Abstract
Mitochondrial creatine kinase (Mi-CK) consists of octameric and dimeric molecules that are interconvertible. In the present study, the kinetic properties of purified chicken heart Mi-CK (Mib-CK) dimers and octamers were investigated separately under highly controlled conditions. Gel-permeation chromatography was performed before and after kinetic measurements in order to clearly define the proportions of octamers and dimers. 'Dimeric' Mi-CK solutions consisted of > or = 90% dimers throughout the experiment whereas 'octameric' Mi-CK solutions consisted in the beginning of 90% octamers, but upon measuring with the highest concentrations of creatine (Cr) and ATP approximately one-third of the octamers dissociated into dimers. These proper controls enabled us to pinpoint the observed kinetic differences between dimers and octamers solely to the oligomeric state of Mib-CK. Both dimeric and octameric Mi-CK displayed synergism in substrate binding (Kd values are higher than Km values), meaning that binding of the first substrate facilities subsequent binding of the second substrate. Most interestingly, Km(Cr) and Kd(Cr) values are both 2-3 times higher for octameric than for dimeric Mi-CK. Thus, at low Cr concentrations, the dimer is kinetically favoured for the forward direction of the reaction (phosphorylcreatine synthesis) compared with the octamer. The possible physiological significance of the lower Kd(Cr) value of dimeric versus octameric Mib-CK, as well as the apparent negative cooperativity of ATP binding at higher [Cr], are discussed within the context of a possible functional role for dimeric Mib-CK in vivo.
Full text
PDF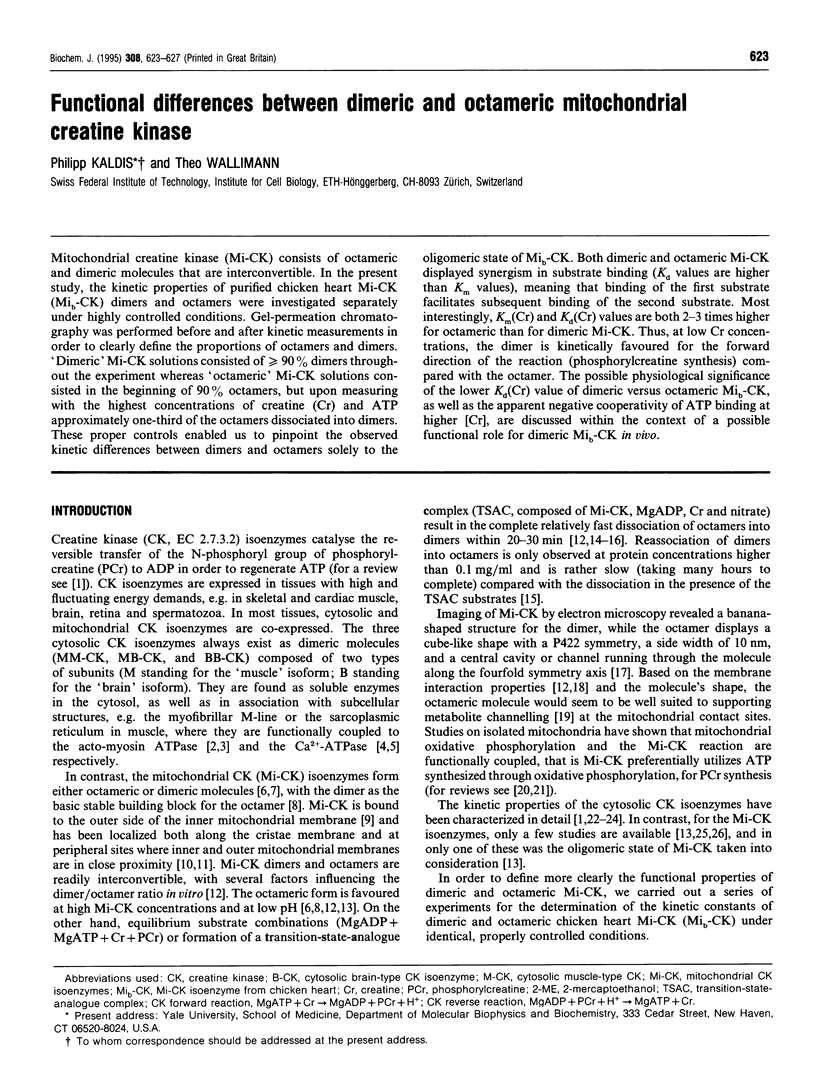
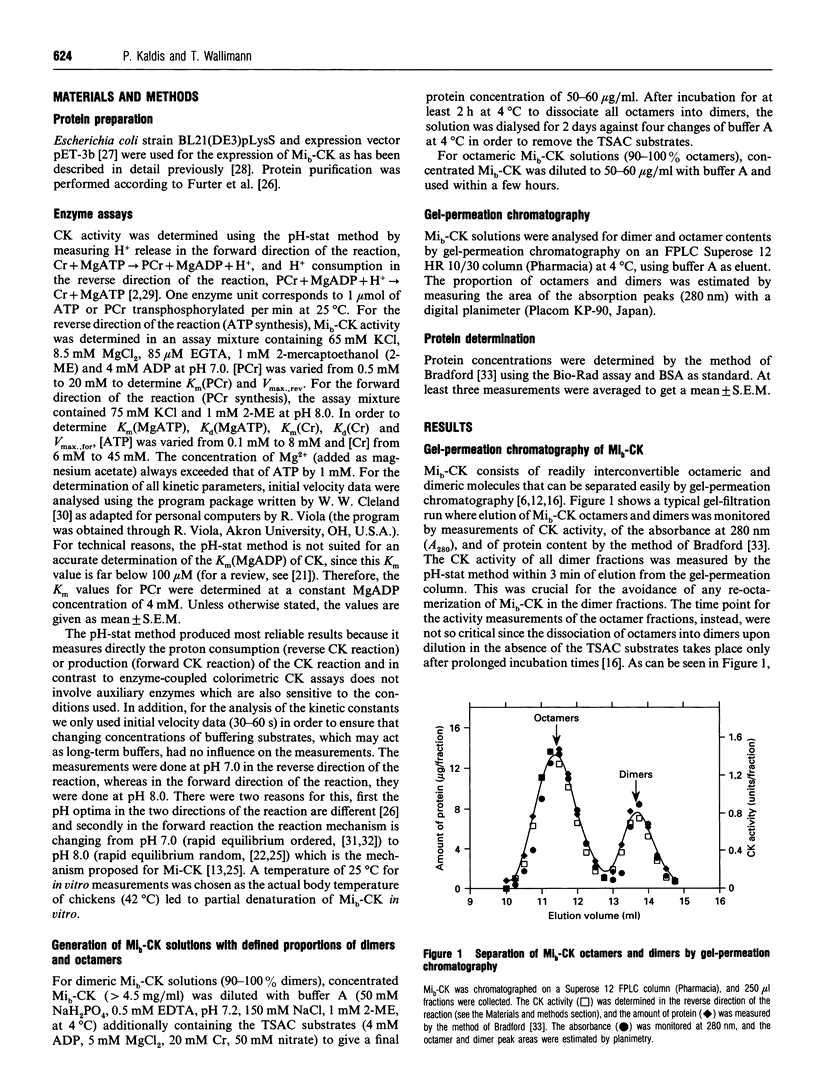
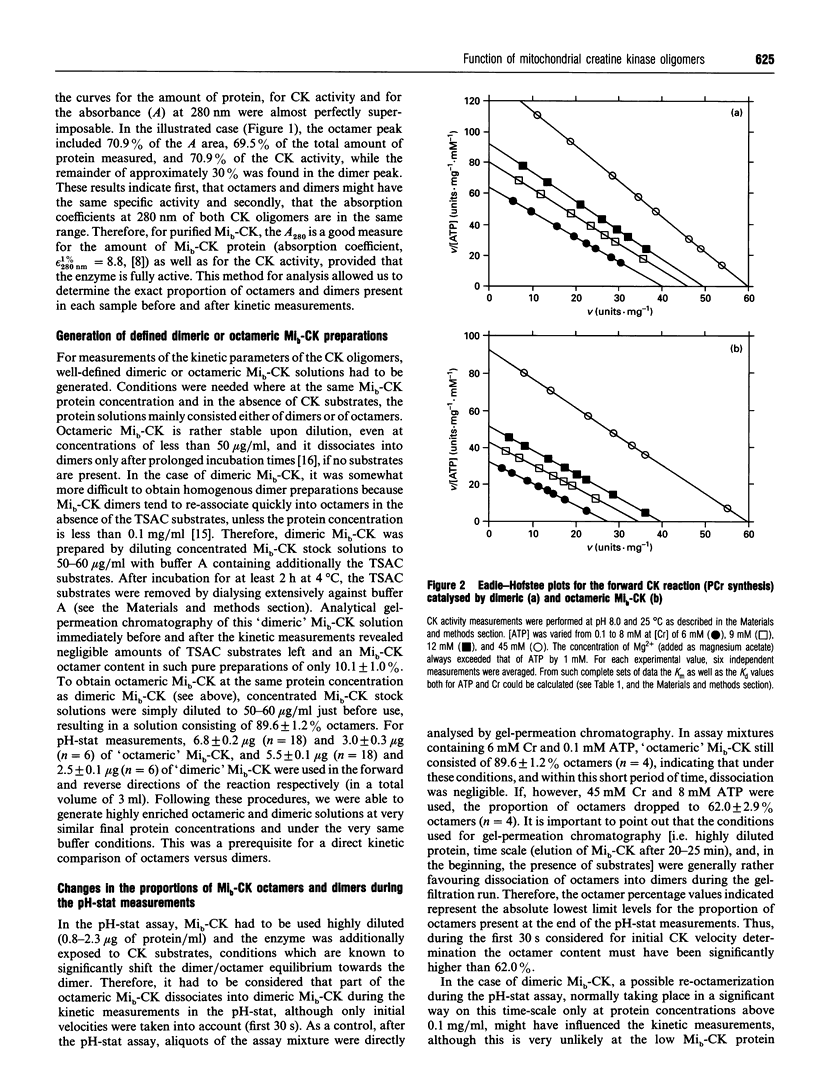
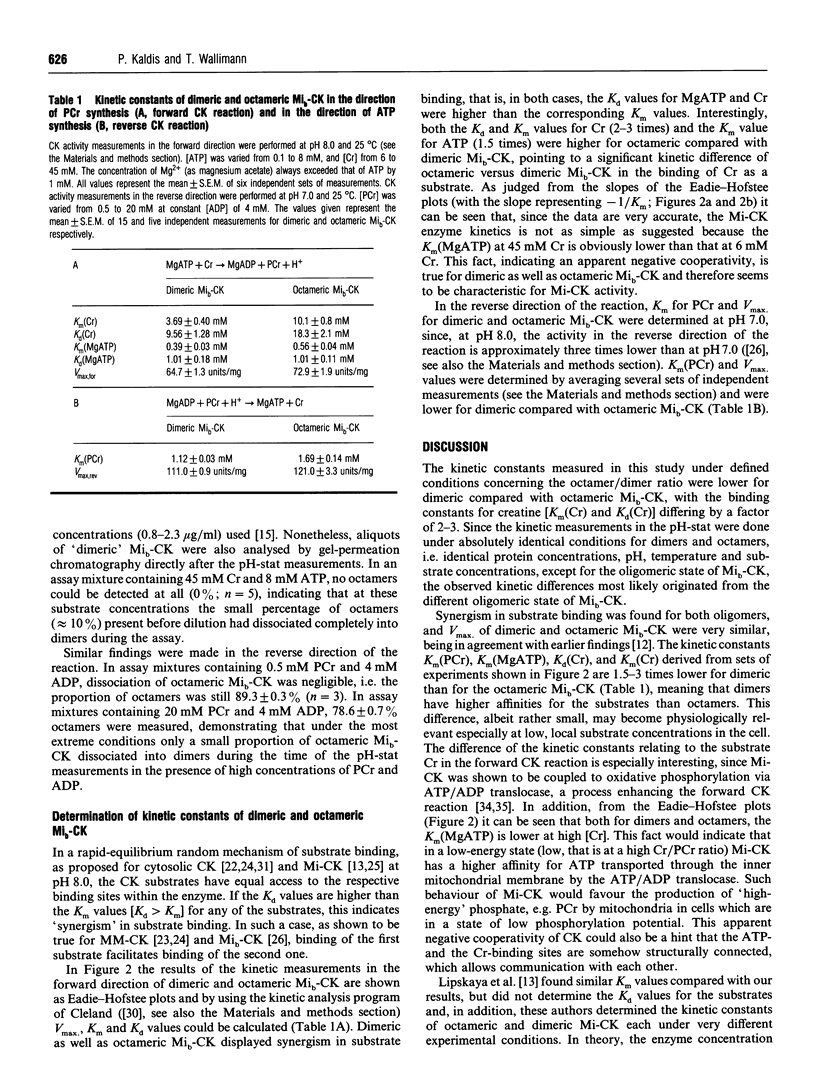
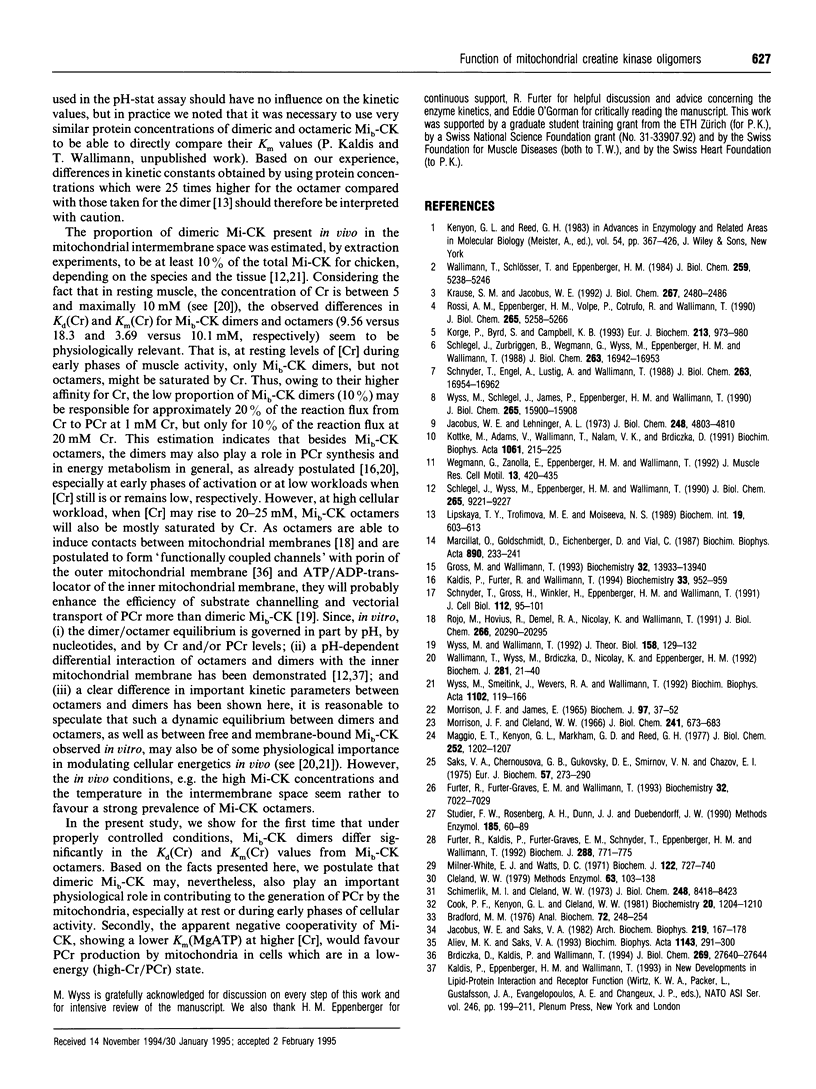
Selected References
These references are in PubMed. This may not be the complete list of references from this article.
- Aliev M. K., Saks V. A. Quantitative analysis of the 'phosphocreatine shuttle': I. A probability approach to the description of phosphocreatine production in the coupled creatine kinase-ATP/ADP translocase-oxidative phosphorylation reactions in heart mitochondria. Biochim Biophys Acta. 1993 Jul 26;1143(3):291–300. doi: 10.1016/0005-2728(93)90200-y. [DOI] [PubMed] [Google Scholar]
- Bradford M. M. A rapid and sensitive method for the quantitation of microgram quantities of protein utilizing the principle of protein-dye binding. Anal Biochem. 1976 May 7;72:248–254. doi: 10.1006/abio.1976.9999. [DOI] [PubMed] [Google Scholar]
- Brdiczka D., Kaldis P., Wallimann T. In vitro complex formation between the octamer of mitochondrial creatine kinase and porin. J Biol Chem. 1994 Nov 4;269(44):27640–27644. [PubMed] [Google Scholar]
- Cleland W. W. Statistical analysis of enzyme kinetic data. Methods Enzymol. 1979;63:103–138. doi: 10.1016/0076-6879(79)63008-2. [DOI] [PubMed] [Google Scholar]
- Cook P. F., Kenyon G. L., Cleland W. W. Use of pH studies to elucidate the catalytic mechanism of rabbit muscle creatine kinase. Biochemistry. 1981 Mar 3;20(5):1204–1210. doi: 10.1021/bi00508a023. [DOI] [PubMed] [Google Scholar]
- Furter R., Furter-Graves E. M., Wallimann T. Creatine kinase: the reactive cysteine is required for synergism but is nonessential for catalysis. Biochemistry. 1993 Jul 13;32(27):7022–7029. doi: 10.1021/bi00078a030. [DOI] [PubMed] [Google Scholar]
- Furter R., Kaldis P., Furter-Graves E. M., Schnyder T., Eppenberger H. M., Wallimann T. Expression of active octameric chicken cardiac mitochondrial creatine kinase in Escherichia coli. Biochem J. 1992 Dec 15;288(Pt 3):771–775. doi: 10.1042/bj2880771. [DOI] [PMC free article] [PubMed] [Google Scholar]
- Gross M., Wallimann T. Kinetics of assembly and dissociation of the mitochondrial creatine kinase octamer. A fluorescence study. Biochemistry. 1993 Dec 21;32(50):13933–13940. doi: 10.1021/bi00213a024. [DOI] [PubMed] [Google Scholar]
- Jacobus W. E., Lehninger A. L. Creatine kinase of rat heart mitochondria. Coupling of creatine phosphorylation to electron transport. J Biol Chem. 1973 Jul 10;248(13):4803–4810. [PubMed] [Google Scholar]
- Jacobus W. E., Saks V. A. Creatine kinase of heart mitochondria: changes in its kinetic properties induced by coupling to oxidative phosphorylation. Arch Biochem Biophys. 1982 Nov;219(1):167–178. doi: 10.1016/0003-9861(82)90146-1. [DOI] [PubMed] [Google Scholar]
- Kaldis P., Furter R., Wallimann T. The N-terminal heptapeptide of mitochondrial creatine kinase is important for octamerization. Biochemistry. 1994 Feb 1;33(4):952–959. doi: 10.1021/bi00170a014. [DOI] [PubMed] [Google Scholar]
- Kenyon G. L., Reed G. H. Creatine kinase: structure-activity relationships. Adv Enzymol Relat Areas Mol Biol. 1983;54:367–426. doi: 10.1002/9780470122990.ch6. [DOI] [PubMed] [Google Scholar]
- Korge P., Byrd S. K., Campbell K. B. Functional coupling between sarcoplasmic-reticulum-bound creatine kinase and Ca(2+)-ATPase. Eur J Biochem. 1993 May 1;213(3):973–980. doi: 10.1111/j.1432-1033.1993.tb17842.x. [DOI] [PubMed] [Google Scholar]
- Kottke M., Adams V., Wallimann T., Nalam V. K., Brdiczka D. Location and regulation of octameric mitochondrial creatine kinase in the contact sites. Biochim Biophys Acta. 1991 Jan 30;1061(2):215–225. doi: 10.1016/0005-2736(91)90287-i. [DOI] [PubMed] [Google Scholar]
- Krause S. M., Jacobus W. E. Specific enhancement of the cardiac myofibrillar ATPase by bound creatine kinase. J Biol Chem. 1992 Feb 5;267(4):2480–2486. [PubMed] [Google Scholar]
- Lipskaya TYu, Trofimova M. E., Moiseeva N. S. Kinetic properties of the octameric and dimeric forms of mitochondrial creatine kinase and physiological role of the enzyme. Biochem Int. 1989 Sep;19(3):603–613. [PubMed] [Google Scholar]
- Maggio E. T., Kenyon G. L. Properties of a CH3-blocked creatine kinase with altered catalytic activity. Kinetic consequences of the presence of the blocking group. J Biol Chem. 1977 Feb 25;252(4):1202–1207. [PubMed] [Google Scholar]
- Marcillat O., Goldschmidt D., Eichenberger D., Vial C. Only one of the two interconvertible forms of mitochondrial creatine kinase binds to heart mitoplasts. Biochim Biophys Acta. 1987 Feb 11;890(2):233–241. doi: 10.1016/0005-2728(87)90024-7. [DOI] [PubMed] [Google Scholar]
- Milner-White E. J., Watts D. C. Inhibition of adenosine 5'-triphosphate-creatine phosphotransferase by substrate-anion complexes. Evidence for the transition-state organization of the catalytic site. Biochem J. 1971 May;122(5):727–740. doi: 10.1042/bj1220727. [DOI] [PMC free article] [PubMed] [Google Scholar]
- Morrison J. F., Cleland W. W. Isotope exchange studies of the mechanism of the reaction catalyzed by adenosine triphosphate: creatine phosphotransferase. J Biol Chem. 1966 Feb 10;241(3):673–683. [PubMed] [Google Scholar]
- Morrison J. F., James E. The mechanism of the reaction catalysed by adenosine triphosphate-creatine phosphotransferase. Biochem J. 1965 Oct;97(1):37–52. doi: 10.1042/bj0970037. [DOI] [PMC free article] [PubMed] [Google Scholar]
- Rojo M., Hovius R., Demel R. A., Nicolay K., Wallimann T. Mitochondrial creatine kinase mediates contact formation between mitochondrial membranes. J Biol Chem. 1991 Oct 25;266(30):20290–20295. [PubMed] [Google Scholar]
- Rossi A. M., Eppenberger H. M., Volpe P., Cotrufo R., Wallimann T. Muscle-type MM creatine kinase is specifically bound to sarcoplasmic reticulum and can support Ca2+ uptake and regulate local ATP/ADP ratios. J Biol Chem. 1990 Mar 25;265(9):5258–5266. [PubMed] [Google Scholar]
- Saks V. A., Chernousova G. B., Gukovsky D. E., Smirnov V. N., Chazov E. I. Studies of energy transport in heart cells. Mitochondrial isoenzyme of creatine phosphokinase: kinetic properties and regulatory action of Mg2+ ions. Eur J Biochem. 1975 Sep 1;57(1):273–290. doi: 10.1111/j.1432-1033.1975.tb02299.x. [DOI] [PubMed] [Google Scholar]
- Schimerlik M. I., Cleland W. W. Inhibition of creatine kinase by chromium nucleotides. J Biol Chem. 1973 Dec 25;248(24):8418–8423. [PubMed] [Google Scholar]
- Schlegel J., Wyss M., Eppenberger H. M., Wallimann T. Functional studies with the octameric and dimeric form of mitochondrial creatine kinase. Differential pH-dependent association of the two oligomeric forms with the inner mitochondrial membrane. J Biol Chem. 1990 Jun 5;265(16):9221–9227. [PubMed] [Google Scholar]
- Schlegel J., Zurbriggen B., Wegmann G., Wyss M., Eppenberger H. M., Wallimann T. Native mitochondrial creatine kinase forms octameric structures. I. Isolation of two interconvertible mitochondrial creatine kinase forms, dimeric and octameric mitochondrial creatine kinase: characterization, localization, and structure-function relationships. J Biol Chem. 1988 Nov 15;263(32):16942–16953. [PubMed] [Google Scholar]
- Schnyder T., Engel A., Lustig A., Wallimann T. Native mitochondrial creatine kinase forms octameric structures. II. Characterization of dimers and octamers by ultracentrifugation, direct mass measurements by scanning transmission electron microscopy, and image analysis of single mitochondrial creatine kinase octamers. J Biol Chem. 1988 Nov 15;263(32):16954–16962. [PubMed] [Google Scholar]
- Schnyder T., Gross H., Winkler H., Eppenberger H. M., Wallimann T. Structure of the mitochondrial creatine kinase octamer: high-resolution shadowing and image averaging of single molecules and formation of linear filaments under specific staining conditions. J Cell Biol. 1991 Jan;112(1):95–101. doi: 10.1083/jcb.112.1.95. [DOI] [PMC free article] [PubMed] [Google Scholar]
- Studier F. W., Rosenberg A. H., Dunn J. J., Dubendorff J. W. Use of T7 RNA polymerase to direct expression of cloned genes. Methods Enzymol. 1990;185:60–89. doi: 10.1016/0076-6879(90)85008-c. [DOI] [PubMed] [Google Scholar]
- Wallimann T., Schlösser T., Eppenberger H. M. Function of M-line-bound creatine kinase as intramyofibrillar ATP regenerator at the receiving end of the phosphorylcreatine shuttle in muscle. J Biol Chem. 1984 Apr 25;259(8):5238–5246. [PubMed] [Google Scholar]
- Wallimann T., Wyss M., Brdiczka D., Nicolay K., Eppenberger H. M. Intracellular compartmentation, structure and function of creatine kinase isoenzymes in tissues with high and fluctuating energy demands: the 'phosphocreatine circuit' for cellular energy homeostasis. Biochem J. 1992 Jan 1;281(Pt 1):21–40. doi: 10.1042/bj2810021. [DOI] [PMC free article] [PubMed] [Google Scholar]
- Wegmann G., Zanolla E., Eppenberger H. M., Wallimann T. In situ compartmentation of creatine kinase in intact sarcomeric muscle: the acto-myosin overlap zone as a molecular sieve. J Muscle Res Cell Motil. 1992 Aug;13(4):420–435. doi: 10.1007/BF01738037. [DOI] [PubMed] [Google Scholar]
- Wyss M., Schlegel J., James P., Eppenberger H. M., Wallimann T. Mitochondrial creatine kinase from chicken brain. Purification, biophysical characterization, and generation of heterodimeric and heterooctameric molecules with subunits of other creatine kinase isoenzymes. J Biol Chem. 1990 Sep 15;265(26):15900–15908. [PubMed] [Google Scholar]
- Wyss M., Smeitink J., Wevers R. A., Wallimann T. Mitochondrial creatine kinase: a key enzyme of aerobic energy metabolism. Biochim Biophys Acta. 1992 Sep 25;1102(2):119–166. doi: 10.1016/0005-2728(92)90096-k. [DOI] [PubMed] [Google Scholar]
- Wyss M., Wallimann T. Metabolite channelling in aerobic energy metabolism. J Theor Biol. 1992 Sep 7;158(1):129–132. doi: 10.1016/s0022-5193(05)80650-2. [DOI] [PubMed] [Google Scholar]