Abstract
In order to detect the two splice variant forms of type-IVB cyclic AMP phosphodiesterase (PDE) activity, DPD (type-IVB1) and PDE-4 (type-IVB2), anti-peptide antisera were generated. One set ('DPD/PDE-4-common'), generated against a peptide sequence found at the common C-terminus of these two PDEs, detected both PDEs. A second set was PDE-4 specific, being directed against a peptide sequence found within the unique N-terminal region of PDE-4. In brain, DPD was found exclusively in the cytosol and PDE-4 exclusively associated with membranes. Both brain DPD and PDE-4 activities, isolated by immunoprecipitation, were cyclic AMP-specific (KmcyclicAMP: approximately 5 microM for DPD; approximately 4 microM for PDE-4) and were inhibited by low rolipram concentrations (K1rolipram approximately 1 microM for both). Transient expression of DPD in COS-1 cells allowed identification of an approx. 64 kDa species which co-migrated on SDS/PAGE with the immunoreactive species identified in both brain cytosol and membrane fractions using the DPD/PDE-4-common antisera. The subunit size observed for PDE-4 (approx. 64 kDa) in brain membranes was similar to that predicted from the cDNA sequence, but that observed for DPD was approx. 4 kDa greater. Type-IV, rolipram-inhibited PDE activity was found in all brain regions except the pituitary, where it formed between 30 and 70% of the PDE activity in membrane and cytosolic fractions when assayed with 1 microM cyclic AMP, PDE-4 formed 40-50% of the membrane type-IV activity in all brain regions save the midbrain (approx. 20%). DPD distribution was highly restricted to certain regions, providing approx. 35% of the type-IV cytosolic activity in hippocampus and 13-21% in cortex, hypothalamus and striatum with no presence in brain stem, cerebellum, midbrain and pituitary. The combined type-IVB PDE activities of DPD and PDE-4 contributed approx. 10% of the total PDE activity in most brain regions except for the pituitary (zero) and the mid-brain (approx. 3%. The isolated cDNAs for DPD and PDE-4 appear to reflect transcription products which are expressed in vivo in brain. The unique N-terminal domain of PDE-4 is suggested to target this PDE to membranes in brain. Type-IVB PDEs are differentially expressed in various brain regions, indicating that there are tissue-specific controls on both the expression of the gene and the splicing of its products.
Full text
PDF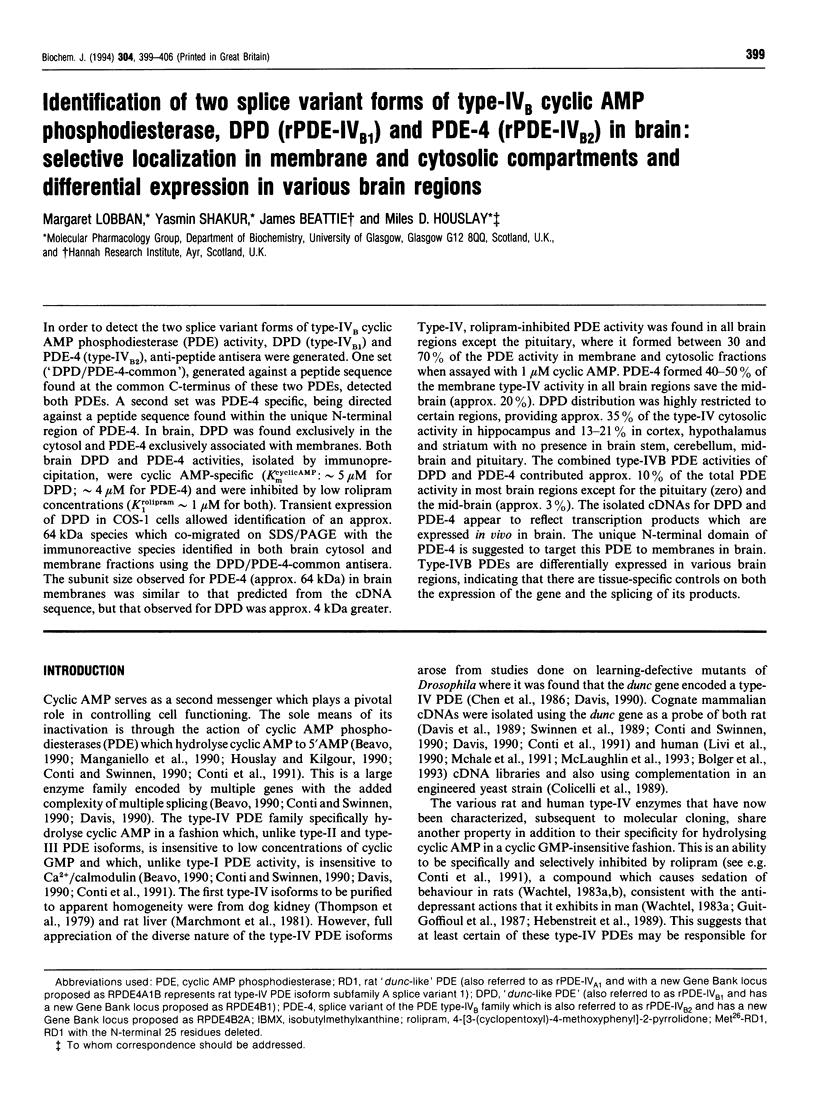
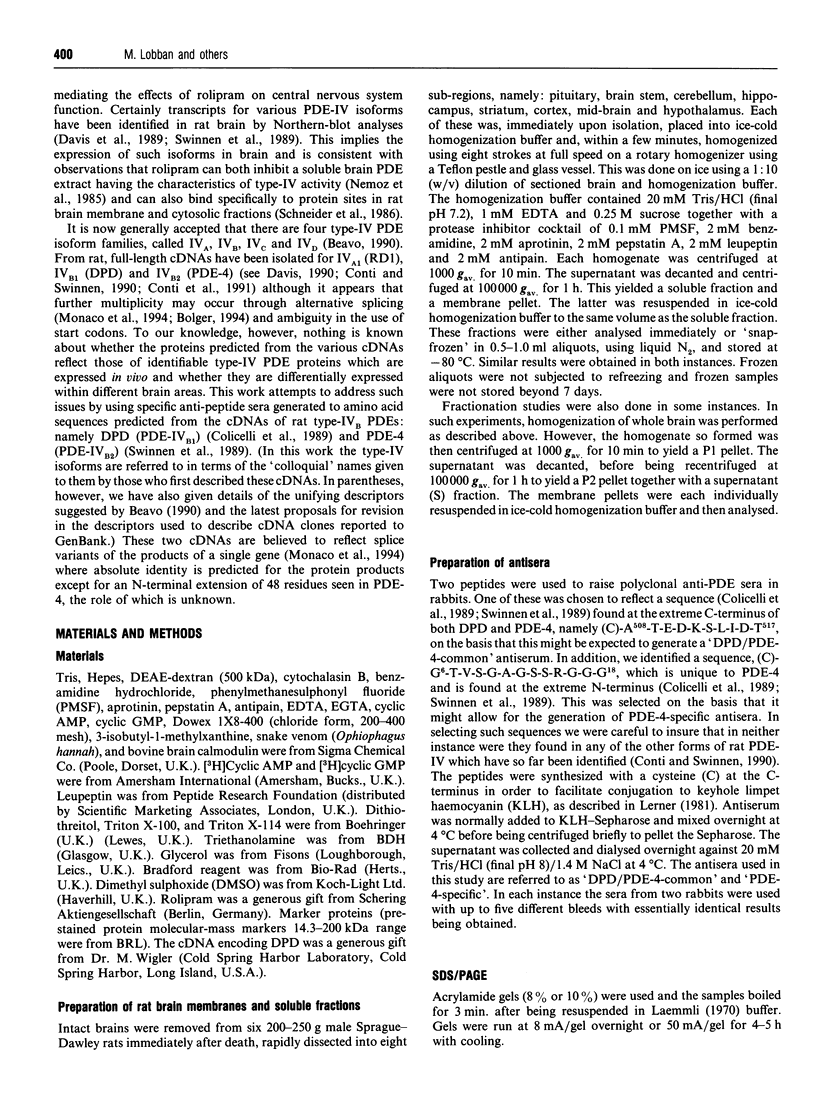
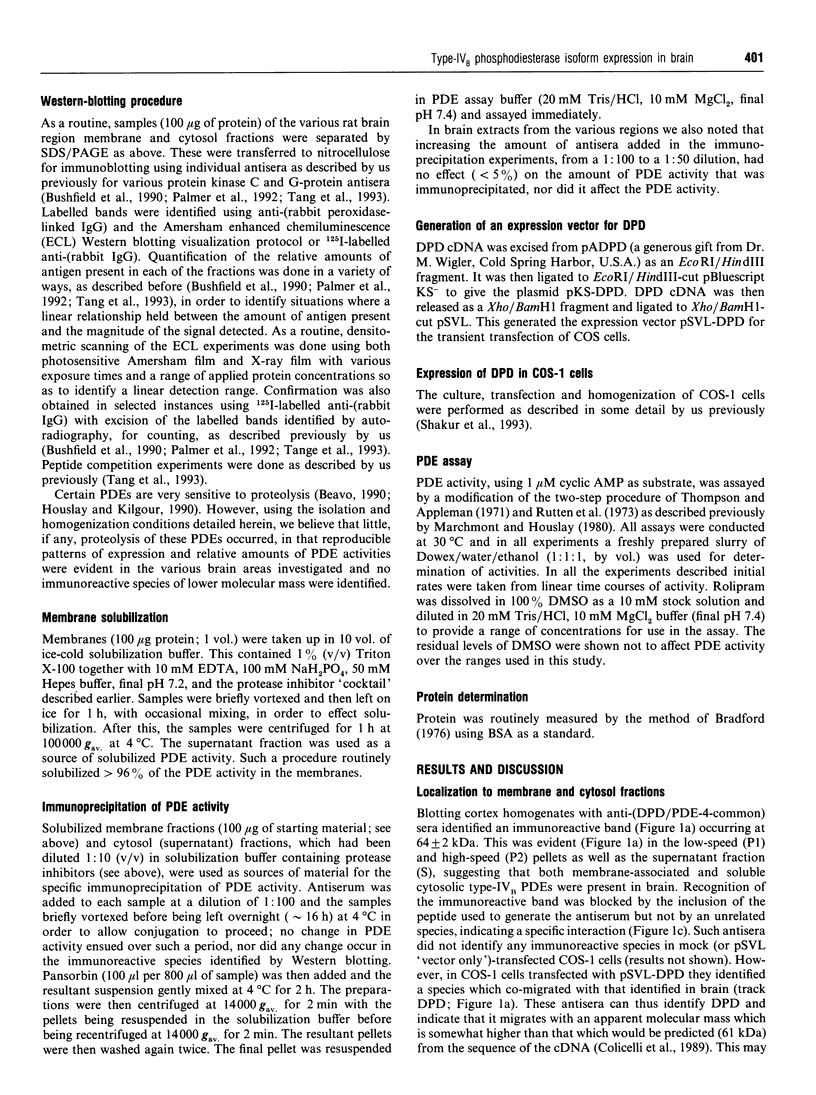
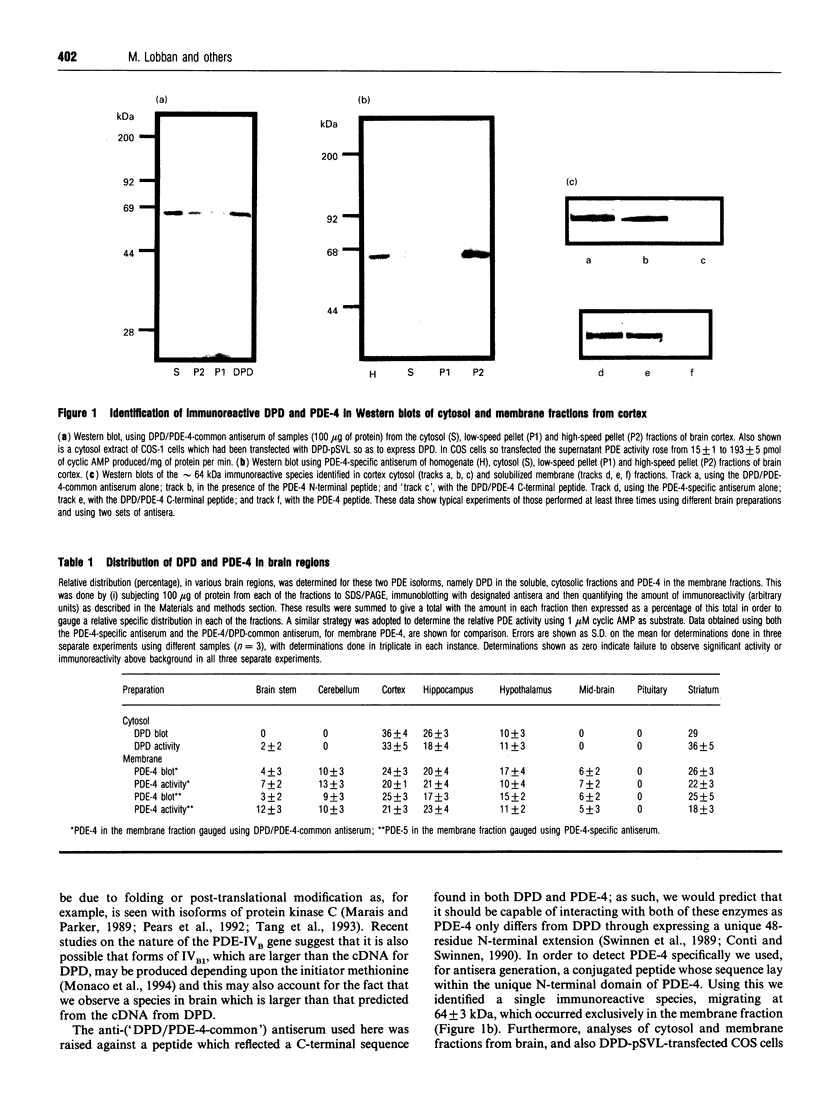
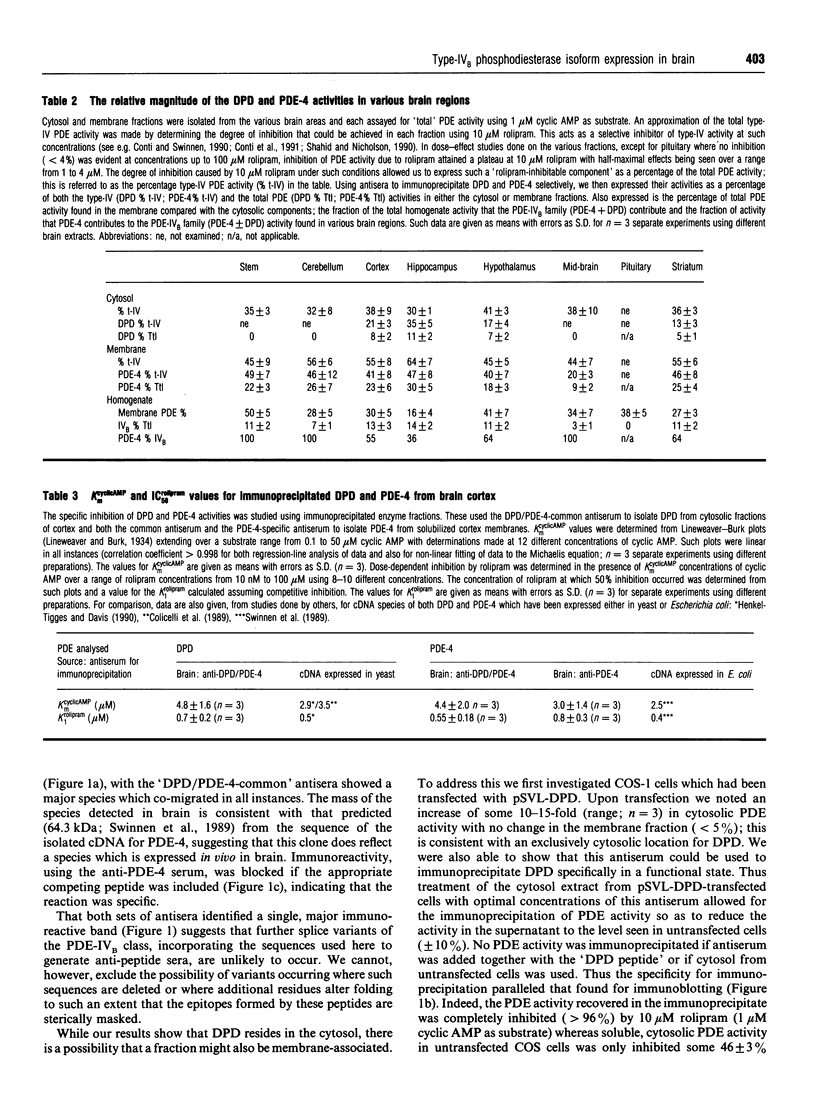
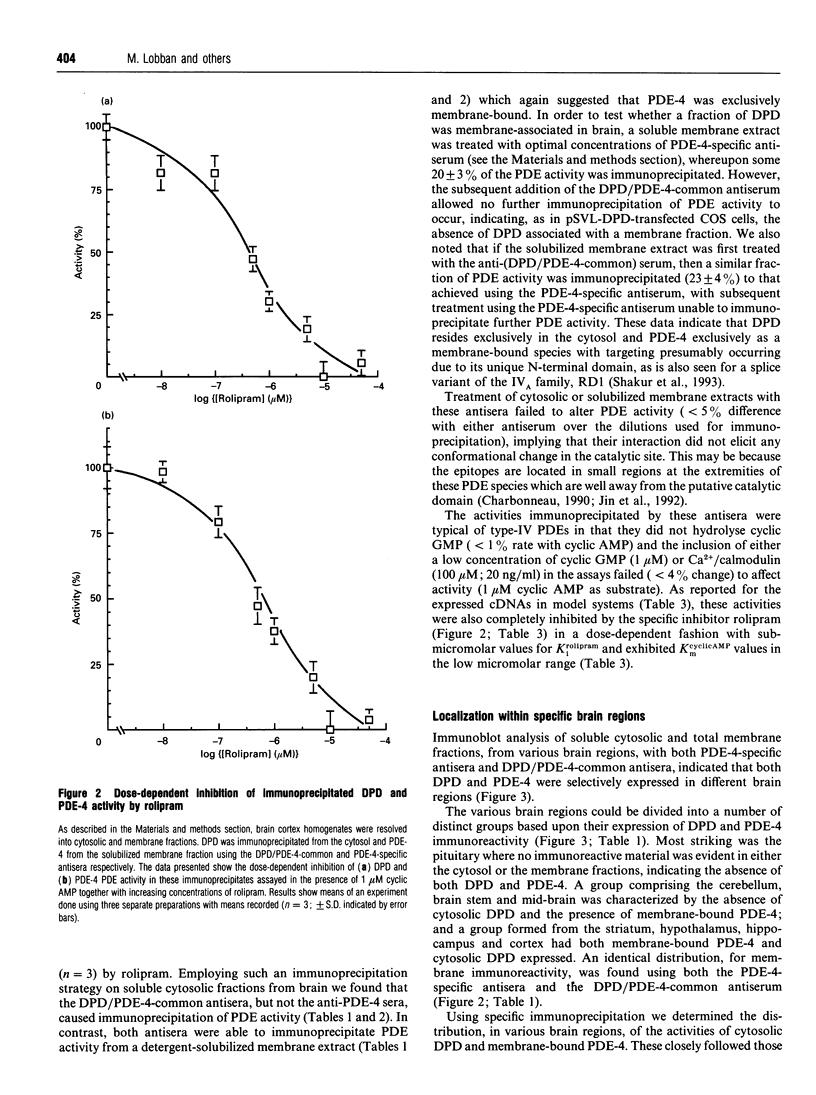
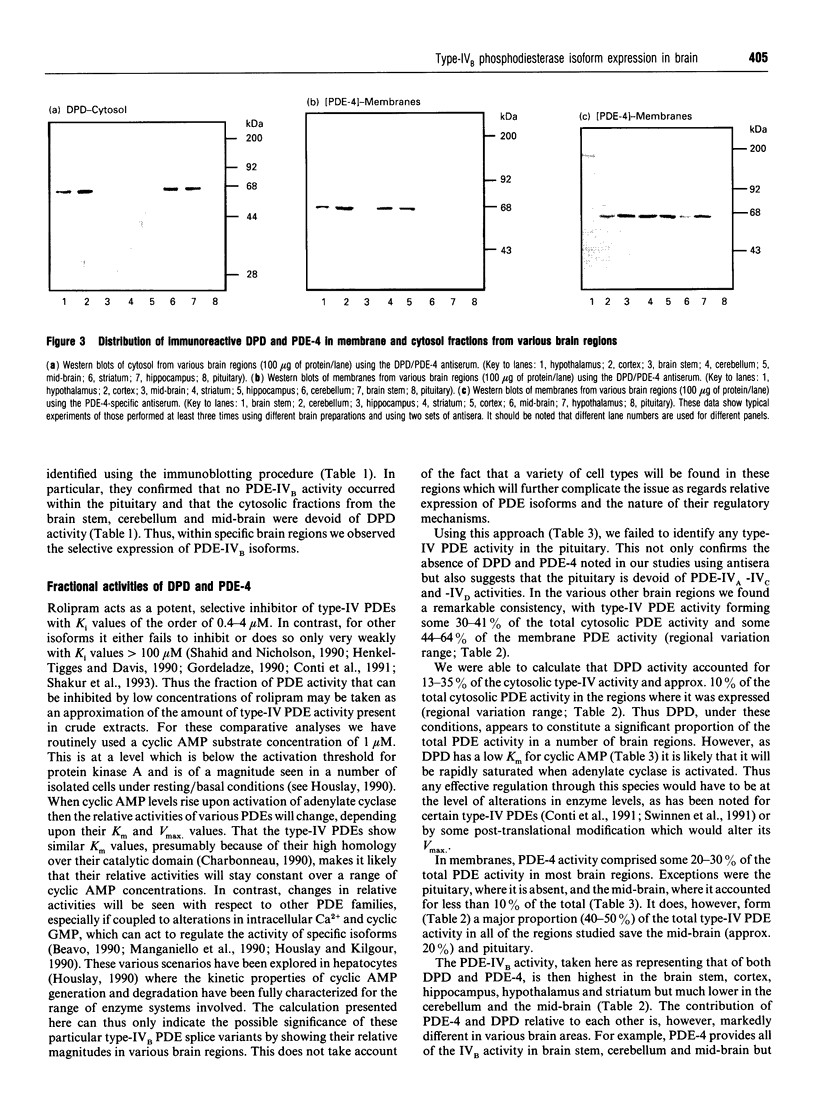
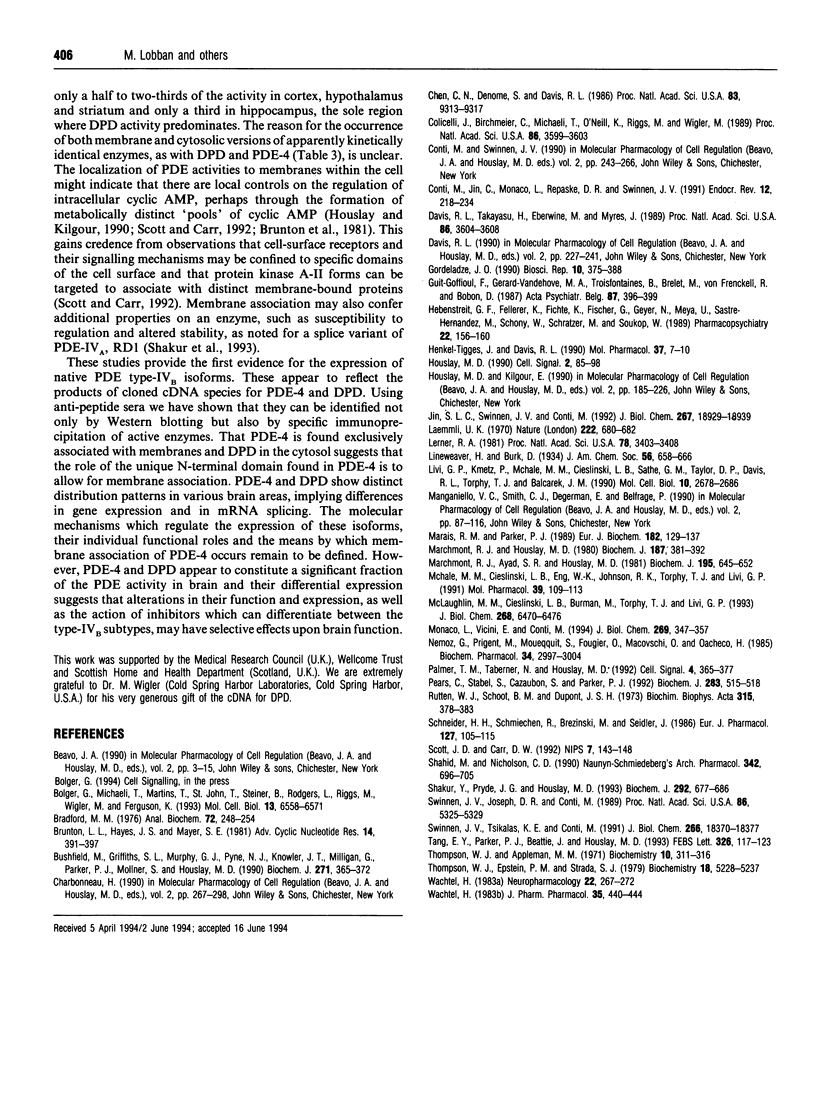
Images in this article
Selected References
These references are in PubMed. This may not be the complete list of references from this article.
- Bolger G., Michaeli T., Martins T., St John T., Steiner B., Rodgers L., Riggs M., Wigler M., Ferguson K. A family of human phosphodiesterases homologous to the dunce learning and memory gene product of Drosophila melanogaster are potential targets for antidepressant drugs. Mol Cell Biol. 1993 Oct;13(10):6558–6571. doi: 10.1128/mcb.13.10.6558. [DOI] [PMC free article] [PubMed] [Google Scholar]
- Bradford M. M. A rapid and sensitive method for the quantitation of microgram quantities of protein utilizing the principle of protein-dye binding. Anal Biochem. 1976 May 7;72:248–254. doi: 10.1016/0003-2697(76)90527-3. [DOI] [PubMed] [Google Scholar]
- Brunton L. L., Hayes J. S., Mayer S. E. Functional compartmentation of cyclic AMP and protein kinase in heart. Adv Cyclic Nucleotide Res. 1981;14:391–397. [PubMed] [Google Scholar]
- Bushfield M., Griffiths S. L., Murphy G. J., Pyne N. J., Knowler J. T., Milligan G., Parker P. J., Mollner S., Houslay M. D. Diabetes-induced alterations in the expression, functioning and phosphorylation state of the inhibitory guanine nucleotide regulatory protein Gi-2 in hepatocytes. Biochem J. 1990 Oct 15;271(2):365–372. doi: 10.1042/bj2710365. [DOI] [PMC free article] [PubMed] [Google Scholar]
- Chen C. N., Denome S., Davis R. L. Molecular analysis of cDNA clones and the corresponding genomic coding sequences of the Drosophila dunce+ gene, the structural gene for cAMP phosphodiesterase. Proc Natl Acad Sci U S A. 1986 Dec;83(24):9313–9317. doi: 10.1073/pnas.83.24.9313. [DOI] [PMC free article] [PubMed] [Google Scholar]
- Colicelli J., Birchmeier C., Michaeli T., O'Neill K., Riggs M., Wigler M. Isolation and characterization of a mammalian gene encoding a high-affinity cAMP phosphodiesterase. Proc Natl Acad Sci U S A. 1989 May;86(10):3599–3603. doi: 10.1073/pnas.86.10.3599. [DOI] [PMC free article] [PubMed] [Google Scholar]
- Davis R. L., Takayasu H., Eberwine M., Myres J. Cloning and characterization of mammalian homologs of the Drosophila dunce+ gene. Proc Natl Acad Sci U S A. 1989 May;86(10):3604–3608. doi: 10.1073/pnas.86.10.3604. [DOI] [PMC free article] [PubMed] [Google Scholar]
- Gordeladze J. O. The pharmacodynamic action of the cyclic AMP phosphodiesterase inhibitor rolipram on prolactin producing rat pituitary adenoma (GH4C1) cells. Biosci Rep. 1990 Aug;10(4):375–388. doi: 10.1007/BF01117237. [DOI] [PubMed] [Google Scholar]
- Hebenstreit G. F., Fellerer K., Fichte K., Fischer G., Geyer N., Meya U., Sastre-y-Hernández M., Schöny W., Schratzer M., Soukop W. Rolipram in major depressive disorder: results of a double-blind comparative study with imipramine. Pharmacopsychiatry. 1989 Jul;22(4):156–160. doi: 10.1055/s-2007-1014599. [DOI] [PubMed] [Google Scholar]
- Henkel-Tigges J., Davis R. L. Rat homologs of the Drosophila dunce gene code for cyclic AMP phosphodiesterases sensitive to rolipram and RO 20-1724. Mol Pharmacol. 1990 Jan;37(1):7–10. [PubMed] [Google Scholar]
- Houslay M. D. The use of selective inhibitors and computer modelling to evaluate the role of specific high affinity cyclic AMP phosphodiesterases in the hormonal regulation of hepatocyte intracellular cyclic AMP concentrations. Cell Signal. 1990;2(1):85–98. doi: 10.1016/0898-6568(90)90036-a. [DOI] [PubMed] [Google Scholar]
- Jin S. L., Swinnen J. V., Conti M. Characterization of the structure of a low Km, rolipram-sensitive cAMP phosphodiesterase. Mapping of the catalytic domain. J Biol Chem. 1992 Sep 15;267(26):18929–18939. [PubMed] [Google Scholar]
- Laemmli U. K. Cleavage of structural proteins during the assembly of the head of bacteriophage T4. Nature. 1970 Aug 15;227(5259):680–685. doi: 10.1038/227680a0. [DOI] [PubMed] [Google Scholar]
- Lerner R. A., Green N., Alexander H., Liu F. T., Sutcliffe J. G., Shinnick T. M. Chemically synthesized peptides predicted from the nucleotide sequence of the hepatitis B virus genome elicit antibodies reactive with the native envelope protein of Dane particles. Proc Natl Acad Sci U S A. 1981 Jun;78(6):3403–3407. doi: 10.1073/pnas.78.6.3403. [DOI] [PMC free article] [PubMed] [Google Scholar]
- Livi G. P., Kmetz P., McHale M. M., Cieslinski L. B., Sathe G. M., Taylor D. P., Davis R. L., Torphy T. J., Balcarek J. M. Cloning and expression of cDNA for a human low-Km, rolipram-sensitive cyclic AMP phosphodiesterase. Mol Cell Biol. 1990 Jun;10(6):2678–2686. doi: 10.1128/mcb.10.6.2678. [DOI] [PMC free article] [PubMed] [Google Scholar]
- Marais R. M., Parker P. J. Purification and characterisation of bovine brain protein kinase C isotypes alpha, beta and gamma. Eur J Biochem. 1989 Jun 1;182(1):129–137. doi: 10.1111/j.1432-1033.1989.tb14809.x. [DOI] [PubMed] [Google Scholar]
- Marchmont R. J., Ayad S. R., Houslay M. D. Purification and properties of the insulin-stimulated cyclic AMP phosphodiesterase from rat liver plasma membranes. Biochem J. 1981 Jun 1;195(3):645–652. doi: 10.1042/bj1950645. [DOI] [PMC free article] [PubMed] [Google Scholar]
- Marchmont R. J., Houslay M. D. A peripheral and an intrinsic enzyme constitute the cyclic AMP phosphodiesterase activity of rat liver plasma membranes. Biochem J. 1980 May 1;187(2):381–392. doi: 10.1042/bj1870381. [DOI] [PMC free article] [PubMed] [Google Scholar]
- McHale M. M., Cieslinski L. B., Eng W. K., Johnson R. K., Torphy T. J., Livi G. P. Expression of human recombinant cAMP phosphodiesterase isozyme IV reverses growth arrest phenotypes in phosphodiesterase-deficient yeast. Mol Pharmacol. 1991 Feb;39(2):109–113. [PubMed] [Google Scholar]
- McLaughlin M. M., Cieslinski L. B., Burman M., Torphy T. J., Livi G. P. A low-Km, rolipram-sensitive, cAMP-specific phosphodiesterase from human brain. Cloning and expression of cDNA, biochemical characterization of recombinant protein, and tissue distribution of mRNA. J Biol Chem. 1993 Mar 25;268(9):6470–6476. [PubMed] [Google Scholar]
- Monaco L., Vicini E., Conti M. Structure of two rat genes coding for closely related rolipram-sensitive cAMP phosphodiesterases. Multiple mRNA variants originate from alternative splicing and multiple start sites. J Biol Chem. 1994 Jan 7;269(1):347–357. [PubMed] [Google Scholar]
- Nemoz G., Prigent A. F., Moueqqit M., Fougier S., Macovschi O., Pacheco H. Selective inhibition of one of the cyclic AMP phosphodiesterases from rat brain by the neurotropic compound rolipram. Biochem Pharmacol. 1985 Aug 15;34(16):2997–3000. doi: 10.1016/0006-2952(85)90029-2. [DOI] [PubMed] [Google Scholar]
- Palmer T. M., Taberner P. V., Houslay M. D. Alterations in G-protein expression, Gi function and stimulatory receptor-mediated regulation of adipocyte adenylyl cyclase in a model of insulin-resistant diabetes with obesity. Cell Signal. 1992 Jul;4(4):365–377. doi: 10.1016/0898-6568(92)90031-3. [DOI] [PubMed] [Google Scholar]
- Pears C., Stabel S., Cazaubon S., Parker P. J. Studies on the phosphorylation of protein kinase C-alpha. Biochem J. 1992 Apr 15;283(Pt 2):515–518. doi: 10.1042/bj2830515. [DOI] [PMC free article] [PubMed] [Google Scholar]
- Schneider H. H., Schmiechen R., Brezinski M., Seidler J. Stereospecific binding of the antidepressant rolipram to brain protein structures. Eur J Pharmacol. 1986 Aug 7;127(1-2):105–115. doi: 10.1016/0014-2999(86)90210-4. [DOI] [PubMed] [Google Scholar]
- Shakur Y., Pryde J. G., Houslay M. D. Engineered deletion of the unique N-terminal domain of the cyclic AMP-specific phosphodiesterase RD1 prevents plasma membrane association and the attainment of enhanced thermostability without altering its sensitivity to inhibition by rolipram. Biochem J. 1993 Jun 15;292(Pt 3):677–686. doi: 10.1042/bj2920677. [DOI] [PMC free article] [PubMed] [Google Scholar]
- Swinnen J. V., Joseph D. R., Conti M. Molecular cloning of rat homologues of the Drosophila melanogaster dunce cAMP phosphodiesterase: evidence for a family of genes. Proc Natl Acad Sci U S A. 1989 Jul;86(14):5325–5329. doi: 10.1073/pnas.86.14.5325. [DOI] [PMC free article] [PubMed] [Google Scholar]
- Swinnen J. V., Tsikalas K. E., Conti M. Properties and hormonal regulation of two structurally related cAMP phosphodiesterases from the rat Sertoli cell. J Biol Chem. 1991 Sep 25;266(27):18370–18377. [PubMed] [Google Scholar]
- Tang E. Y., Parker P. J., Beattie J., Houslay M. D. Diabetes induces selective alterations in the expression of protein kinase C isoforms in hepatocytes. FEBS Lett. 1993 Jul 12;326(1-3):117–123. doi: 10.1016/0014-5793(93)81774-t. [DOI] [PubMed] [Google Scholar]
- Thompson W. J., Appleman M. M. Multiple cyclic nucleotide phosphodiesterase activities from rat brain. Biochemistry. 1971 Jan 19;10(2):311–316. [PubMed] [Google Scholar]
- Thompson W. J., Epstein P. M., Strada S. J. Purification and characterization of high-affinity cyclic adenosine monophosphate phosphodiesterase from dog kidney. Biochemistry. 1979 Nov 13;18(23):5228–5237. doi: 10.1021/bi00590a030. [DOI] [PubMed] [Google Scholar]
- Wachtel H. Neurotropic effects of the optical isomers of the selective adenosine cyclic 3',5'-monophosphate phosphodiesterase inhibitor rolipram in rats in-vivo. J Pharm Pharmacol. 1983 Jul;35(7):440–444. doi: 10.1111/j.2042-7158.1983.tb04318.x. [DOI] [PubMed] [Google Scholar]
- Wachtel H. Potential antidepressant activity of rolipram and other selective cyclic adenosine 3',5'-monophosphate phosphodiesterase inhibitors. Neuropharmacology. 1983 Mar;22(3):267–272. doi: 10.1016/0028-3908(83)90239-3. [DOI] [PubMed] [Google Scholar]