Abstract
The air-filled microspheres of the ultrasound-contrast agent Albunex are unique in that the walls consist of human serum albumin molecules which have been made insoluble by sonication of the albumin solution. The microspheres were isolated by flotation, and the washed microspheres were labelled with 125I. The labelled material was cleared from the circulation mainly as particles, not as soluble albumin molecules. In rats, 80% of intravenously injected microspheres were cleared from the blood within 2 min. Nearly 60% of the dose was recovered in the liver, only 5% in the lungs, 9% in the spleen, and negligible quantities in kidneys, heart and brain. Of the radioactivity in the liver, more than 90% was taken up by Kupffer cells (liver macrophages). The protein in the liver was degraded apparently with first-order kinetics (half-life 40 min). In pigs, over 90% of the intravenously injected dose was recovered in the lungs. The vastly increased recovery in pig lungs, compared with that in rats, is probably due to the pulmonary intravascular macrophages of the pig; macrophages are not normally found in this location in rats (or humans). In a separate series of experiments in rats, the biodistribution of shell material from the microspheres was examined. The microspheres were made to collapse by applying external pressure on the suspension, leaving sedimentable protein material consisting of layers of insoluble albumin from the 'shells' surrounding the air bubble. The 'shells' and the microspheres were cleared from the circulation and taken up by the liver with the same kinetics. In the lungs, a higher proportion (15%) of shells than of microspheres was recovered.
Full text
PDF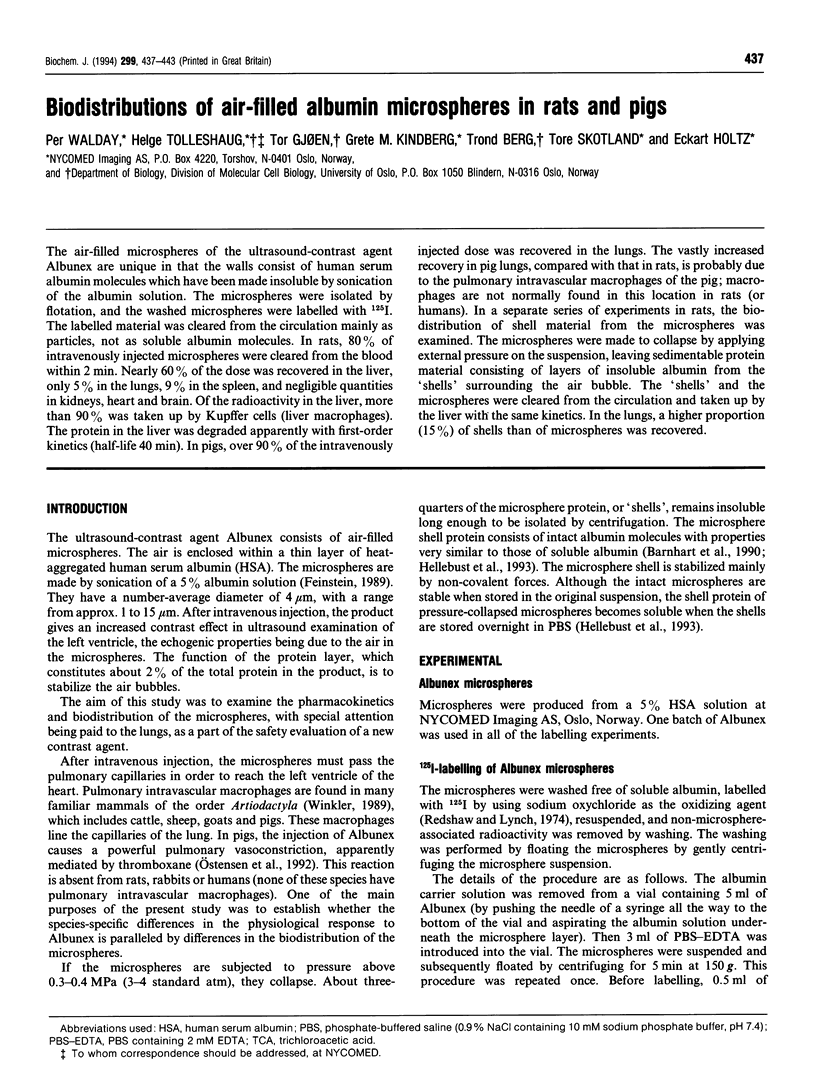
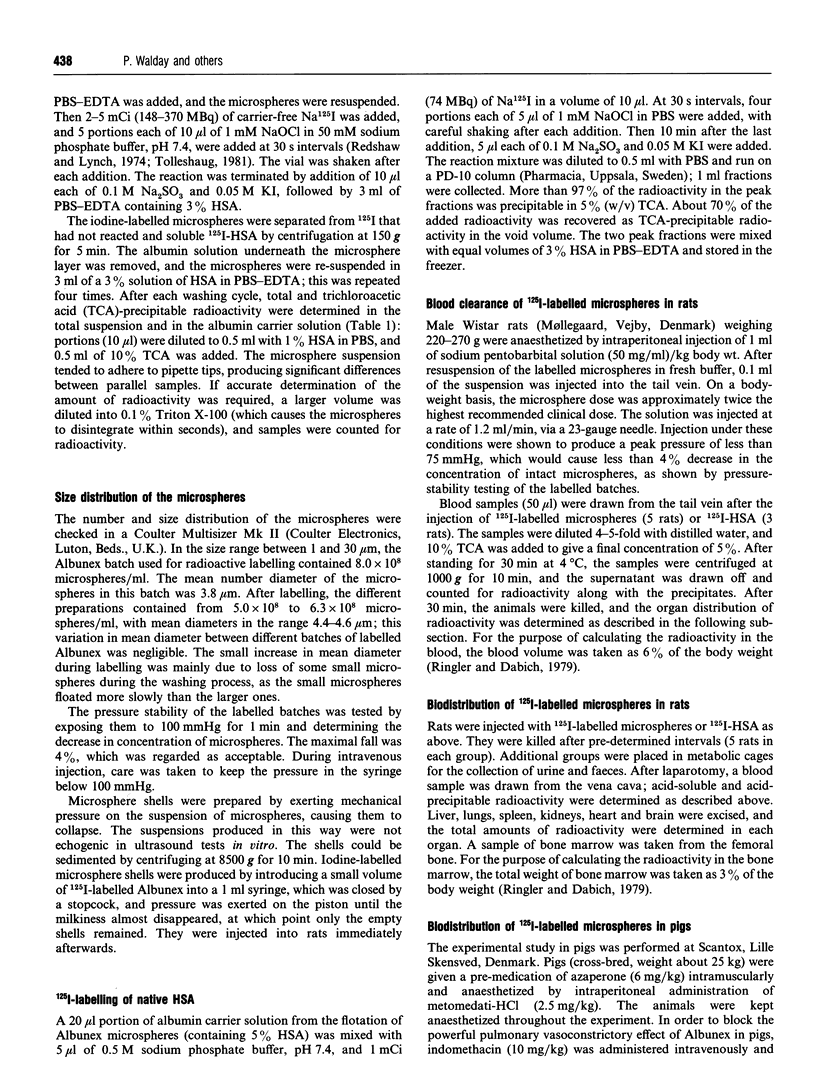
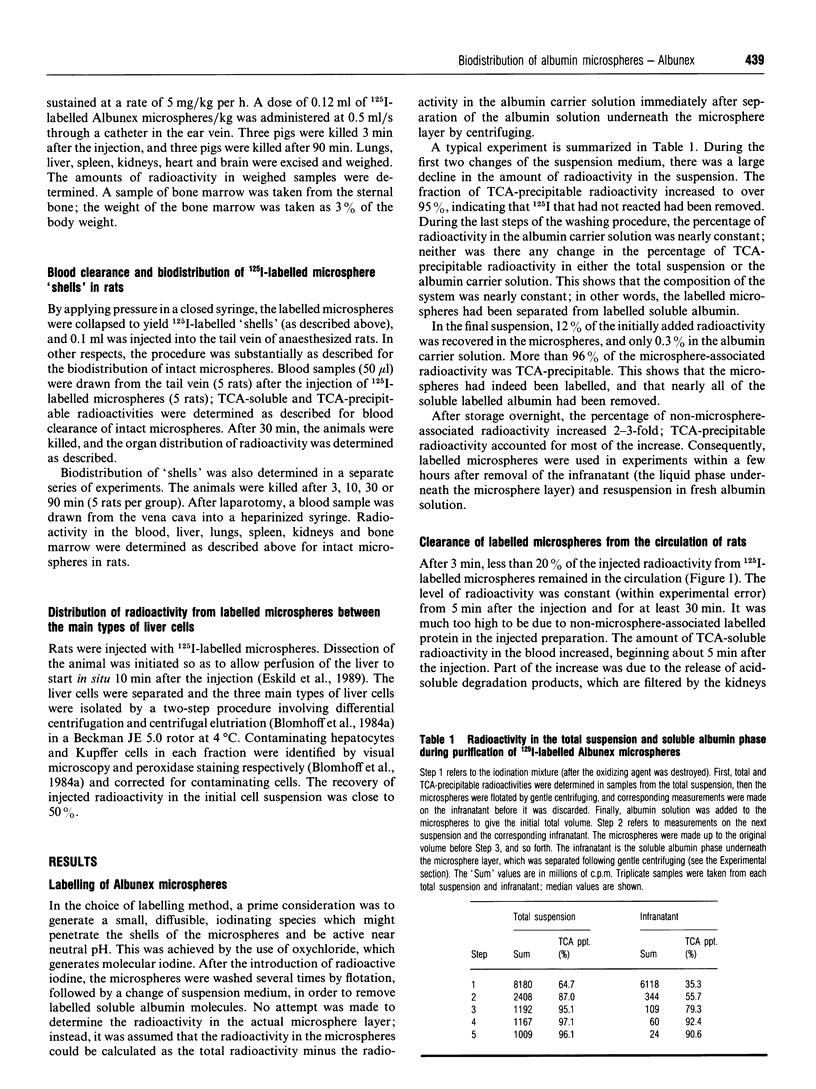
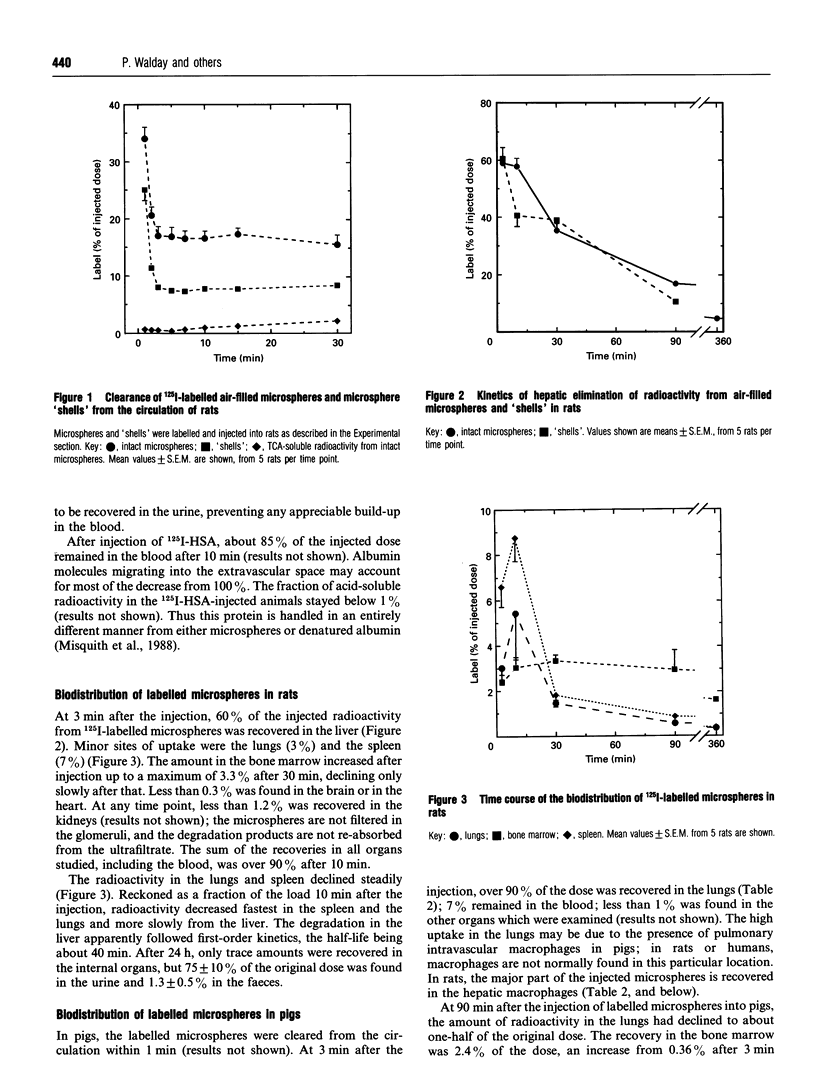
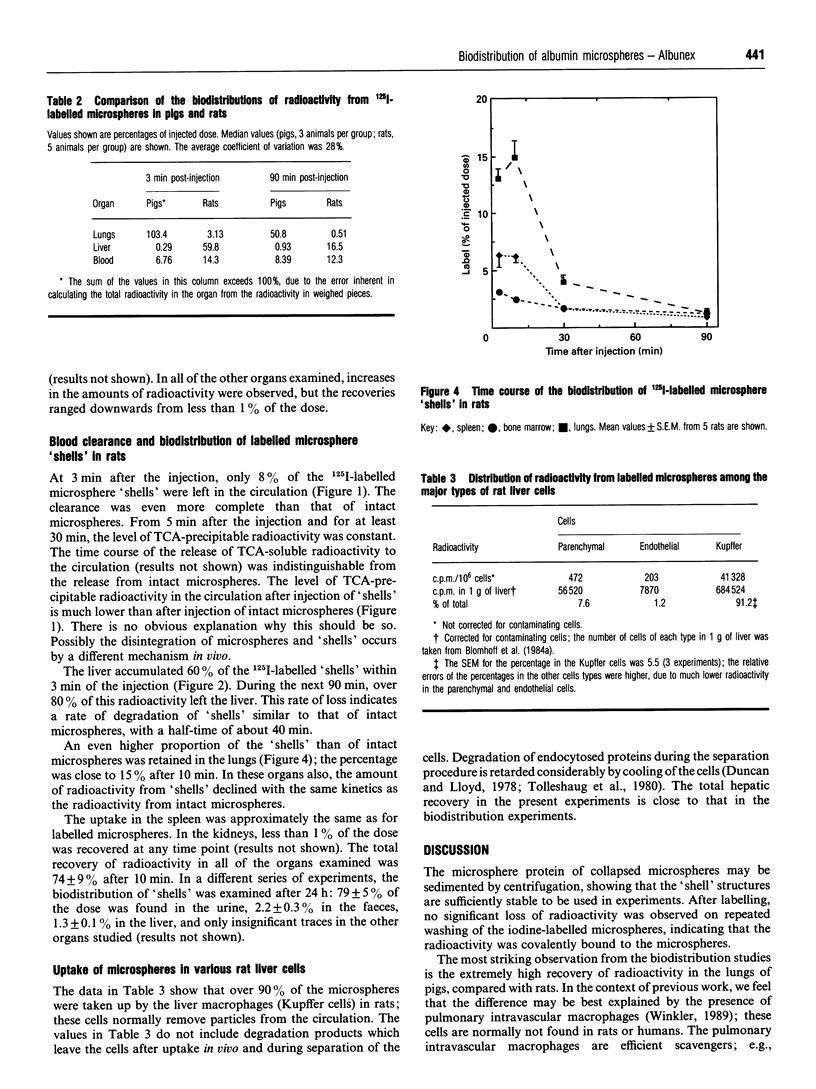
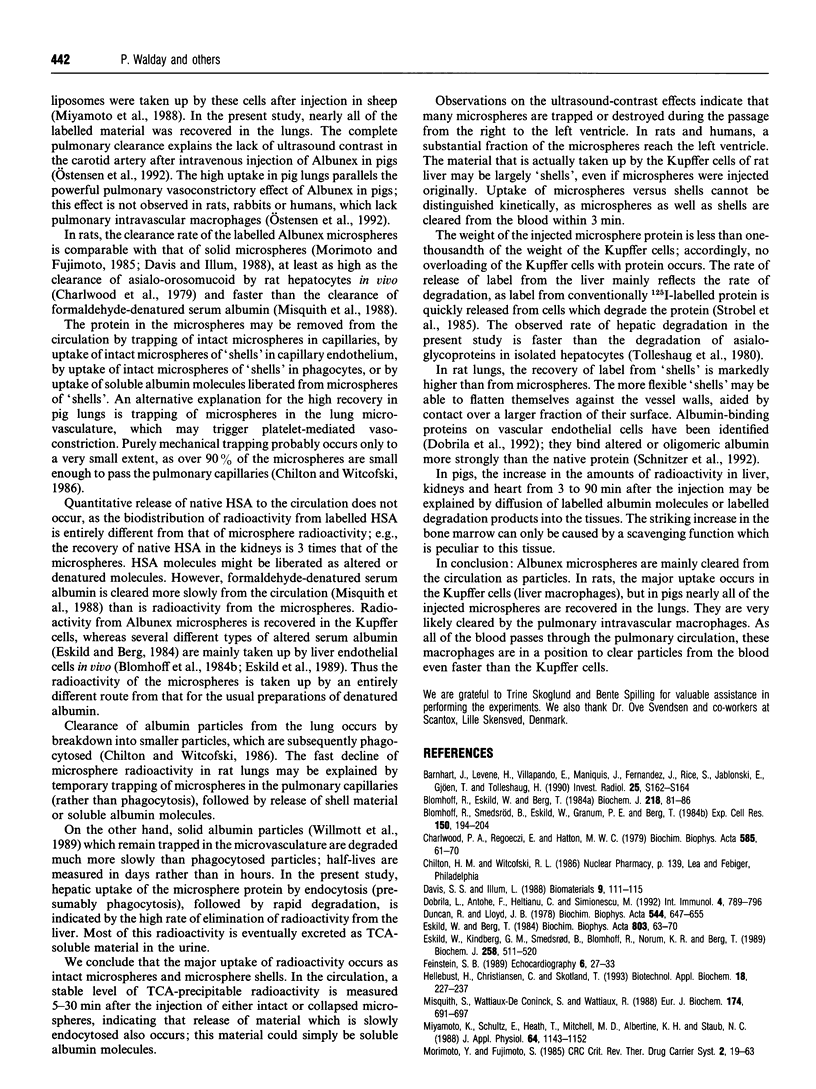
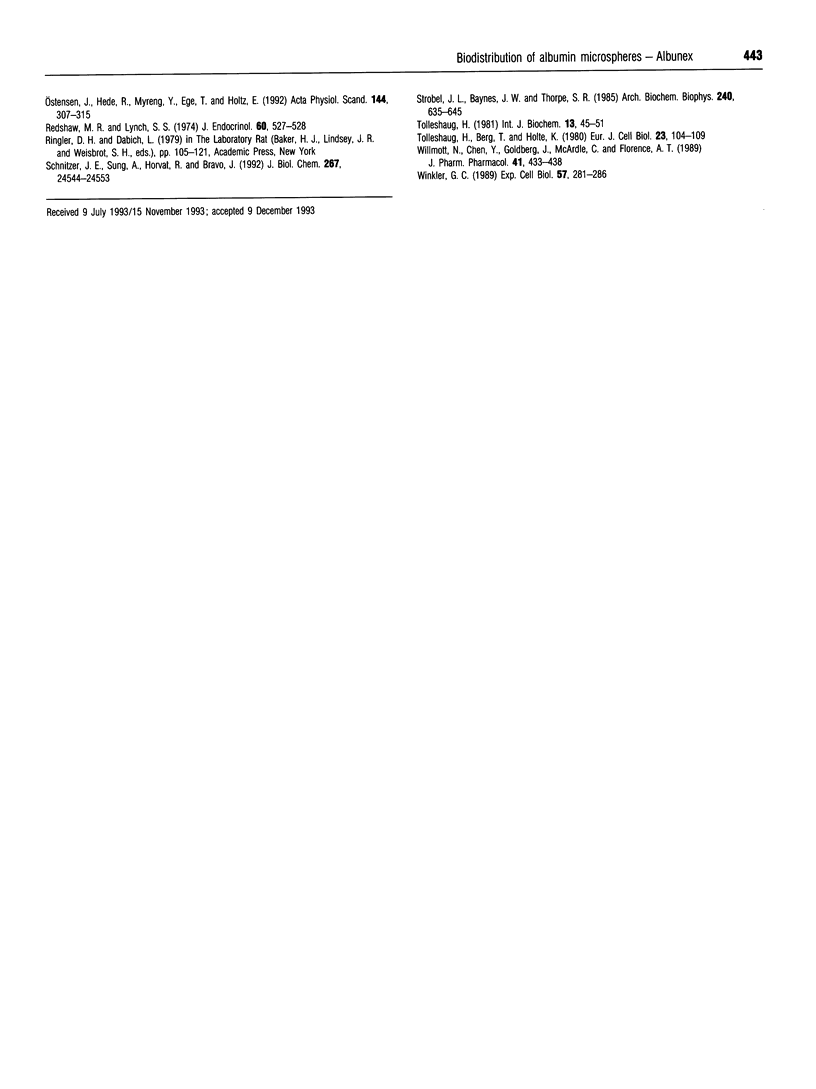
Selected References
These references are in PubMed. This may not be the complete list of references from this article.
- Barnhart J., Levene H., Villapando E., Maniquis J., Fernandez J., Rice S., Jablonski E., Gjøen T., Tolleshaug H. Characteristics of Albunex: air-filled albumin microspheres for echocardiography contrast enhancement. Invest Radiol. 1990 Sep;25 (Suppl 1):S162–S164. doi: 10.1097/00004424-199009001-00070. [DOI] [PubMed] [Google Scholar]
- Blomhoff R., Eskild W., Berg T. Endocytosis of formaldehyde-treated serum albumin via scavenger pathway in liver endothelial cells. Biochem J. 1984 Feb 15;218(1):81–86. doi: 10.1042/bj2180081. [DOI] [PMC free article] [PubMed] [Google Scholar]
- Blomhoff R., Smedsrød B., Eskild W., Granum P. E., Berg T. Preparation of isolated liver endothelial cells and Kupffer cells in high yield by means of an enterotoxin. Exp Cell Res. 1984 Jan;150(1):194–204. doi: 10.1016/0014-4827(84)90714-6. [DOI] [PubMed] [Google Scholar]
- Charlwood P. A., Regoeczi E., Hatton M. W. Hepatic uptake and degradation of trace doses of asialofetuin and asialoorosomucoid in the intact rat. Biochim Biophys Acta. 1979 Jun 1;585(1):61–70. doi: 10.1016/0304-4165(79)90325-8. [DOI] [PubMed] [Google Scholar]
- Davis S. S., Illum L. Polymeric microspheres as drug carriers. Biomaterials. 1988 Jan;9(1):111–115. doi: 10.1016/0142-9612(88)90081-6. [DOI] [PubMed] [Google Scholar]
- Dobrila L., Antohe F., Heltianu C., Simionescu M. Albumin binding proteins of endothelial cells identified by anti-idiotypic antibodies. Int Immunol. 1992 Jul;4(7):789–796. doi: 10.1093/intimm/4.7.789. [DOI] [PubMed] [Google Scholar]
- Duncan R., Lloyd J. B. Pinocytosis in the rat visceral yolk sac. Effects of temperature, metabolic inhibitors and some other modifiers. Biochim Biophys Acta. 1978 Dec 18;544(3):647–655. doi: 10.1016/0304-4165(78)90339-2. [DOI] [PubMed] [Google Scholar]
- Eskild W., Berg T. Endocytosis of formaldehyde-denatured serum albumin by nonparenchymal liver cells in vitro. Biochim Biophys Acta. 1984 Feb 17;803(1-2):63–70. doi: 10.1016/0167-4889(84)90055-7. [DOI] [PubMed] [Google Scholar]
- Eskild W., Kindberg G. M., Smedsrod B., Blomhoff R., Norum K. R., Berg T. Intracellular transport of formaldehyde-treated serum albumin in liver endothelial cells after uptake via scavenger receptors. Biochem J. 1989 Mar 1;258(2):511–520. doi: 10.1042/bj2580511. [DOI] [PMC free article] [PubMed] [Google Scholar]
- Hellebust H., Christiansen C., Skotland T. Biochemical characterization of air-filled albumin microspheres. Biotechnol Appl Biochem. 1993 Dec;18(Pt 3):227–237. [PubMed] [Google Scholar]
- Misquith S., Wattiaux-De Coninck S., Wattiaux R. Uptake and intracellular transport in rat liver of formaldehyde-treated bovine serum albumin labelled with 125I-tyramine-cellobiose. Eur J Biochem. 1988 Jul 1;174(4):691–697. doi: 10.1111/j.1432-1033.1988.tb14153.x. [DOI] [PubMed] [Google Scholar]
- Miyamoto K., Schultz E., Heath T., Mitchell M. D., Albertine K. H., Staub N. C. Pulmonary intravascular macrophages and hemodynamic effects of liposomes in sheep. J Appl Physiol (1985) 1988 Mar;64(3):1143–1152. doi: 10.1152/jappl.1988.64.3.1143. [DOI] [PubMed] [Google Scholar]
- Morimoto Y., Fujimoto S. Albumin microspheres as drug carriers. Crit Rev Ther Drug Carrier Syst. 1985;2(1):19–63. [PubMed] [Google Scholar]
- Ostensen J., Hede R., Myreng Y., Ege T., Holtz E. Intravenous injection of Albunex microspheres causes thromboxane mediated pulmonary hypertension in pigs, but not in monkeys or rabbits. Acta Physiol Scand. 1992 Mar;144(3):307–315. doi: 10.1111/j.1748-1716.1992.tb09299.x. [DOI] [PubMed] [Google Scholar]
- Redshaw M. R., Lynch S. S. An improved method for the preparation of iodinated antigens for radioimmunoassay. J Endocrinol. 1974 Mar;60(3):527–528. doi: 10.1677/joe.0.0600527. [DOI] [PubMed] [Google Scholar]
- Schnitzer J. E., Sung A., Horvat R., Bravo J. Preferential interaction of albumin-binding proteins, gp30 and gp18, with conformationally modified albumins. Presence in many cells and tissues with a possible role in catabolism. J Biol Chem. 1992 Dec 5;267(34):24544–24553. [PubMed] [Google Scholar]
- Strobel J. L., Baynes J. W., Thorpe S. R. 125I-glycoconjugate labels for identifying sites of protein catabolism in vivo: effect of structure and chemistry of coupling to protein on label entrapment in cells after protein degradation. Arch Biochem Biophys. 1985 Aug 1;240(2):635–645. doi: 10.1016/0003-9861(85)90071-2. [DOI] [PubMed] [Google Scholar]
- Tolleshaug H., Berg T., Holte K. Kinetics of internalization and degradation of asialo-glycoproteins in isolated rat hepatocytes. Eur J Cell Biol. 1980 Dec;23(1):104–109. [PubMed] [Google Scholar]
- Tolleshaug H. Binding and internalization of asialo-glycoproteins by isolated rat hepatocytes. Int J Biochem. 1981;13(1):45–51. doi: 10.1016/0020-711x(81)90135-x. [DOI] [PubMed] [Google Scholar]
- Willmott N., Chen Y., Goldberg J., Mcardle C., Florence A. T. Biodegradation rate of embolized protein microspheres in lung, liver and kidney of rats. J Pharm Pharmacol. 1989 Jul;41(7):433–438. doi: 10.1111/j.2042-7158.1989.tb06496.x. [DOI] [PubMed] [Google Scholar]
- Winkler G. C. Review of the significance of pulmonary intravascular macrophages with respect to animal species and age. Exp Cell Biol. 1989;57(6):281–286. doi: 10.1159/000163539. [DOI] [PubMed] [Google Scholar]