Abstract
The interaction of clavulanic acid with beta-lactamase from Staphylococcus aureus was investigated, particularly with a view to determining whether conformational effects are involved. The inactivation at neutral pH is essentially stoichiometric, leading to an inactive species with an enamine chromophore. Two forms of the enamine were observed, the first-formed having a positive ellipticity with a maximum near 290 nm. This species slowly converted into the stable form of the inactivated enzyme that had a negative ellipticity with a minimum at 275 nm. This change in sign of the ellipticity of the enamine is consistent with the previously proposed cis-trans isomerization of the enamine [Cartwright & Coulson (1979) Nature (London) 278, 360-361). Both the far-u.v.c.d. and the intrinsic viscosity of the inactivated enzyme indicated that negligible change in conformation of the enzyme accompanied inactivation. The rates of inactivation and enamine formation were compared at low temperatures, where the initial rates were slow enough to be monitored. The rate of loss of 95% of the catalytic activity was almost 100-fold faster than the rate of formation of the first-formed enamine species. The remaining 5% activity was lost with a rate comparable with that for formation of the initial enamine. The simplest explanation of these results is that a relatively stable acyl-enzyme intermediate builds up initially and more slowly partitions between turnover (hydrolysis) and enamine formation. The initially formed enamine is in the cis conformation but slowly isomerizes to the more stable trans form.
Full text
PDF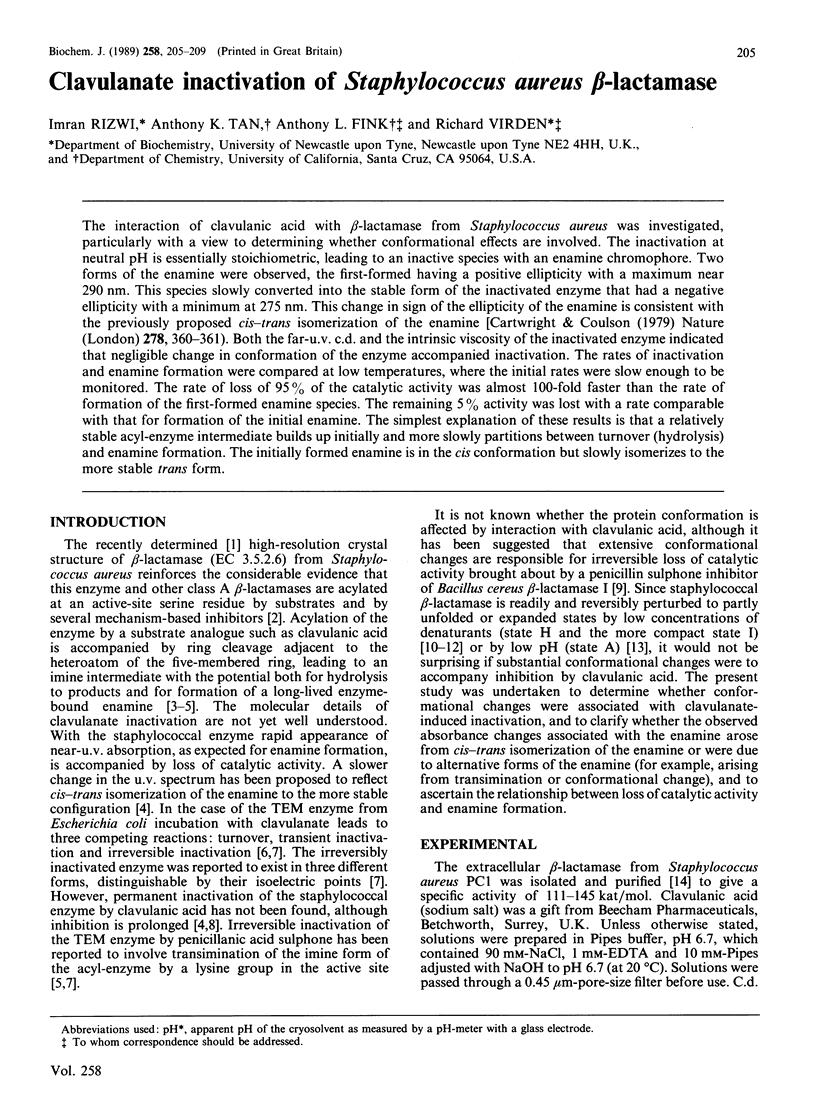
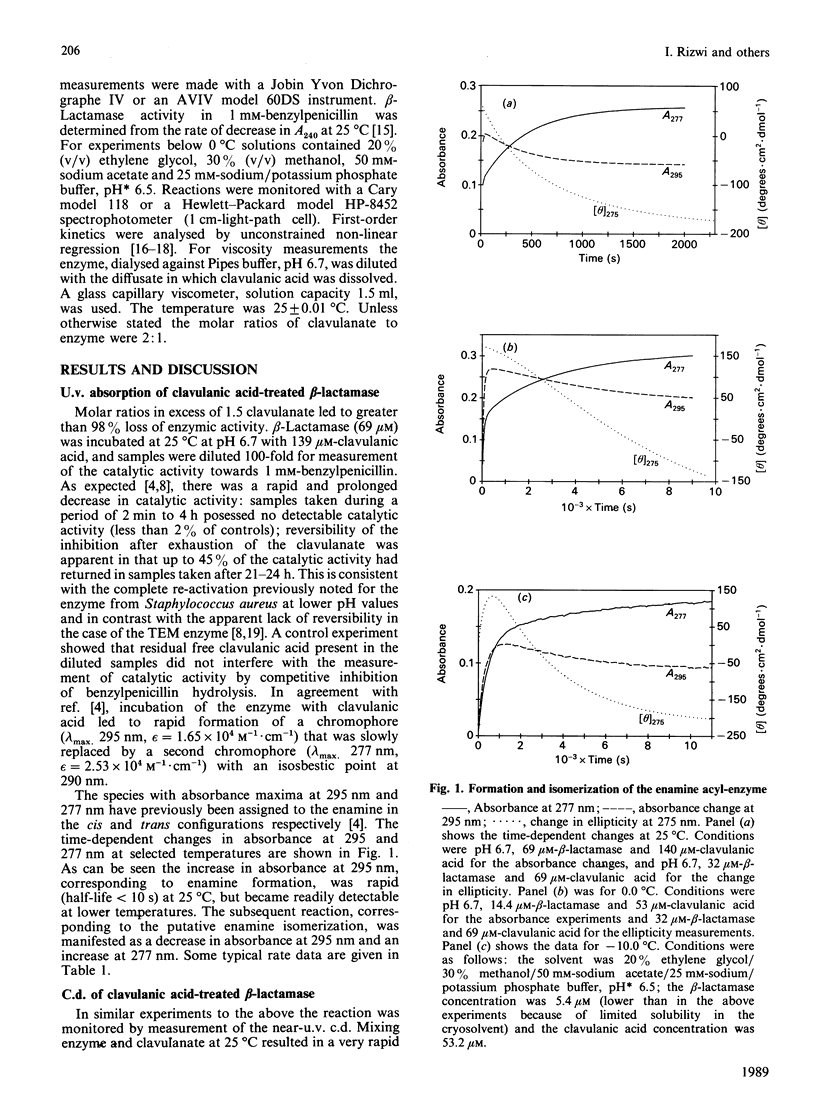
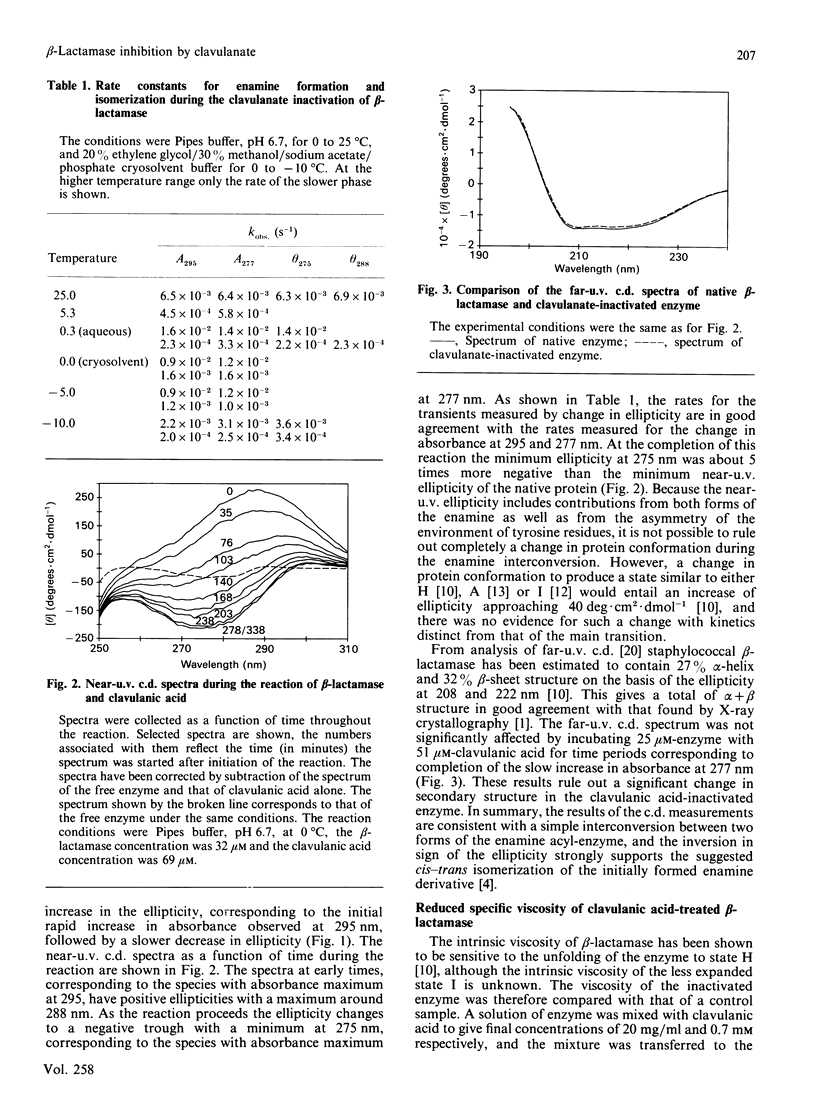
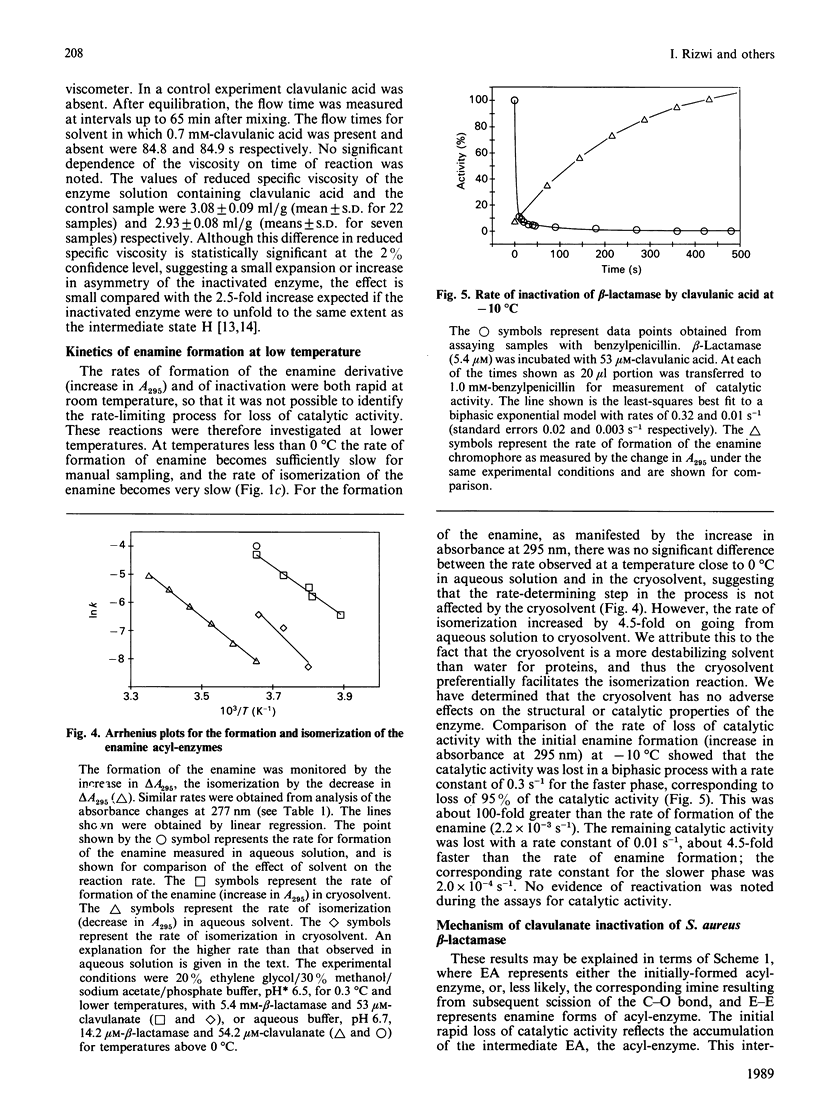
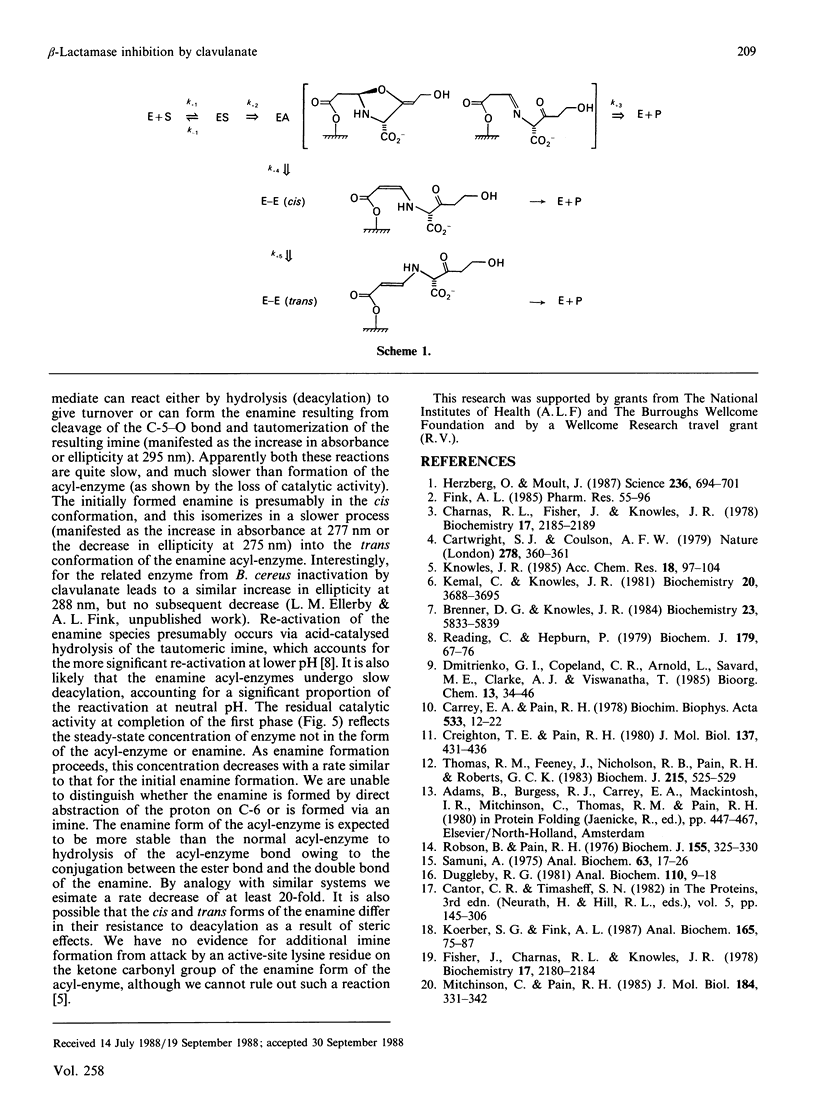
Selected References
These references are in PubMed. This may not be the complete list of references from this article.
- Brenner D. G., Knowles J. R. Penicillanic acid sulfone: nature of irreversible inactivation of RTEM beta-lactamase from Escherichia coli. Biochemistry. 1984 Nov 20;23(24):5833–5839. doi: 10.1021/bi00319a024. [DOI] [PubMed] [Google Scholar]
- Carrey E. A., Pain R. H. Conformation of a stable intermediate on the folding pathway of Staphylococcus aureus penicillinase. Biochim Biophys Acta. 1978 Mar 28;533(1):12–22. doi: 10.1016/0005-2795(78)90542-1. [DOI] [PubMed] [Google Scholar]
- Cartwright S. J., Coulson A. F. A semi-synthetic penicillinase inactivator. Nature. 1979 Mar 22;278(5702):360–361. doi: 10.1038/278360a0. [DOI] [PubMed] [Google Scholar]
- Charnas R. L., Fisher J., Knowles J. R. Chemical studies on the inactivation of Escherichia coli RTEM beta-lactamase by clavulanic acid. Biochemistry. 1978 May 30;17(11):2185–2189. doi: 10.1021/bi00604a025. [DOI] [PubMed] [Google Scholar]
- Creighton T. E., Pain R. H. Unfolding and refolding of Staphylococcus aureus penicillinase by urea-gradient electrophoresis. J Mol Biol. 1980 Mar 15;137(4):431–436. doi: 10.1016/0022-2836(80)90167-9. [DOI] [PubMed] [Google Scholar]
- Duggleby R. G. A nonlinear regression program for small computers. Anal Biochem. 1981 Jan 1;110(1):9–18. doi: 10.1016/0003-2697(81)90104-4. [DOI] [PubMed] [Google Scholar]
- Fisher J., Charnas R. L., Knowles J. R. Kinetic studies on the inactivation of Escherichia coli RTEM beta-lactamase by clavulanic acid. Biochemistry. 1978 May 30;17(11):2180–2184. doi: 10.1021/bi00604a024. [DOI] [PubMed] [Google Scholar]
- Herzberg O., Moult J. Bacterial resistance to beta-lactam antibiotics: crystal structure of beta-lactamase from Staphylococcus aureus PC1 at 2.5 A resolution. Science. 1987 May 8;236(4802):694–701. doi: 10.1126/science.3107125. [DOI] [PubMed] [Google Scholar]
- Kemal C., Knowles J. R. Penicillanic acid sulfone: interaction with RTEM beta-lactamase from Escherichia coli at different pH values. Biochemistry. 1981 Jun 23;20(13):3688–3695. doi: 10.1021/bi00516a004. [DOI] [PubMed] [Google Scholar]
- Koerber S. C., Fink A. L. The analysis of enzyme progress curves by numerical differentiation, including competitive product inhibition and enzyme reactivation. Anal Biochem. 1987 Aug 15;165(1):75–87. doi: 10.1016/0003-2697(87)90203-x. [DOI] [PubMed] [Google Scholar]
- Mitchinson C., Pain R. H. Effects of sulphate and urea on the stability and reversible unfolding of beta-lactamase from Staphylococcus aureus. Implications for the folding pathway of beta-lactamase. J Mol Biol. 1985 Jul 20;184(2):331–342. doi: 10.1016/0022-2836(85)90384-5. [DOI] [PubMed] [Google Scholar]
- Reading C., Hepburn P. The inhibition of staphylococcal beta-lactamase by clavulanic acid. Biochem J. 1979 Apr 1;179(1):67–76. doi: 10.1042/bj1790067. [DOI] [PMC free article] [PubMed] [Google Scholar]
- Robson B., Pain R. H. The mechanism of folding of globular proteins. Suitability of a penicillinase from Staphylococcus Aureus as a model for refolding studies. Biochem J. 1976 May 1;155(2):325–330. doi: 10.1042/bj1550325. [DOI] [PMC free article] [PubMed] [Google Scholar]
- Samuni A. A direct spectrophotometric assay and determination of Michaelis constants for the beta-lactamase reaction. Anal Biochem. 1975 Jan;63(1):17–26. doi: 10.1016/0003-2697(75)90185-2. [DOI] [PubMed] [Google Scholar]
- Thomas R. M., Feeney J., Nicholson R. B., Pain R. H., Roberts G. C. Identification by n.m.r. spectroscopy of a stable intermediate structure in the unfolding of staphylococcal beta-lactamase. Biochem J. 1983 Dec 1;215(3):525–529. doi: 10.1042/bj2150525. [DOI] [PMC free article] [PubMed] [Google Scholar]