Abstract
The transport of Pi between the cytosol and the mitochondria was investigated in perfused rat liver stimulated with phenylephrine and metabolic precursors of glucose and urea: pyruvate, lactate, NH4+ and ornithine. The relative concentrations of phosphorus metabolites in the liver were measured by 31P-n.m.r. spectroscopy. When added simultaneously, phenylephrine and the precursors induced a decrease in the Pi level which in 4-5 min reached a new steady state at 73% of the control level. After 5 min or more of stimulation the ATP level had also decreased. When the stimulation ended, Pi and ATP returned to their initial levels within 15 min. In mitochondria isolated after 5 min of stimulation, Pi was increased more than 2-fold as compared with control mitochondria and, in addition, an accumulation of Pi from the perfusion buffer into the liver was observed. Phenylephrine by itself did not cause any significant changes in the ATP or Pi levels, whereas the glucose and urea precursors in the absence of phenylephrine induced a 9% decrease in Pi, while ATP remained constant. The Pi content of mitochondria isolated under these conditions was not significantly increased as compared with control mitochondria. These results showed that Pi accumulated into the mitochondria by a mechanism possibly involving exchange for malate, and that a major part of the intramitochondrial Pi was invisible by n.m.r.
Full text
PDF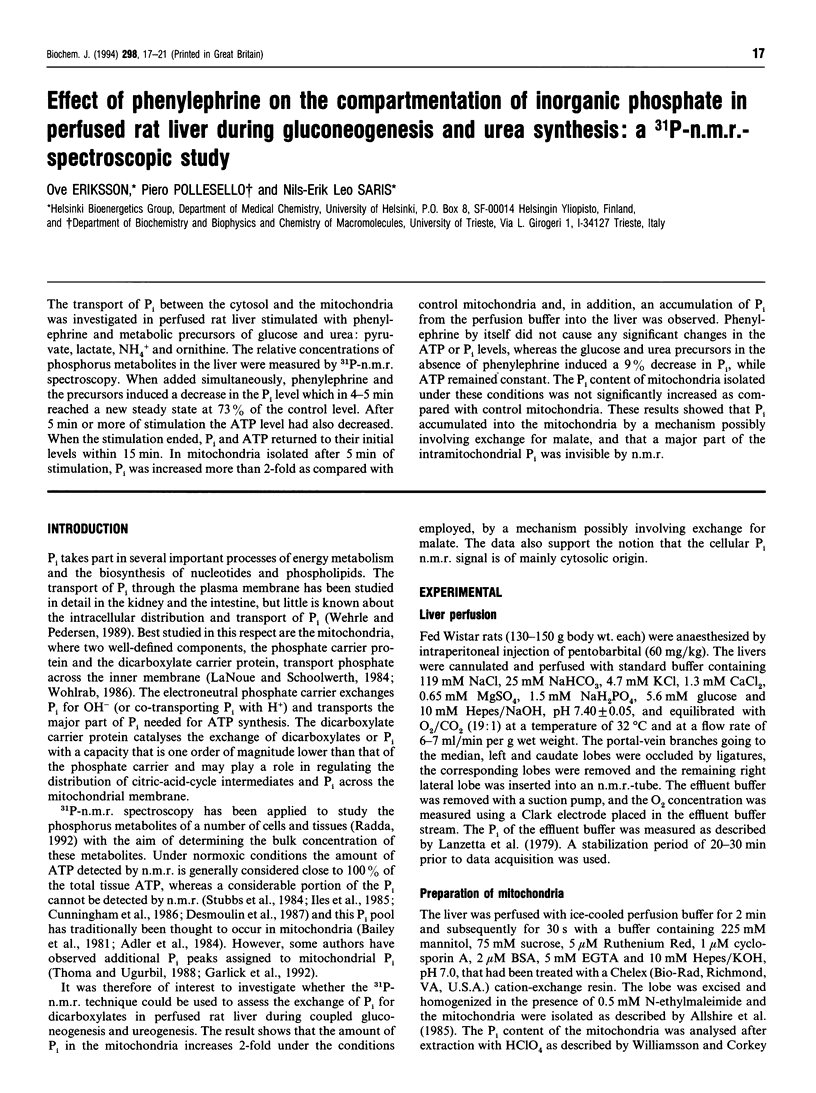
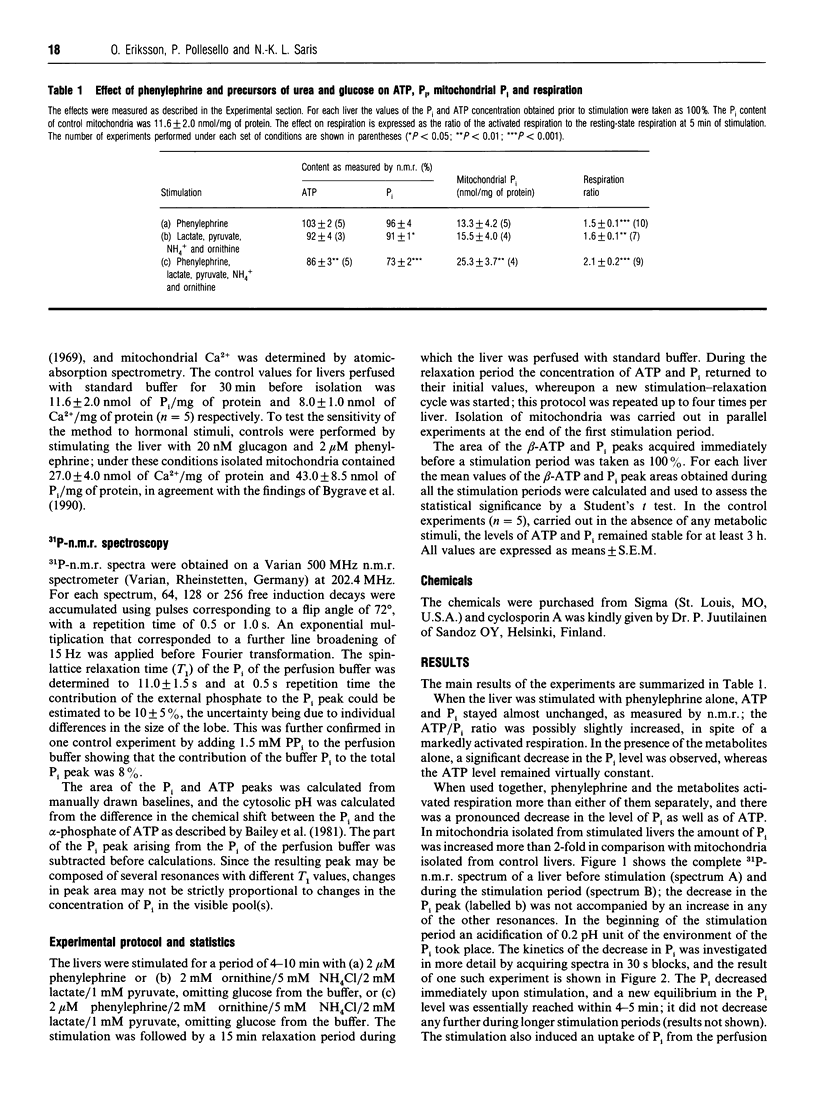
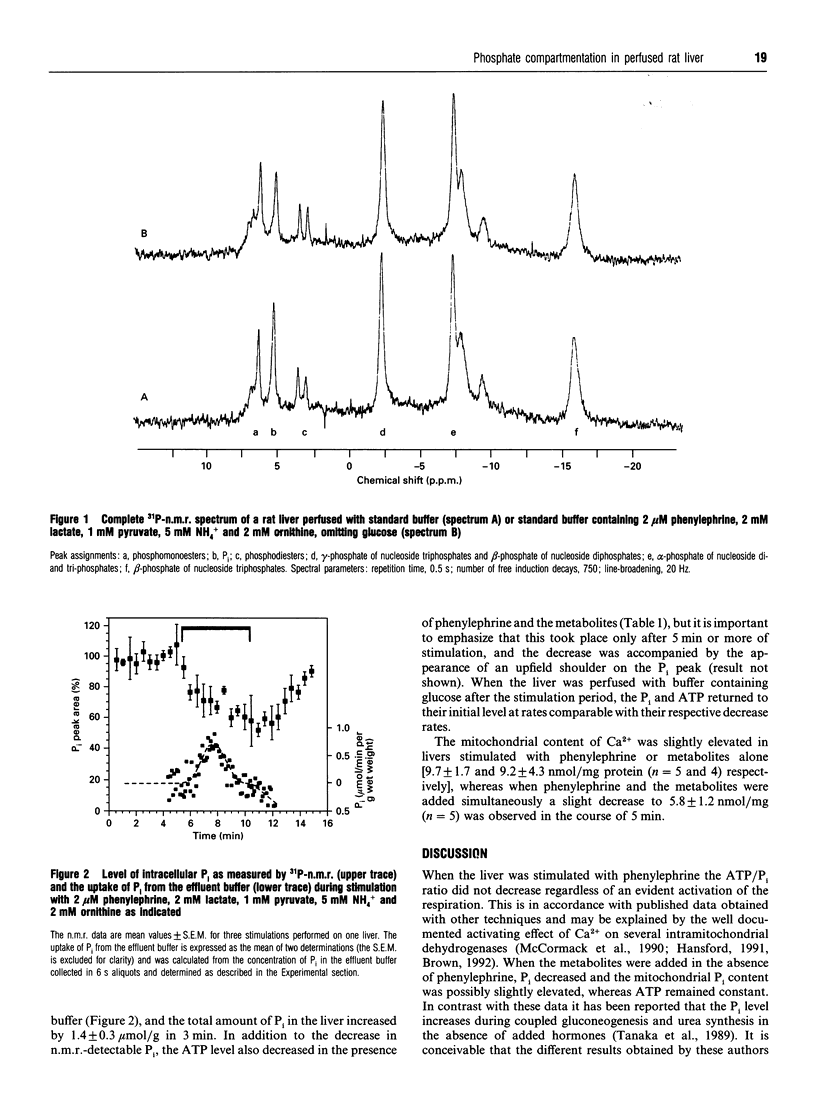
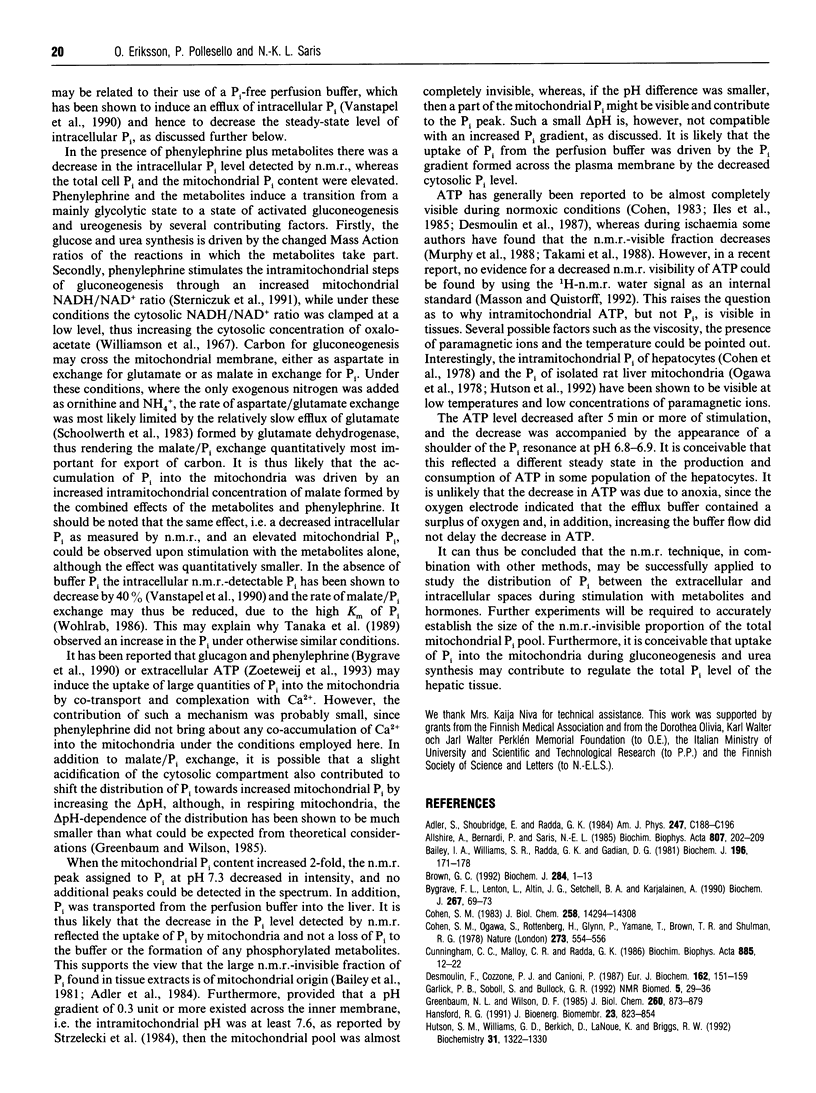
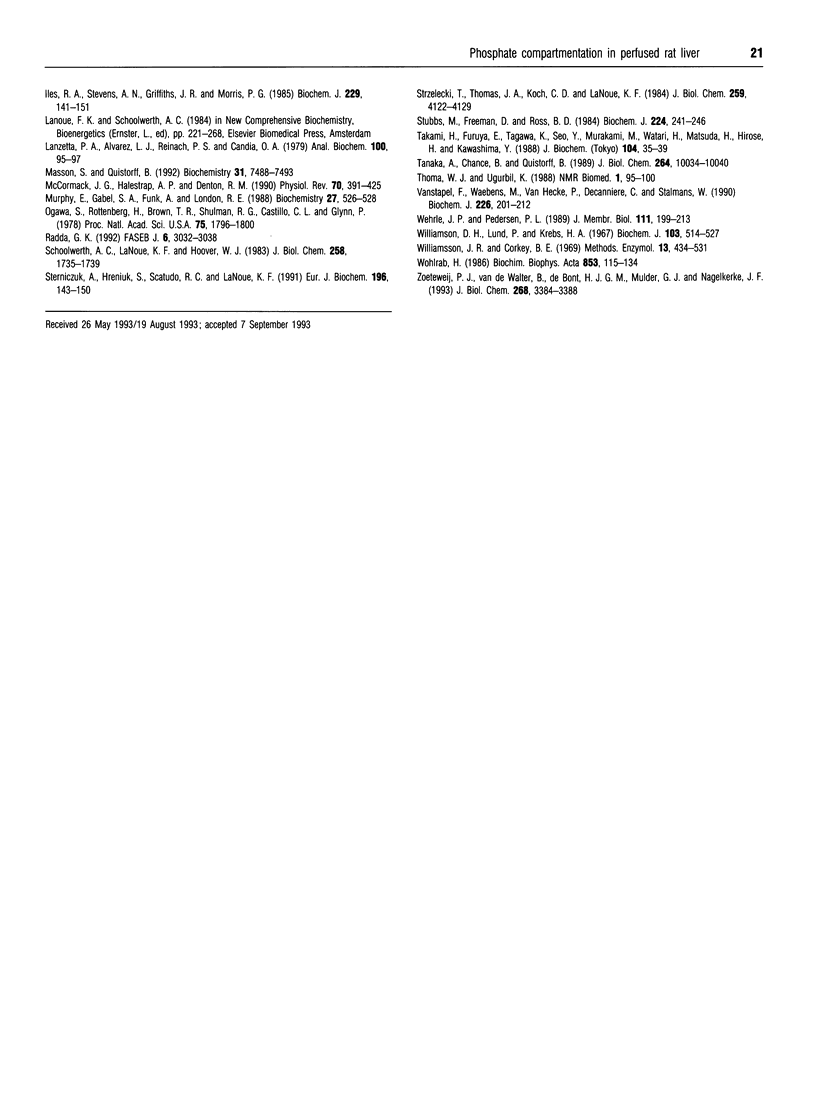
Selected References
These references are in PubMed. This may not be the complete list of references from this article.
- Adler S., Shoubridge E., Radda G. K. Estimation of cellular pH gradients with 31P-NMR in intact rabbit renal tubular cells. Am J Physiol. 1984 Sep;247(3 Pt 1):C188–C196. doi: 10.1152/ajpcell.1984.247.3.C188. [DOI] [PubMed] [Google Scholar]
- Allshire A., Bernardi P., Saris N. E. Manganese stimulates calcium flux through the mitochondrial uniporter. Biochim Biophys Acta. 1985 May 3;807(2):202–209. doi: 10.1016/0005-2728(85)90123-9. [DOI] [PubMed] [Google Scholar]
- Bailey I. A., Williams S. R., Radda G. K., Gadian D. G. Activity of phosphorylase in total global ischaemia in the rat heart. A phosphorus-31 nuclear-magnetic-resonance study. Biochem J. 1981 Apr 15;196(1):171–178. doi: 10.1042/bj1960171. [DOI] [PMC free article] [PubMed] [Google Scholar]
- Brown G. C. Control of respiration and ATP synthesis in mammalian mitochondria and cells. Biochem J. 1992 May 15;284(Pt 1):1–13. doi: 10.1042/bj2840001. [DOI] [PMC free article] [PubMed] [Google Scholar]
- Bygrave F. L., Lenton L., Altin J. G., Setchell B. A., Karjalainen A. Phosphate and calcium uptake by mitochondria and by perfused rat liver induced by the synergistic action of glucagon and vasopressin. Biochem J. 1990 Apr 1;267(1):69–73. doi: 10.1042/bj2670069. [DOI] [PMC free article] [PubMed] [Google Scholar]
- Cohen S. M., Ogawa S., Rottenberg H., Glynn P., Yamane T., Brown T. R., Shulman R. G. P nuclear magnetic resonance studies of isolated rat liver cells. Nature. 1978 Jun 15;273(5663):554–556. doi: 10.1038/273554a0. [DOI] [PubMed] [Google Scholar]
- Cohen S. M. Simultaneous 13C and 31P NMR studies of perfused rat liver. Effects of insulin and glucagon and a 13C NMR assay of free Mg2+. J Biol Chem. 1983 Dec 10;258(23):14294–14308. [PubMed] [Google Scholar]
- Cunningham C. C., Malloy C. R., Radda G. K. Effect of fasting and acute ethanol administration on the energy state of in vivo liver as measured by 31P-NMR spectroscopy. Biochim Biophys Acta. 1986 Jan 23;885(1):12–22. doi: 10.1016/0167-4889(86)90033-9. [DOI] [PubMed] [Google Scholar]
- Desmoulin F., Cozzone P. J., Canioni P. Phosphorus-31 nuclear-magnetic-resonance study of phosphorylated metabolites compartmentation, intracellular pH and phosphorylation state during normoxia, hypoxia and ethanol perfusion, in the perfused rat liver. Eur J Biochem. 1987 Jan 2;162(1):151–159. doi: 10.1111/j.1432-1033.1987.tb10555.x. [DOI] [PubMed] [Google Scholar]
- Garlick P. B., Soboll S., Bullock G. R. Evidence that mitochondrial phosphate is visible in 31P NMR spectra of isolated, perfused rat hearts. NMR Biomed. 1992 Jan-Feb;5(1):29–36. doi: 10.1002/nbm.1940050106. [DOI] [PubMed] [Google Scholar]
- Greenbaum N. L., Wilson D. F. The distribution of inorganic phosphate and malate between intra- and extramitochondrial spaces. Relationship with the transmembrane pH difference. J Biol Chem. 1985 Jan 25;260(2):873–879. [PubMed] [Google Scholar]
- Hansford R. G. Dehydrogenase activation by Ca2+ in cells and tissues. J Bioenerg Biomembr. 1991 Dec;23(6):823–854. doi: 10.1007/BF00786004. [DOI] [PubMed] [Google Scholar]
- Iles R. A., Stevens A. N., Griffiths J. R., Morris P. G. Phosphorylation status of liver by 31P-n.m.r. spectroscopy, and its implications for metabolic control. A comparison of 31P-n.m.r. spectroscopy (in vivo and in vitro) with chemical and enzymic determinations of ATP, ADP and Pi. Biochem J. 1985 Jul 1;229(1):141–151. doi: 10.1042/bj2290141. [DOI] [PMC free article] [PubMed] [Google Scholar]
- Lanzetta P. A., Alvarez L. J., Reinach P. S., Candia O. A. An improved assay for nanomole amounts of inorganic phosphate. Anal Biochem. 1979 Nov 15;100(1):95–97. doi: 10.1016/0003-2697(79)90115-5. [DOI] [PubMed] [Google Scholar]
- Masson S., Quistorff B. The 31P NMR visibility of ATP in perfused rat liver remains about 90%, unaffected by changes of metabolic state. Biochemistry. 1992 Aug 25;31(33):7488–7493. doi: 10.1021/bi00148a008. [DOI] [PubMed] [Google Scholar]
- McCormack J. G., Halestrap A. P., Denton R. M. Role of calcium ions in regulation of mammalian intramitochondrial metabolism. Physiol Rev. 1990 Apr;70(2):391–425. doi: 10.1152/physrev.1990.70.2.391. [DOI] [PubMed] [Google Scholar]
- Murphy E., Gabel S. A., Funk A., London R. E. NMR observability of ATP: preferential depletion of cytosolic ATP during ischemia in perfused rat liver. Biochemistry. 1988 Jan 26;27(2):526–528. doi: 10.1021/bi00402a003. [DOI] [PubMed] [Google Scholar]
- Ogawa S., Rottenberg H., Brown T. R., Shulman R. G., Castillo C. L., Glynn P. High-resolution 31P nuclear magnetic resonance study of rat liver mitochondria. Proc Natl Acad Sci U S A. 1978 Apr;75(4):1796–1800. doi: 10.1073/pnas.75.4.1796. [DOI] [PMC free article] [PubMed] [Google Scholar]
- Radda G. K. Control, bioenergetics, and adaptation in health and disease: noninvasive biochemistry from nuclear magnetic resonance. FASEB J. 1992 Sep;6(12):3032–3038. doi: 10.1096/fasebj.6.12.1521736. [DOI] [PubMed] [Google Scholar]
- Schoolwerth A. C., LaNoue K. F., Hoover W. J. Glutamate transprot in rat kidney mitochondria. J Biol Chem. 1983 Feb 10;258(3):1735–1739. [PubMed] [Google Scholar]
- Sterniczuk A., Hreniuk S., Scaduto R. C., Jr, LaNoue K. F. The mechanism of Ca2(+)-related control of gluconeogenesis in perfused liver. Eur J Biochem. 1991 Feb 26;196(1):143–150. doi: 10.1111/j.1432-1033.1991.tb15797.x. [DOI] [PubMed] [Google Scholar]
- Strzelecki T., Thomas J. A., Koch C. D., LaNoue K. F. The effect of hormones on proton compartmentation in hepatocytes. J Biol Chem. 1984 Apr 10;259(7):4122–4129. [PubMed] [Google Scholar]
- Stubbs M., Freeman D., Ross B. D. Formation of n.m.r.-invisible ADP during renal ischaemia in rats. Biochem J. 1984 Nov 15;224(1):241–246. doi: 10.1042/bj2240241. [DOI] [PMC free article] [PubMed] [Google Scholar]
- Takami H., Furuya E., Tagawa K., Seo Y., Murakami M., Watari H., Matsuda H., Hirose H., Kawashima Y. NMR-invisible ATP in rat heart and its change in ischemia. J Biochem. 1988 Jul;104(1):35–39. doi: 10.1093/oxfordjournals.jbchem.a122417. [DOI] [PubMed] [Google Scholar]
- Tanaka A., Chance B., Quistorff B. A possible role of inorganic phosphate as a regulator of oxidative phosphorylation in combined urea synthesis and gluconeogenesis in perfused rat liver. A phosphorus magnetic resonance spectroscopy study. J Biol Chem. 1989 Jun 15;264(17):10034–10040. [PubMed] [Google Scholar]
- Thoma W. J., Uğurbil K. pH and compartmentation of isolated perfused rat liver studied by 31P and 19F NMR. NMR Biomed. 1988 Apr;1(2):95–100. doi: 10.1002/nbm.1940010207. [DOI] [PubMed] [Google Scholar]
- Vanstapel F., Waebens M., Van Hecke P., Decanniere C., Stalmans W. The cytosolic concentration of phosphate determines the maximal rate of glycogenolysis in perfused rat liver. Biochem J. 1990 Feb 15;266(1):207–212. doi: 10.1042/bj2660207. [DOI] [PMC free article] [PubMed] [Google Scholar]
- Wehrle J. P., Pedersen P. L. Phosphate transport processes in eukaryotic cells. J Membr Biol. 1989 Nov;111(3):199–213. doi: 10.1007/BF01871006. [DOI] [PubMed] [Google Scholar]
- Williamson D. H., Lund P., Krebs H. A. The redox state of free nicotinamide-adenine dinucleotide in the cytoplasm and mitochondria of rat liver. Biochem J. 1967 May;103(2):514–527. doi: 10.1042/bj1030514. [DOI] [PMC free article] [PubMed] [Google Scholar]
- Wohlrab H. Molecular aspects of inorganic phosphate transport in mitochondria. Biochim Biophys Acta. 1986;853(2):115–134. doi: 10.1016/0304-4173(86)90007-8. [DOI] [PubMed] [Google Scholar]
- Zoeteweij J. P., van de Water B., de Bont H. J., Mulder G. J., Nagelkerke J. F. Calcium-induced cytotoxicity in hepatocytes after exposure to extracellular ATP is dependent on inorganic phosphate. Effects on mitochondrial calcium. J Biol Chem. 1993 Feb 15;268(5):3384–3388. [PubMed] [Google Scholar]