Abstract
The role of cysteine residues in transport function of the glucose transporter GLUT1 was investigated by a mutagenesis-expression strategy. Each of the six cysteine residues was individually replaced by site-directed mutagenesis. Expression of the heterologous wild-type or mutant glucose transporters and transport measurements at two hexose concentrations (50 microM and 5 mM) were undertaken in Xenopus oocytes. The catalytic activity of GLUT1 was retained, despite substitution of each single cysteine residue, which indicated that no individual residue is essential for hexose transport. This finding questions the involvement of oligomerization or intramolecular stabilization by a single disulphide bond as a prerequisite for transporter activation under basal conditions. Application of the impermeant mercurial thiol-group-reactive reagent p-chloromercuribenzenesulphonate (pCMBS) to the external or internal surface of plasma membrane demonstrated that cysteine-429, within the sixth external loop, and cysteine-207, at the beginning of the large intracellular loop which connects transmembrane segments 6 and 7, are the residues which are involved in transport inhibition by impermeant thiol-group-reactive reagents from either side of the cell. These data support the predicted membrane topology of the transport protein by transport measurements. If residues other than the cysteines at positions 429 or 207 are exposed to either side of the plasma membrane by conformational changes, they do not contribute to the transport inhibition by pCMBS. Application of pCMBS to one side of the plasma membrane also inhibited transport from the opposite direction, most likely due to the hindrance of sugar-induced interconversion of transporter conformation.
Full text
PDF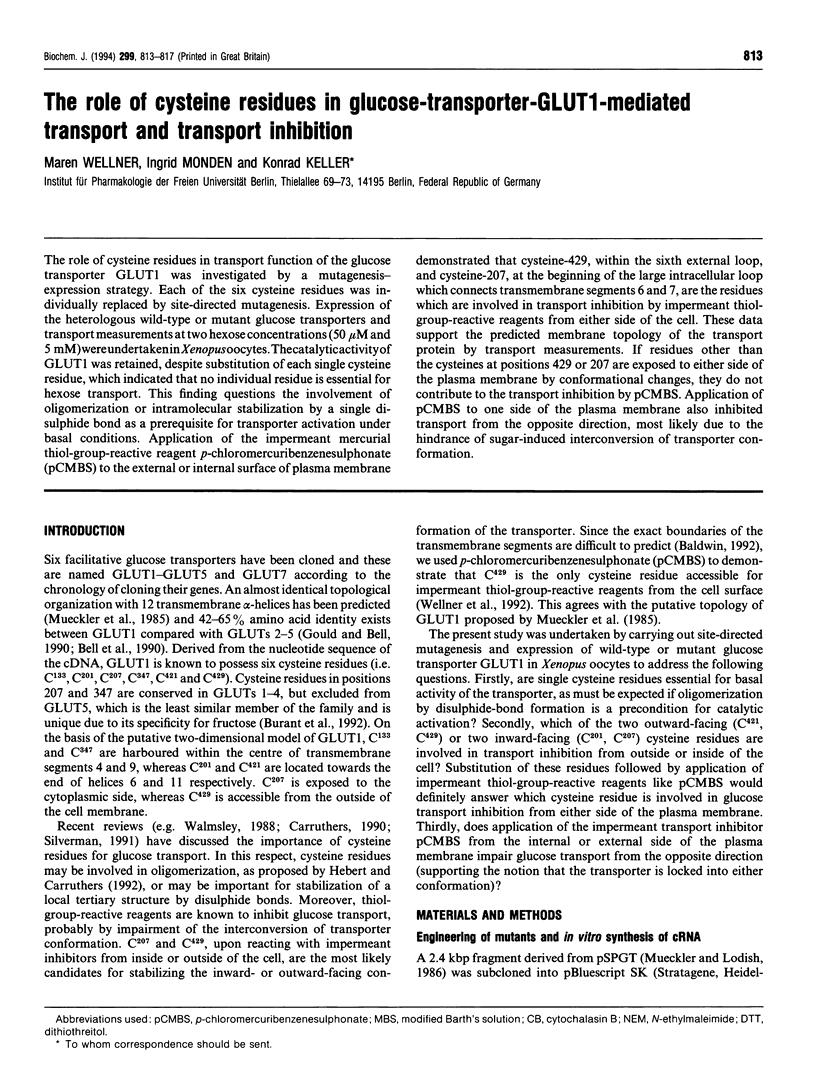
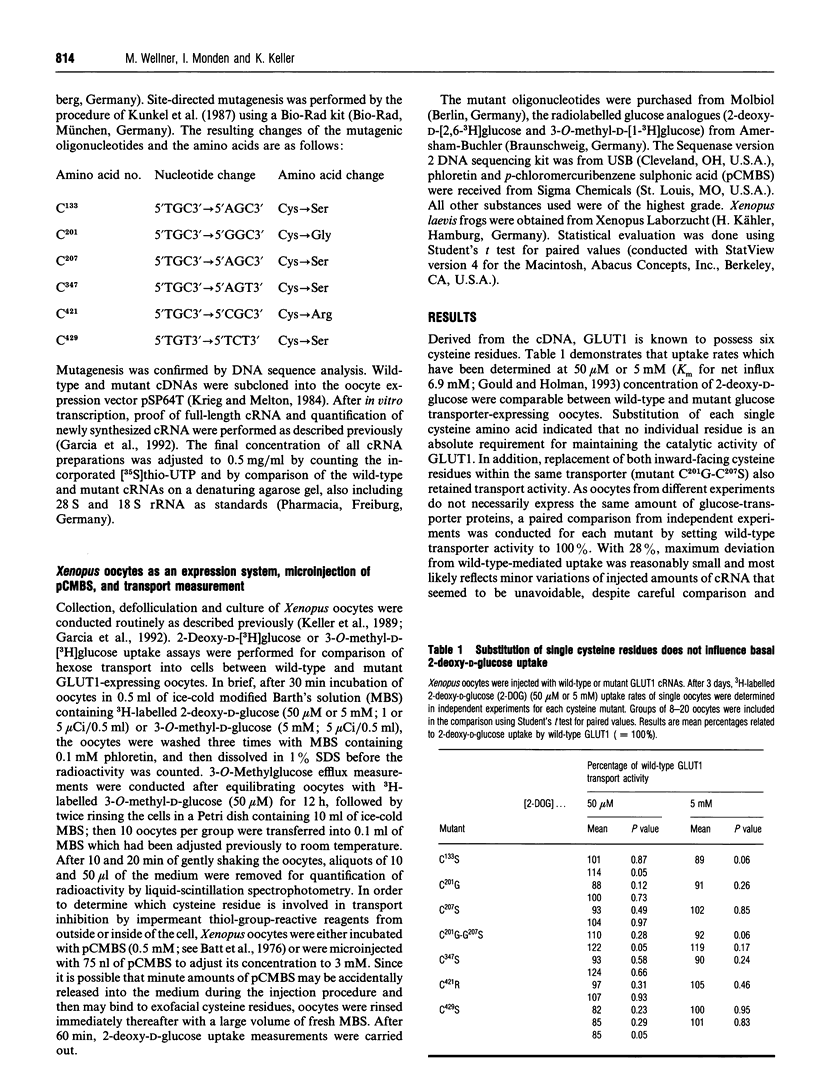
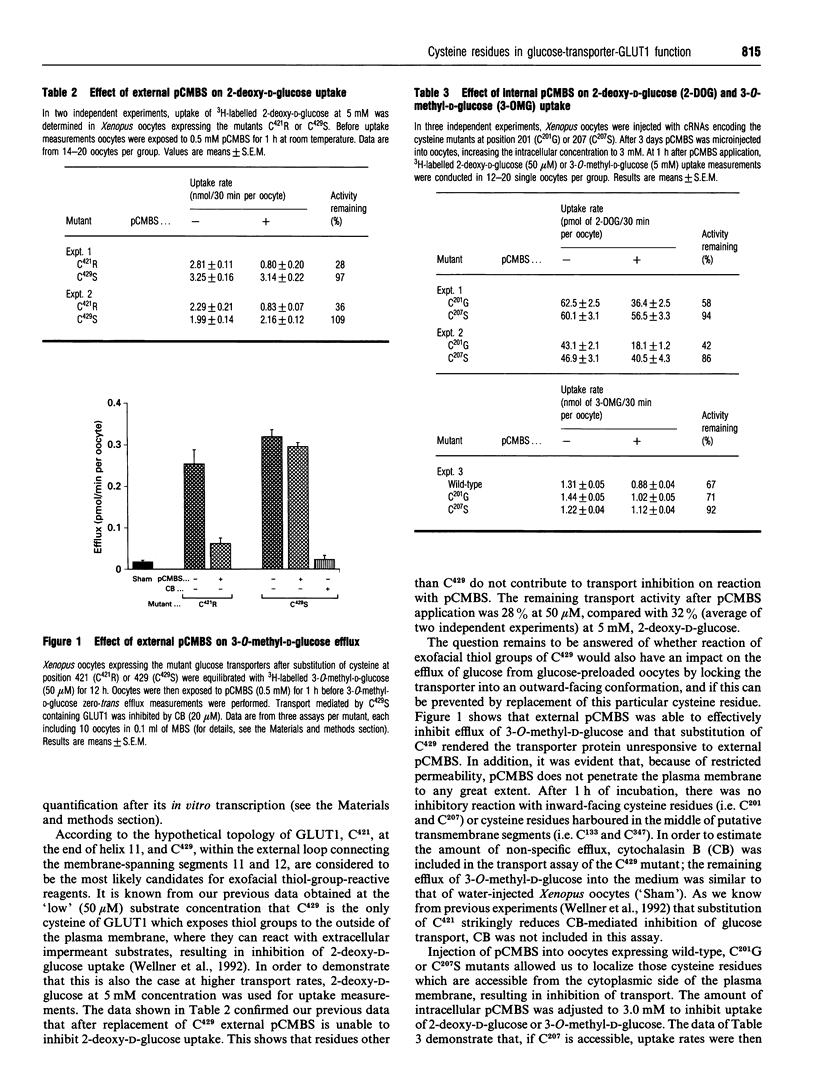
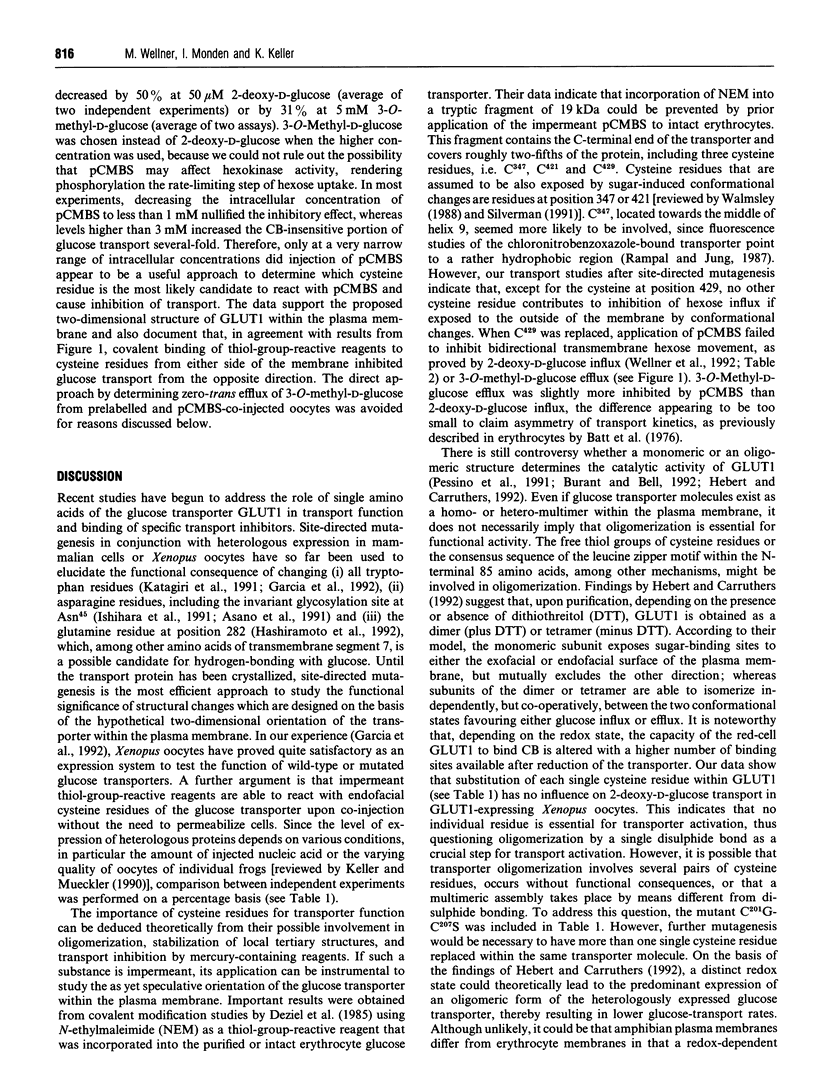
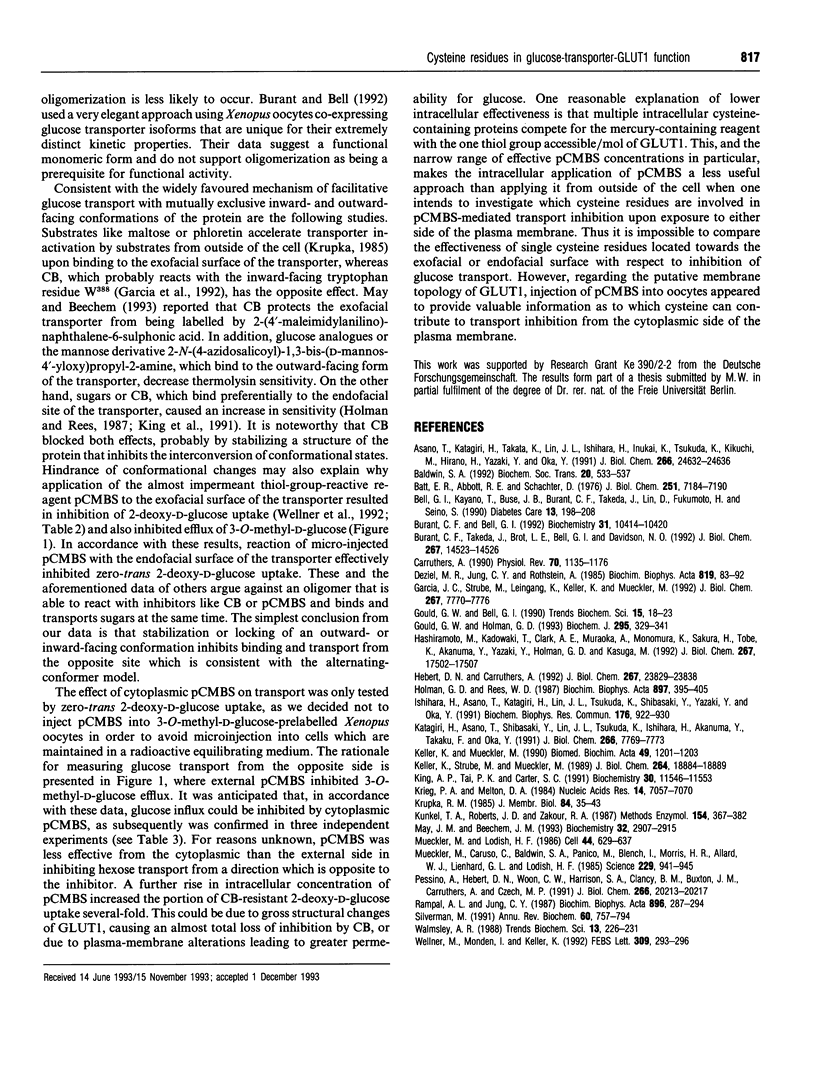
Selected References
These references are in PubMed. This may not be the complete list of references from this article.
- Asano T., Katagiri H., Takata K., Lin J. L., Ishihara H., Inukai K., Tsukuda K., Kikuchi M., Hirano H., Yazaki Y. The role of N-glycosylation of GLUT1 for glucose transport activity. J Biol Chem. 1991 Dec 25;266(36):24632–24636. [PubMed] [Google Scholar]
- Baldwin S. A. Probing the structure and function of the human erythrocyte glucose transporter. Biochem Soc Trans. 1992 Aug;20(3):533–537. doi: 10.1042/bst0200533. [DOI] [PubMed] [Google Scholar]
- Batt E. R., Abbott R. E., Schachter D. Impermeant maleimides. Identification of an exofacial component of the human erythrocyte hexose transport mechanism. J Biol Chem. 1976 Nov 25;251(22):7184–7190. [PubMed] [Google Scholar]
- Bell G. I., Kayano T., Buse J. B., Burant C. F., Takeda J., Lin D., Fukumoto H., Seino S. Molecular biology of mammalian glucose transporters. Diabetes Care. 1990 Mar;13(3):198–208. doi: 10.2337/diacare.13.3.198. [DOI] [PubMed] [Google Scholar]
- Burant C. F., Bell G. I. Mammalian facilitative glucose transporters: evidence for similar substrate recognition sites in functionally monomeric proteins. Biochemistry. 1992 Oct 27;31(42):10414–10420. doi: 10.1021/bi00157a032. [DOI] [PubMed] [Google Scholar]
- Burant C. F., Takeda J., Brot-Laroche E., Bell G. I., Davidson N. O. Fructose transporter in human spermatozoa and small intestine is GLUT5. J Biol Chem. 1992 Jul 25;267(21):14523–14526. [PubMed] [Google Scholar]
- Carruthers A. Facilitated diffusion of glucose. Physiol Rev. 1990 Oct;70(4):1135–1176. doi: 10.1152/physrev.1990.70.4.1135. [DOI] [PubMed] [Google Scholar]
- Deziel M. R., Jung C. Y., Rothstein A. The topology of the major band 4.5 protein component of the human erythrocyte membrane: characterization of reactive cysteine residues. Biochim Biophys Acta. 1985 Sep 25;819(1):83–92. doi: 10.1016/0005-2736(85)90198-1. [DOI] [PubMed] [Google Scholar]
- Garcia J. C., Strube M., Leingang K., Keller K., Mueckler M. M. Amino acid substitutions at tryptophan 388 and tryptophan 412 of the HepG2 (Glut1) glucose transporter inhibit transport activity and targeting to the plasma membrane in Xenopus oocytes. J Biol Chem. 1992 Apr 15;267(11):7770–7776. [PubMed] [Google Scholar]
- Gould G. W., Bell G. I. Facilitative glucose transporters: an expanding family. Trends Biochem Sci. 1990 Jan;15(1):18–23. doi: 10.1016/0968-0004(90)90125-u. [DOI] [PubMed] [Google Scholar]
- Gould G. W., Holman G. D. The glucose transporter family: structure, function and tissue-specific expression. Biochem J. 1993 Oct 15;295(Pt 2):329–341. doi: 10.1042/bj2950329. [DOI] [PMC free article] [PubMed] [Google Scholar]
- Hashiramoto M., Kadowaki T., Clark A. E., Muraoka A., Momomura K., Sakura H., Tobe K., Akanuma Y., Yazaki Y., Holman G. D. Site-directed mutagenesis of GLUT1 in helix 7 residue 282 results in perturbation of exofacial ligand binding. J Biol Chem. 1992 Sep 5;267(25):17502–17507. [PubMed] [Google Scholar]
- Hebert D. N., Carruthers A. Glucose transporter oligomeric structure determines transporter function. Reversible redox-dependent interconversions of tetrameric and dimeric GLUT1. J Biol Chem. 1992 Nov 25;267(33):23829–23838. [PubMed] [Google Scholar]
- Holman G. D., Rees W. D. Photolabelling of the hexose transporter at external and internal sites: fragmentation patterns and evidence for a conformational change. Biochim Biophys Acta. 1987 Mar 12;897(3):395–405. doi: 10.1016/0005-2736(87)90437-8. [DOI] [PubMed] [Google Scholar]
- Ishihara H., Asano T., Katagiri H., Lin J. L., Tsukuda K., Shibasaki Y., Yazaki Y., Oka Y. The glucose transport activity of GLUT1 is markedly decreased by substitution of a single amino acid with a different charge at residue 415. Biochem Biophys Res Commun. 1991 Apr 30;176(2):922–930. doi: 10.1016/s0006-291x(05)80274-8. [DOI] [PubMed] [Google Scholar]
- Katagiri H., Asano T., Shibasaki Y., Lin J. L., Tsukuda K., Ishihara H., Akanuma Y., Takaku F., Oka Y. Substitution of leucine for tryptophan 412 does not abolish cytochalasin B labeling but markedly decreases the intrinsic activity of GLUT1 glucose transporter. J Biol Chem. 1991 Apr 25;266(12):7769–7773. [PubMed] [Google Scholar]
- Keller K., Mueckler M. Different mammalian facilitative glucose transporters expressed in Xenopus oocytes. Biomed Biochim Acta. 1990;49(12):1201–1203. [PubMed] [Google Scholar]
- Keller K., Strube M., Mueckler M. Functional expression of the human HepG2 and rat adipocyte glucose transporters in Xenopus oocytes. Comparison of kinetic parameters. J Biol Chem. 1989 Nov 15;264(32):18884–18889. [PubMed] [Google Scholar]
- King A. P., Tai P. K., Carter-Su C. Cytochalasin B interferes with conformational changes of the human erythrocyte glucose transporter induced by internal and external sugar binding. Biochemistry. 1991 Dec 10;30(49):11546–11553. doi: 10.1021/bi00113a009. [DOI] [PubMed] [Google Scholar]
- Krieg P. A., Melton D. A. Functional messenger RNAs are produced by SP6 in vitro transcription of cloned cDNAs. Nucleic Acids Res. 1984 Sep 25;12(18):7057–7070. doi: 10.1093/nar/12.18.7057. [DOI] [PMC free article] [PubMed] [Google Scholar]
- Krupka R. M. Reaction of the glucose carrier of erythrocytes with sodium tetrathionate: evidence for inward-facing and outward-facing carrier conformations. J Membr Biol. 1985;84(1):35–43. doi: 10.1007/BF01871646. [DOI] [PubMed] [Google Scholar]
- Kunkel T. A., Roberts J. D., Zakour R. A. Rapid and efficient site-specific mutagenesis without phenotypic selection. Methods Enzymol. 1987;154:367–382. doi: 10.1016/0076-6879(87)54085-x. [DOI] [PubMed] [Google Scholar]
- May J. M., Beechem J. M. Monitoring conformational change in the human erythrocyte glucose carrier: use of a fluorescent probe attached to an exofacial carrier sulfhydryl. Biochemistry. 1993 Mar 23;32(11):2907–2915. doi: 10.1021/bi00062a022. [DOI] [PubMed] [Google Scholar]
- Mueckler M., Caruso C., Baldwin S. A., Panico M., Blench I., Morris H. R., Allard W. J., Lienhard G. E., Lodish H. F. Sequence and structure of a human glucose transporter. Science. 1985 Sep 6;229(4717):941–945. doi: 10.1126/science.3839598. [DOI] [PubMed] [Google Scholar]
- Mueckler M., Lodish H. F. The human glucose transporter can insert posttranslationally into microsomes. Cell. 1986 Feb 28;44(4):629–637. doi: 10.1016/0092-8674(86)90272-2. [DOI] [PubMed] [Google Scholar]
- Pessino A., Hebert D. N., Woon C. W., Harrison S. A., Clancy B. M., Buxton J. M., Carruthers A., Czech M. P. Evidence that functional erythrocyte-type glucose transporters are oligomers. J Biol Chem. 1991 Oct 25;266(30):20213–20217. [PubMed] [Google Scholar]
- Rampal A. L., Jung C. Y. Substrate-induced conformational change of human erythrocyte glucose transporter: inactivation by alkylating reagents. Biochim Biophys Acta. 1987 Jan 26;896(2):287–294. doi: 10.1016/0005-2736(87)90189-1. [DOI] [PubMed] [Google Scholar]
- Silverman M. Structure and function of hexose transporters. Annu Rev Biochem. 1991;60:757–794. doi: 10.1146/annurev.bi.60.070191.003545. [DOI] [PubMed] [Google Scholar]
- Walmsley A. R. The dynamics of the glucose transporter. Trends Biochem Sci. 1988 Jun;13(6):226–231. doi: 10.1016/0968-0004(88)90089-8. [DOI] [PubMed] [Google Scholar]
- Wellner M., Monden I., Keller K. The differential role of Cys-421 and Cys-429 of the Glut1 glucose transporter in transport inhibition by p-chloromercuribenzenesulfonic acid (pCMBS) or cytochalasin B (CB). FEBS Lett. 1992 Sep 14;309(3):293–296. doi: 10.1016/0014-5793(92)80792-f. [DOI] [PubMed] [Google Scholar]