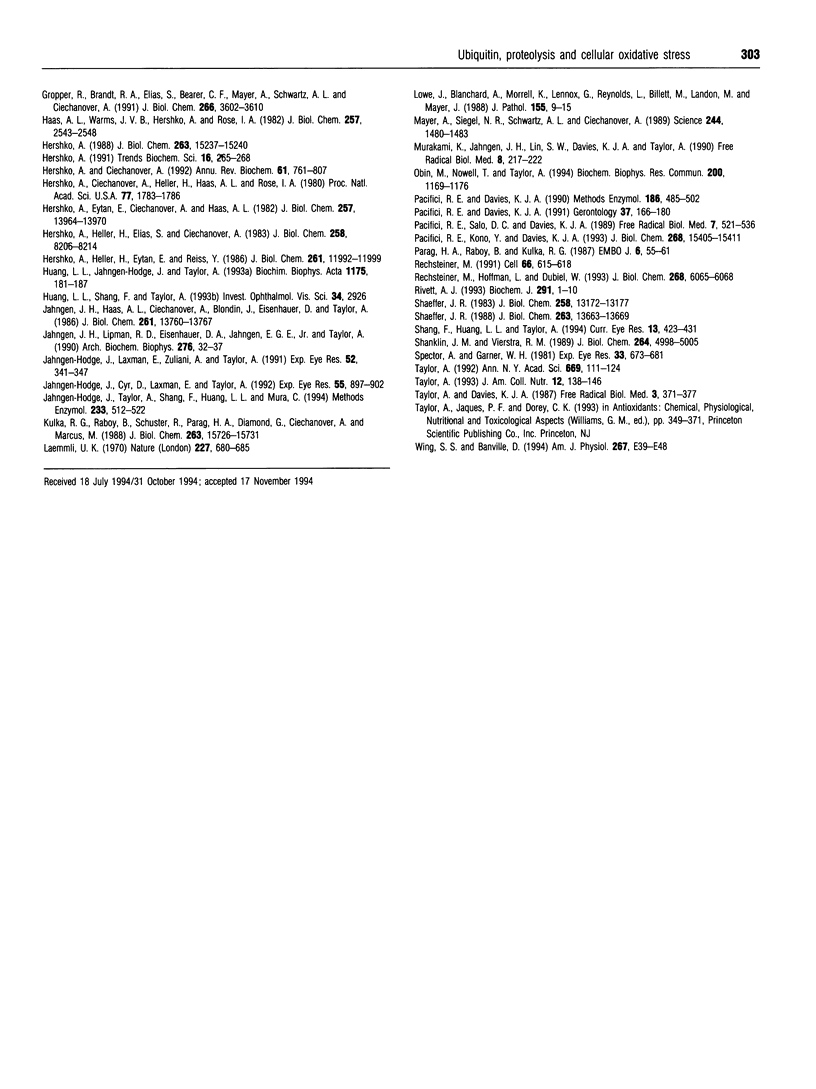
Images in this article
Selected References
These references are in PubMed. This may not be the complete list of references from this article.
- Agarwal S., Sohal R. S. Aging and proteolysis of oxidized proteins. Arch Biochem Biophys. 1994 Feb 15;309(1):24–28. doi: 10.1006/abbi.1994.1078. [DOI] [PubMed] [Google Scholar]
- Ames B. N., Shigenaga M. K., Hagen T. M. Oxidants, antioxidants, and the degenerative diseases of aging. Proc Natl Acad Sci U S A. 1993 Sep 1;90(17):7915–7922. doi: 10.1073/pnas.90.17.7915. [DOI] [PMC free article] [PubMed] [Google Scholar]
- Bachmair A., Finley D., Varshavsky A. In vivo half-life of a protein is a function of its amino-terminal residue. Science. 1986 Oct 10;234(4773):179–186. doi: 10.1126/science.3018930. [DOI] [PubMed] [Google Scholar]
- Berger J. J., Eisenhauer D. A., Taylor A. Intracellular protein degradation in cultured bovine lens epithelial cells. In Vitro Cell Dev Biol. 1988 Oct;24(10):990–994. doi: 10.1007/BF02620871. [DOI] [PubMed] [Google Scholar]
- Blondin J., Taylor A. Measures of leucine aminopeptidase can be used to anticipate UV-induced age-related damage to lens proteins: ascorbate can delay this damage. Mech Ageing Dev. 1987 Nov;41(1-2):39–46. doi: 10.1016/0047-6374(87)90052-2. [DOI] [PubMed] [Google Scholar]
- Bond U., Agell N., Haas A. L., Redman K., Schlesinger M. J. Ubiquitin in stressed chicken embryo fibroblasts. J Biol Chem. 1988 Feb 15;263(5):2384–2388. [PubMed] [Google Scholar]
- Bradford M. M. A rapid and sensitive method for the quantitation of microgram quantities of protein utilizing the principle of protein-dye binding. Anal Biochem. 1976 May 7;72:248–254. doi: 10.1006/abio.1976.9999. [DOI] [PubMed] [Google Scholar]
- Calvin H. I., von Hagen S., Hess J. L., Patel S. A., Fu S. C. Lens GSH depletion and electrolyte changes preceding cataracts induced by buthionine sulfoximine in suckling mice. Exp Eye Res. 1992 Apr;54(4):621–626. doi: 10.1016/0014-4835(92)90141-e. [DOI] [PubMed] [Google Scholar]
- Chau V., Tobias J. W., Bachmair A., Marriott D., Ecker D. J., Gonda D. K., Varshavsky A. A multiubiquitin chain is confined to specific lysine in a targeted short-lived protein. Science. 1989 Mar 24;243(4898):1576–1583. doi: 10.1126/science.2538923. [DOI] [PubMed] [Google Scholar]
- Chin D. T., Kuehl L., Rechsteiner M. Conjugation of ubiquitin to denatured hemoglobin is proportional to the rate of hemoglobin degradation in HeLa cells. Proc Natl Acad Sci U S A. 1982 Oct;79(19):5857–5861. doi: 10.1073/pnas.79.19.5857. [DOI] [PMC free article] [PubMed] [Google Scholar]
- Ciechanover A., Finley D., Varshavsky A. Ubiquitin dependence of selective protein degradation demonstrated in the mammalian cell cycle mutant ts85. Cell. 1984 May;37(1):57–66. doi: 10.1016/0092-8674(84)90300-3. [DOI] [PubMed] [Google Scholar]
- Ciechanover A., Schwartz A. L. The ubiquitin-mediated proteolytic pathway: mechanisms of recognition of the proteolytic substrate and involvement in the degradation of native cellular proteins. FASEB J. 1994 Feb;8(2):182–191. doi: 10.1096/fasebj.8.2.8119489. [DOI] [PubMed] [Google Scholar]
- Davies K. J., Delsignore M. E., Lin S. W. Protein damage and degradation by oxygen radicals. II. Modification of amino acids. J Biol Chem. 1987 Jul 15;262(20):9902–9907. [PubMed] [Google Scholar]
- Davies K. J., Delsignore M. E. Protein damage and degradation by oxygen radicals. III. Modification of secondary and tertiary structure. J Biol Chem. 1987 Jul 15;262(20):9908–9913. [PubMed] [Google Scholar]
- Davies K. J., Goldberg A. L. Oxygen radicals stimulate intracellular proteolysis and lipid peroxidation by independent mechanisms in erythrocytes. J Biol Chem. 1987 Jun 15;262(17):8220–8226. [PubMed] [Google Scholar]
- Davies K. J., Goldberg A. L. Proteins damaged by oxygen radicals are rapidly degraded in extracts of red blood cells. J Biol Chem. 1987 Jun 15;262(17):8227–8234. [PubMed] [Google Scholar]
- Davies K. J., Lin S. W. Degradation of oxidatively denatured proteins in Escherichia coli. Free Radic Biol Med. 1988;5(4):215–223. doi: 10.1016/0891-5849(88)90015-9. [DOI] [PubMed] [Google Scholar]
- Davies K. J., Lin S. W., Pacifici R. E. Protein damage and degradation by oxygen radicals. IV. Degradation of denatured protein. J Biol Chem. 1987 Jul 15;262(20):9914–9920. [PubMed] [Google Scholar]
- Davies K. J. Protein damage and degradation by oxygen radicals. I. general aspects. J Biol Chem. 1987 Jul 15;262(20):9895–9901. [PubMed] [Google Scholar]
- Dice J. F. Cellular and molecular mechanisms of aging. Physiol Rev. 1993 Jan;73(1):149–159. doi: 10.1152/physrev.1993.73.1.149. [DOI] [PubMed] [Google Scholar]
- Eichhorn M., Horneber M., Streilein J. W., Lutjen-Drecoll E. Anterior chamber-associated immune deviation elicited via primate eyes. Invest Ophthalmol Vis Sci. 1993 Sep;34(10):2926–2930. [PubMed] [Google Scholar]
- Fagan J. M., Waxman L. The ATP-independent pathway in red blood cells that degrades oxidant-damaged hemoglobin. J Biol Chem. 1992 Nov 15;267(32):23015–23022. [PubMed] [Google Scholar]
- Floyd R. A. Oxidative damage to behavior during aging. Science. 1991 Dec 13;254(5038):1597–1597. doi: 10.1126/science.1684251. [DOI] [PubMed] [Google Scholar]
- Gershon D. Current status of age altered enzymes: alternative mechanisms. Mech Ageing Dev. 1979 Feb;9(3-4):189–196. doi: 10.1016/0047-6374(79)90098-8. [DOI] [PubMed] [Google Scholar]
- Giblin F. J., McCready J. P., Reddy V. N. The role of glutathione metabolism in the detoxification of H2O2 in rabbit lens. Invest Ophthalmol Vis Sci. 1982 Mar;22(3):330–335. [PubMed] [Google Scholar]
- Giblin F. J., Reddan J. R., Schrimscher L., Dziedzic D. C., Reddy V. N. The relative roles of the glutathione redox cycle and catalase in the detoxification of H2O2 by cultured rabbit lens epithelial cells. Exp Eye Res. 1990 Jun;50(6):795–804. doi: 10.1016/0014-4835(90)90130-m. [DOI] [PubMed] [Google Scholar]
- Goldberg A. L., Boches F. S. Oxidized proteins in erythrocytes are rapidly degraded by the adenosine triphosphate-dependent proteolytic system. Science. 1982 Feb 26;215(4536):1107–1109. doi: 10.1126/science.7038874. [DOI] [PubMed] [Google Scholar]
- Goldberg A. L., St John A. C. Intracellular protein degradation in mammalian and bacterial cells: Part 2. Annu Rev Biochem. 1976;45:747–803. doi: 10.1146/annurev.bi.45.070176.003531. [DOI] [PubMed] [Google Scholar]
- Gropper R., Brandt R. A., Elias S., Bearer C. F., Mayer A., Schwartz A. L., Ciechanover A. The ubiquitin-activating enzyme, E1, is required for stress-induced lysosomal degradation of cellular proteins. J Biol Chem. 1991 Feb 25;266(6):3602–3610. [PubMed] [Google Scholar]
- Haas A. L., Warms J. V., Hershko A., Rose I. A. Ubiquitin-activating enzyme. Mechanism and role in protein-ubiquitin conjugation. J Biol Chem. 1982 Mar 10;257(5):2543–2548. [PubMed] [Google Scholar]
- Hershko A., Ciechanover A., Heller H., Haas A. L., Rose I. A. Proposed role of ATP in protein breakdown: conjugation of protein with multiple chains of the polypeptide of ATP-dependent proteolysis. Proc Natl Acad Sci U S A. 1980 Apr;77(4):1783–1786. doi: 10.1073/pnas.77.4.1783. [DOI] [PMC free article] [PubMed] [Google Scholar]
- Hershko A., Ciechanover A. The ubiquitin system for protein degradation. Annu Rev Biochem. 1992;61:761–807. doi: 10.1146/annurev.bi.61.070192.003553. [DOI] [PubMed] [Google Scholar]
- Hershko A., Eytan E., Ciechanover A., Haas A. L. Immunochemical analysis of the turnover of ubiquitin-protein conjugates in intact cells. Relationship to the breakdown of abnormal proteins. J Biol Chem. 1982 Dec 10;257(23):13964–13970. [PubMed] [Google Scholar]
- Hershko A., Heller H., Elias S., Ciechanover A. Components of ubiquitin-protein ligase system. Resolution, affinity purification, and role in protein breakdown. J Biol Chem. 1983 Jul 10;258(13):8206–8214. [PubMed] [Google Scholar]
- Hershko A., Heller H., Eytan E., Reiss Y. The protein substrate binding site of the ubiquitin-protein ligase system. J Biol Chem. 1986 Sep 15;261(26):11992–11999. [PubMed] [Google Scholar]
- Hershko A. The ubiquitin pathway for protein degradation. Trends Biochem Sci. 1991 Jul;16(7):265–268. doi: 10.1016/0968-0004(91)90101-z. [DOI] [PubMed] [Google Scholar]
- Hershko A. Ubiquitin-mediated protein degradation. J Biol Chem. 1988 Oct 25;263(30):15237–15240. [PubMed] [Google Scholar]
- Huang L. L., Jahngen-Hodge J., Taylor A. Bovine lens epithelial cells have a ubiquitin-dependent proteolysis system. Biochim Biophys Acta. 1993 Jan 17;1175(2):181–187. doi: 10.1016/0167-4889(93)90021-g. [DOI] [PubMed] [Google Scholar]
- Jabben M., Shanklin J., Vierstra R. D. Ubiquitin-phytochrome conjugates. Pool dynamics during in vivo phytochrome degradation. J Biol Chem. 1989 Mar 25;264(9):4998–5005. [PubMed] [Google Scholar]
- Jahngen-Hodge J., Cyr D., Laxman E., Taylor A. Ubiquitin and ubiquitin conjugates in human lens. Exp Eye Res. 1992 Dec;55(6):897–902. doi: 10.1016/0014-4835(92)90016-l. [DOI] [PubMed] [Google Scholar]
- Jahngen-Hodge J., Laxman E., Zuliani A., Taylor A. Evidence for ATP and ubiquitin dependent degradation of proteins in cultured bovine lens epithelial cells. Exp Eye Res. 1991 Mar;52(3):341–347. doi: 10.1016/0014-4835(91)90099-z. [DOI] [PubMed] [Google Scholar]
- Jahngen-Hodge J., Taylor A., Shang F., Huang L. L., Mura C. Oxidative stress to lens crystallins. Methods Enzymol. 1994;233:512–522. doi: 10.1016/s0076-6879(94)33057-3. [DOI] [PubMed] [Google Scholar]
- Jahngen J. H., Haas A. L., Ciechanover A., Blondin J., Eisenhauer D., Taylor A. The eye lens has an active ubiquitin-protein conjugation system. J Biol Chem. 1986 Oct 15;261(29):13760–13767. [PubMed] [Google Scholar]
- Jahngen J. H., Lipman R. D., Eisenhauer D. A., Jahngen E. G., Jr, Taylor A. Aging and cellular maturation cause changes in ubiquitin-eye lens protein conjugates. Arch Biochem Biophys. 1990 Jan;276(1):32–37. doi: 10.1016/0003-9861(90)90006-k. [DOI] [PubMed] [Google Scholar]
- Kulka R. G., Raboy B., Schuster R., Parag H. A., Diamond G., Ciechanover A., Marcus M. A Chinese hamster cell cycle mutant arrested at G2 phase has a temperature-sensitive ubiquitin-activating enzyme, E1. J Biol Chem. 1988 Oct 25;263(30):15726–15731. [PubMed] [Google Scholar]
- Laemmli U. K. Cleavage of structural proteins during the assembly of the head of bacteriophage T4. Nature. 1970 Aug 15;227(5259):680–685. doi: 10.1038/227680a0. [DOI] [PubMed] [Google Scholar]
- Lowe J., Blanchard A., Morrell K., Lennox G., Reynolds L., Billett M., Landon M., Mayer R. J. Ubiquitin is a common factor in intermediate filament inclusion bodies of diverse type in man, including those of Parkinson's disease, Pick's disease, and Alzheimer's disease, as well as Rosenthal fibres in cerebellar astrocytomas, cytoplasmic bodies in muscle, and mallory bodies in alcoholic liver disease. J Pathol. 1988 May;155(1):9–15. doi: 10.1002/path.1711550105. [DOI] [PubMed] [Google Scholar]
- Mayer A., Siegel N. R., Schwartz A. L., Ciechanover A. Degradation of proteins with acetylated amino termini by the ubiquitin system. Science. 1989 Jun 23;244(4911):1480–1483. doi: 10.1126/science.2544030. [DOI] [PubMed] [Google Scholar]
- Murakami K., Jahngen J. H., Lin S. W., Davies K. J., Taylor A. Lens proteasome shows enhanced rates of degradation of hydroxyl radical modified alpha-crystallin. Free Radic Biol Med. 1990;8(3):217–222. doi: 10.1016/0891-5849(90)90066-r. [DOI] [PubMed] [Google Scholar]
- Obin M., Nowell T., Taylor A. The photoreceptor G-protein transducin (Gt) is a substrate for ubiquitin-dependent proteolysis. Biochem Biophys Res Commun. 1994 May 16;200(3):1169–1176. doi: 10.1006/bbrc.1994.1574. [DOI] [PubMed] [Google Scholar]
- Pacifici R. E., Davies K. J. Protein degradation as an index of oxidative stress. Methods Enzymol. 1990;186:485–502. doi: 10.1016/0076-6879(90)86143-j. [DOI] [PubMed] [Google Scholar]
- Pacifici R. E., Davies K. J. Protein, lipid and DNA repair systems in oxidative stress: the free-radical theory of aging revisited. Gerontology. 1991;37(1-3):166–180. doi: 10.1159/000213257. [DOI] [PubMed] [Google Scholar]
- Pacifici R. E., Kono Y., Davies K. J. Hydrophobicity as the signal for selective degradation of hydroxyl radical-modified hemoglobin by the multicatalytic proteinase complex, proteasome. J Biol Chem. 1993 Jul 25;268(21):15405–15411. [PubMed] [Google Scholar]
- Pacifici R. E., Salo D. C., Davies K. J. Macroxyproteinase (M.O.P.): a 670 kDa proteinase complex that degrades oxidatively denatured proteins in red blood cells. Free Radic Biol Med. 1989;7(5):521–536. doi: 10.1016/0891-5849(89)90028-2. [DOI] [PubMed] [Google Scholar]
- Parag H. A., Raboy B., Kulka R. G. Effect of heat shock on protein degradation in mammalian cells: involvement of the ubiquitin system. EMBO J. 1987 Jan;6(1):55–61. doi: 10.1002/j.1460-2075.1987.tb04718.x. [DOI] [PMC free article] [PubMed] [Google Scholar]
- Rechsteiner M., Hoffman L., Dubiel W. The multicatalytic and 26 S proteases. J Biol Chem. 1993 Mar 25;268(9):6065–6068. [PubMed] [Google Scholar]
- Rechsteiner M. Natural substrates of the ubiquitin proteolytic pathway. Cell. 1991 Aug 23;66(4):615–618. doi: 10.1016/0092-8674(91)90104-7. [DOI] [PubMed] [Google Scholar]
- Rivett A. J. Proteasomes: multicatalytic proteinase complexes. Biochem J. 1993 Apr 1;291(Pt 1):1–10. doi: 10.1042/bj2910001. [DOI] [PMC free article] [PubMed] [Google Scholar]
- Shaeffer J. R. ATP-dependent proteolysis of hemoglobin alpha chains in beta-thalassemic hemolysates is ubiquitin-dependent. J Biol Chem. 1988 Sep 25;263(27):13663–13669. [PubMed] [Google Scholar]
- Shaeffer J. R. Turnover of excess hemoglobin alpha chains in beta-thalassemic cells is ATP-dependent. J Biol Chem. 1983 Nov 10;258(21):13172–13177. [PubMed] [Google Scholar]
- Shang F., Huang L., Taylor A. Degradation of native and oxidized beta- and gamma-crystallin using bovine lens epithelial cell and rabbit reticulocyte extracts. Curr Eye Res. 1994 Jun;13(6):423–431. doi: 10.3109/02713689408999870. [DOI] [PubMed] [Google Scholar]
- Spector A., Garner W. H. Hydrogen peroxide and human cataract. Exp Eye Res. 1981 Dec;33(6):673–681. doi: 10.1016/s0014-4835(81)80107-8. [DOI] [PubMed] [Google Scholar]
- Taylor A. Cataract: relationship between nutrition and oxidation. J Am Coll Nutr. 1993 Apr;12(2):138–146. doi: 10.1080/07315724.1993.10718294. [DOI] [PubMed] [Google Scholar]
- Taylor A., Davies K. J. Protein oxidation and loss of protease activity may lead to cataract formation in the aged lens. Free Radic Biol Med. 1987;3(6):371–377. doi: 10.1016/0891-5849(87)90015-3. [DOI] [PubMed] [Google Scholar]
- Taylor A., Jacques P. F., Dorey C. K. Oxidation and aging: impact on vision. Toxicol Ind Health. 1993 Jan-Apr;9(1-2):349–371. doi: 10.1177/0748233793009001-226. [DOI] [PubMed] [Google Scholar]
- Taylor A. Role of nutrients in delaying cataracts. Ann N Y Acad Sci. 1992 Sep 30;669:111–124. doi: 10.1111/j.1749-6632.1992.tb17093.x. [DOI] [PubMed] [Google Scholar]
- Wing S. S., Banville D. 14-kDa ubiquitin-conjugating enzyme: structure of the rat gene and regulation upon fasting and by insulin. Am J Physiol. 1994 Jul;267(1 Pt 1):E39–E48. doi: 10.1152/ajpendo.1994.267.1.E39. [DOI] [PubMed] [Google Scholar]