Abstract
1. Intracellular recordings were made from rat locus coeruleus (LC) neurons in completely submerged brain slices. Trains of action potentials in LC neurons were followed by a prolonged post-stimulus hyperpolarization (PSH). If trains were elicited with depolarizing current pulses of sufficient intensity, PSH was composed of a fast, early component (PSHE) and a slow, late component (PSHL). PSH which followed trains elicited with lower intensity depolarizing current pulses consisted only of PSHL. 2. Both PSHE and PSHL were augmented by increasing the number of action potentials in the train and both were associated with an increase in membrane conductance. The reversal potential for PSHE was -108 mV and for PSHL it was -114 mV. 3. When a hybrid voltage clamp protocol was used, the current underlying PSH (IPSH) was observed to consist of an early, rapidly decaying component, IE, followed by a late, slower decaying component, IL. The time course of decay of IPSH was biexponential with the time constant of decay of IL more than one order of magnitude larger than the time constant of decay of IE. An increase in the concentration of external K+ shifted the reversal potentials for IE and IL in the depolarizing direction; the mean value of shift per tenfold increase in external K+ concentration was 57.1 mV for IE and 57.6 mV for IL. 4. Both PSHE and PSHL were inhibited by lowering the external Ca2+ concentration or by application of the Ca2+ channel blockers Cd2+ (200-500 microM) or nifedipine (100 microM). Intracellular injection of EGTA abolished both components of PSH. Increasing the external Ca2+ concentration augmented both PSH components. 5. Superfusion of dantrolene (25 microM) or ryanodine (20 microM) decreased the amplitude and duration of PSHL with much less effect on PSHE. 6. d-Tubocurarine (20-200 microM) selectively blocked PSHE with no effect on PSHL; this effect is the same as that of apamin which we have previously described. Superfusion with charybdotoxin (40 nM) or TEA (400 microM-1 mM) did not reduce PSHE or PSHL. 7. Inhibition of IA by 4-aminopyridine or 2,4-diaminopyridine also did not reduce either component of PSH. In fact, these agents slightly augmented both components of PSH; this effect was probably secondary to the prolongation of action potential duration. Superfusion of TEA in concentrations of 2-10 mM increased the size and duration of PSHL and increased the duration but decreased the size of PSHE.(ABSTRACT TRUNCATED AT 400 WORDS)
Full text
PDF
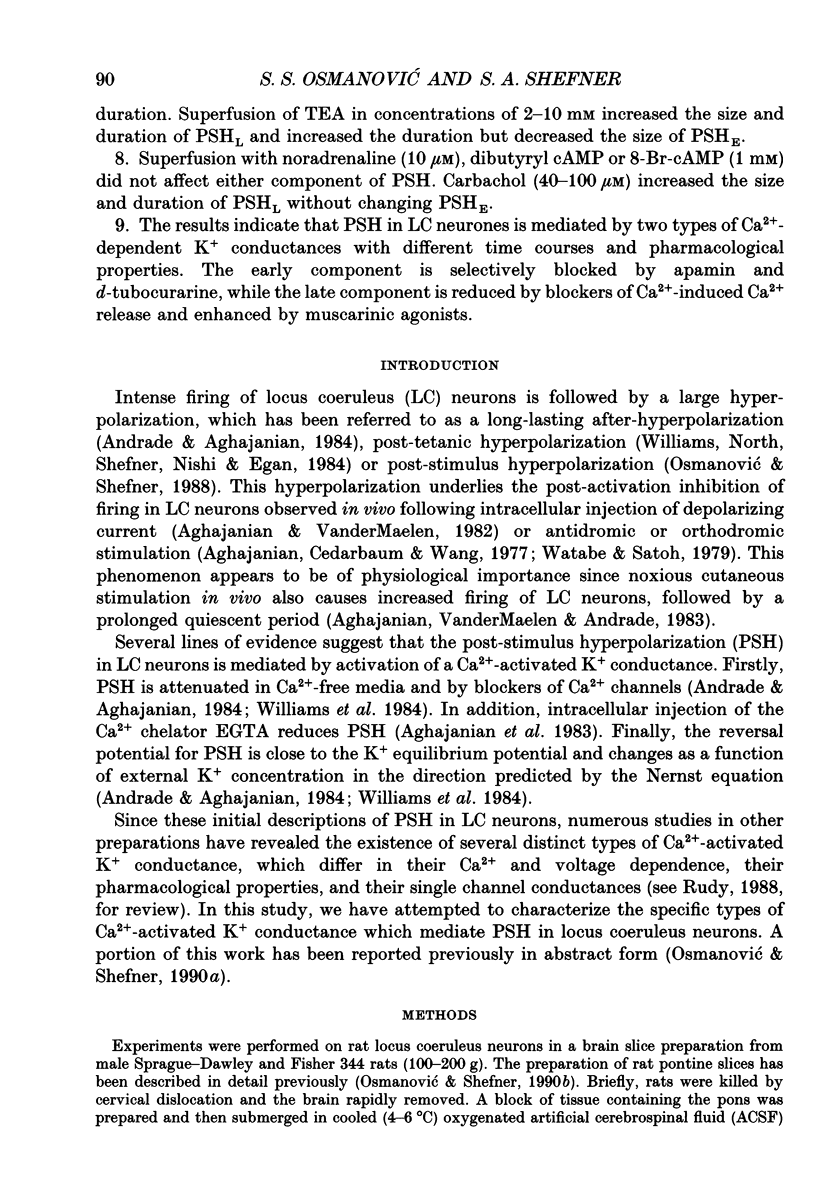
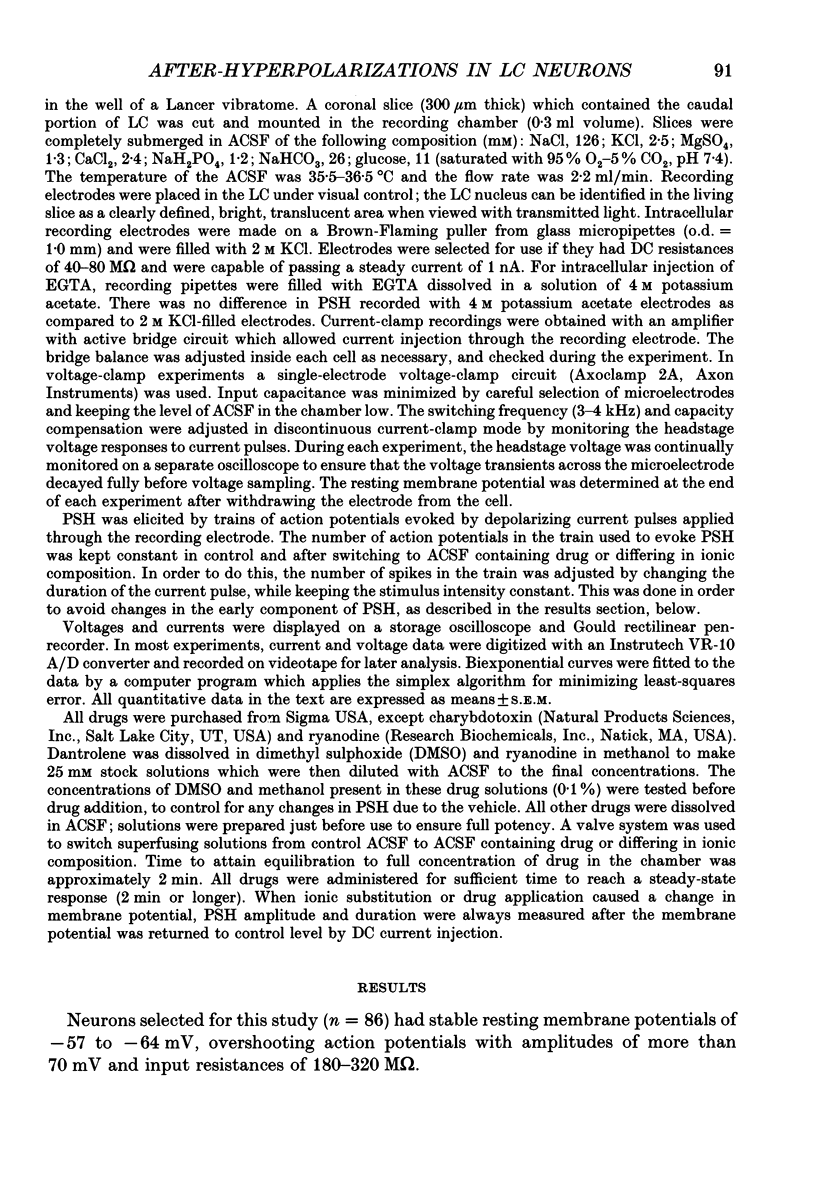
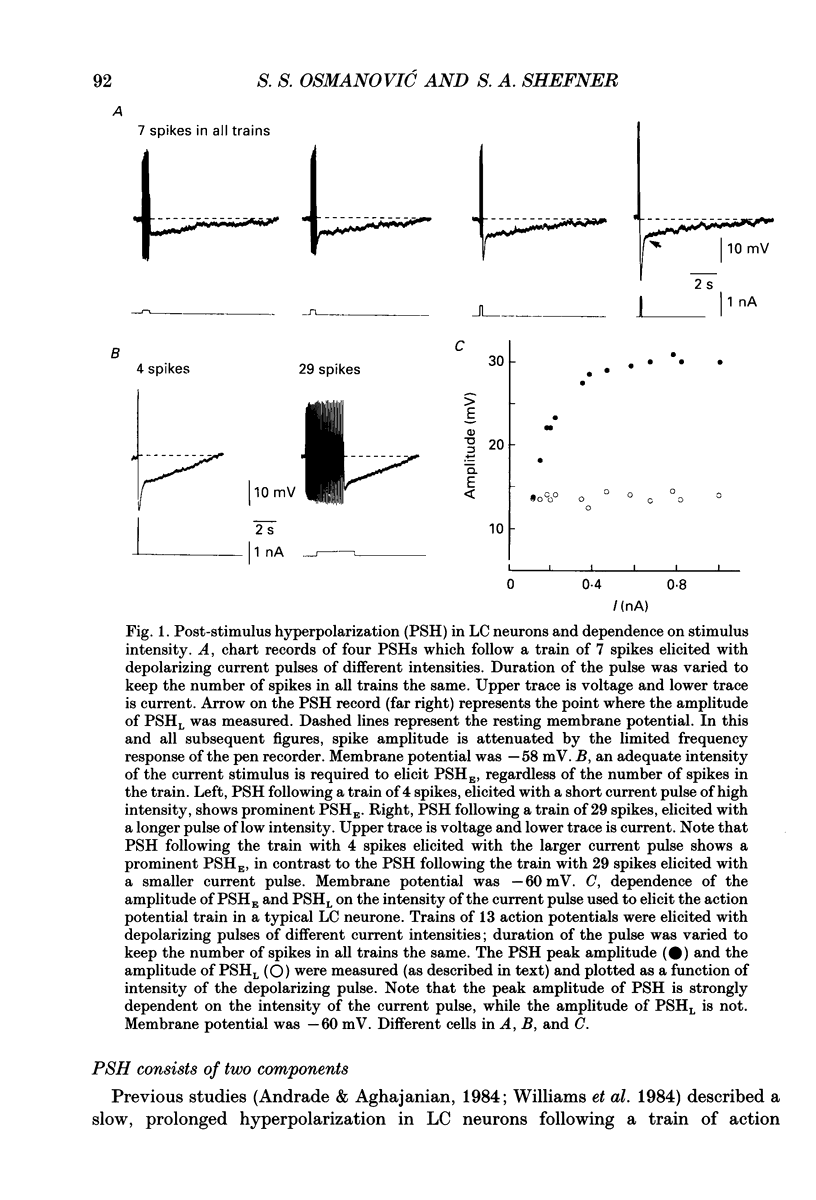
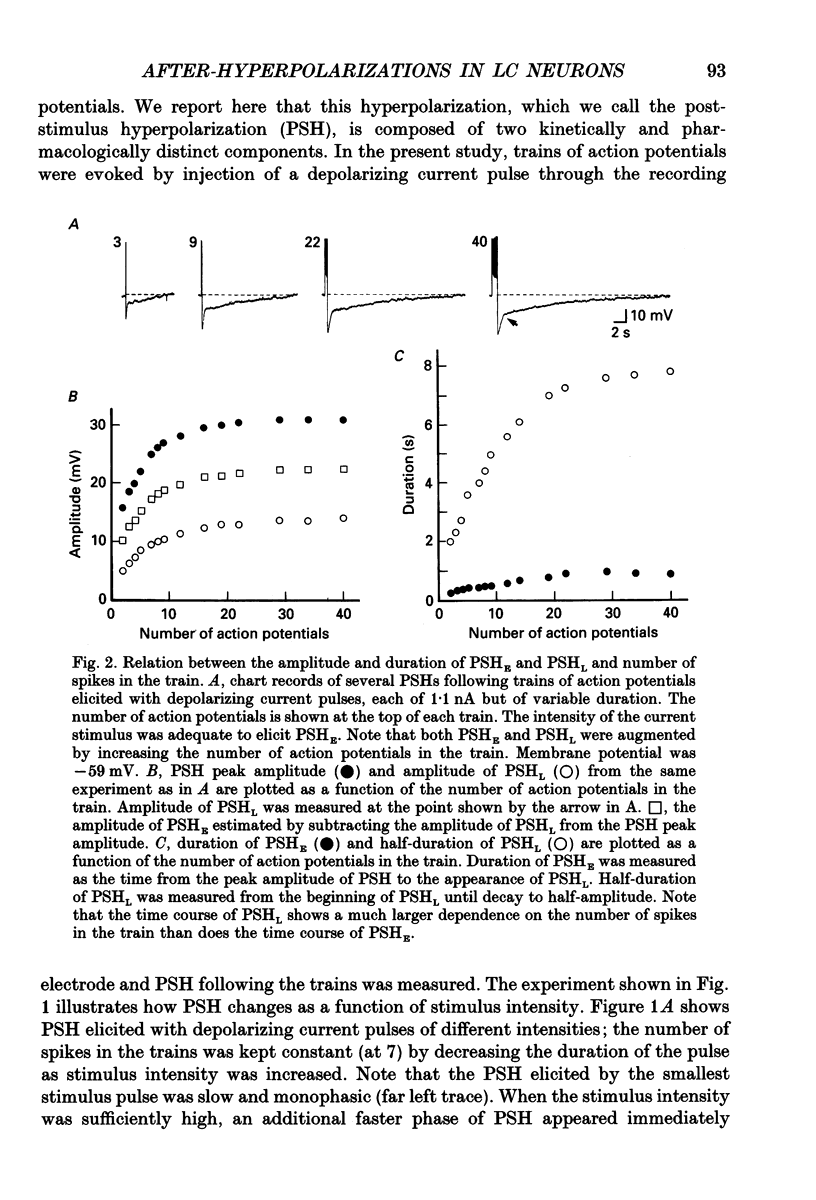
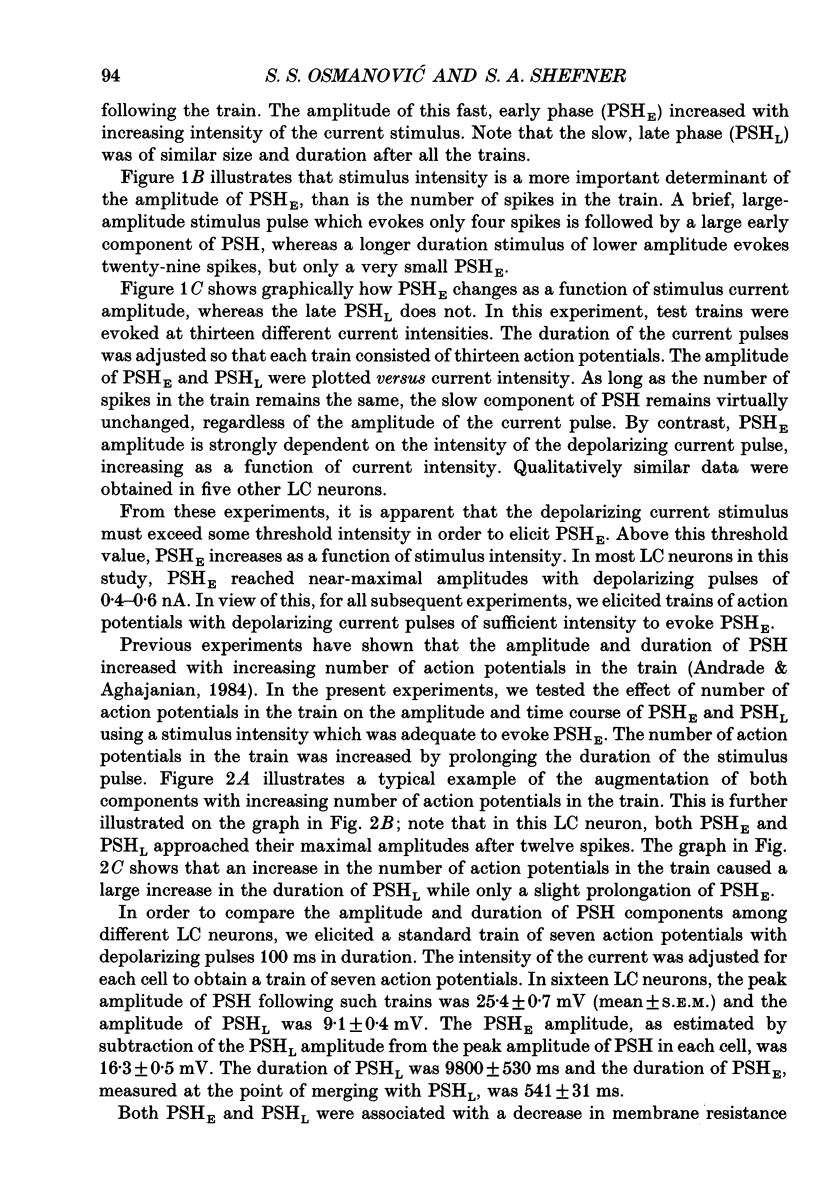
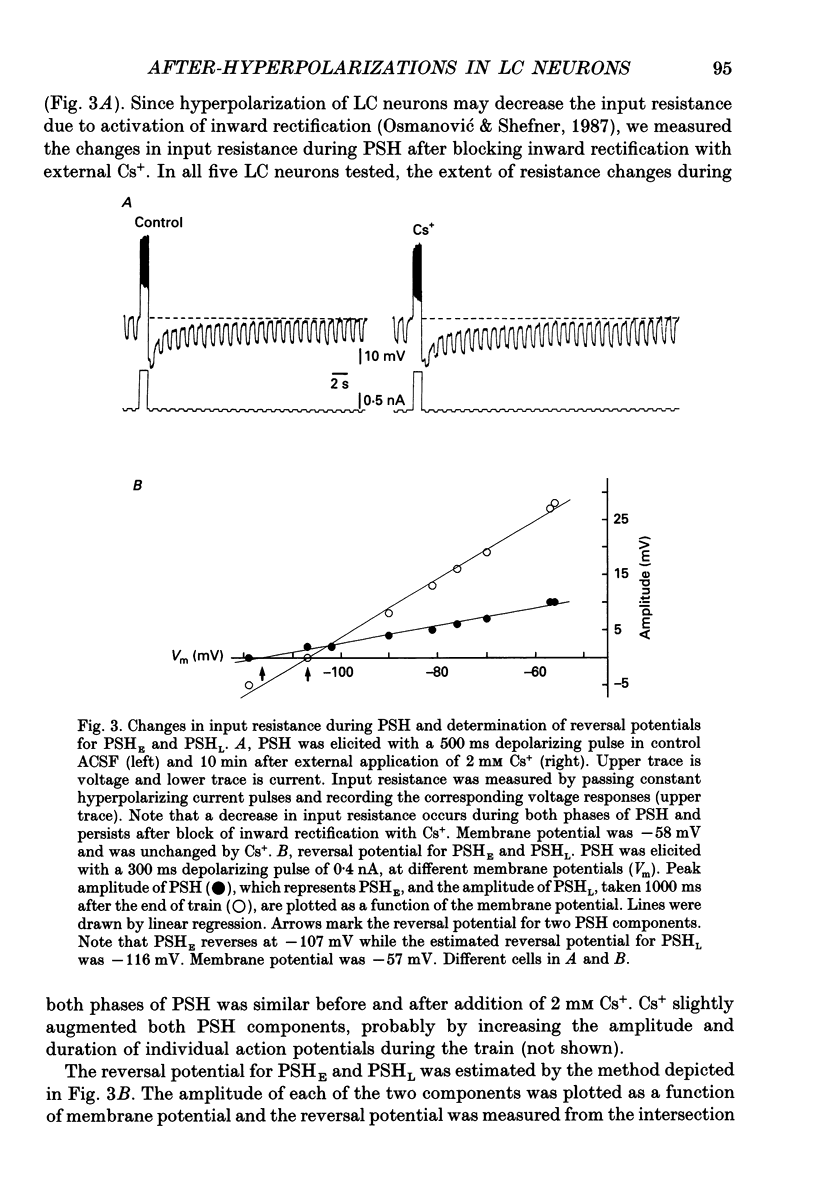
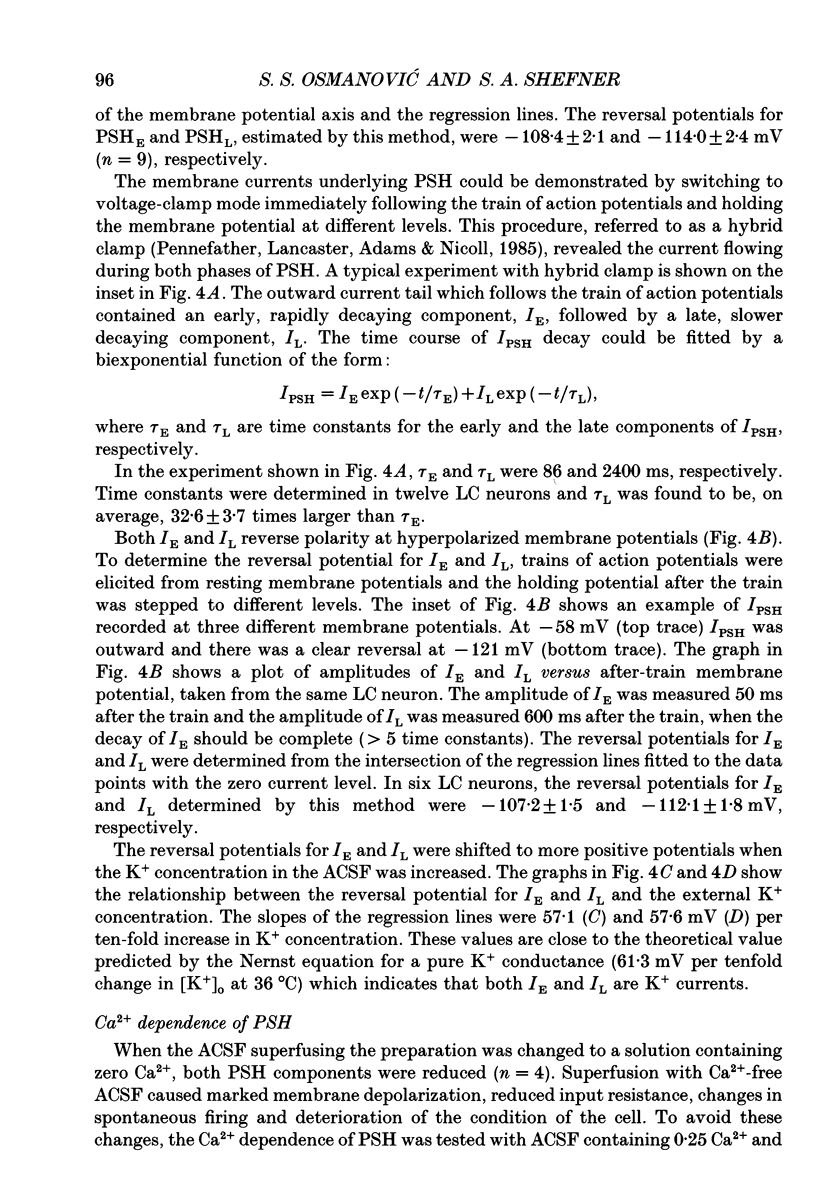
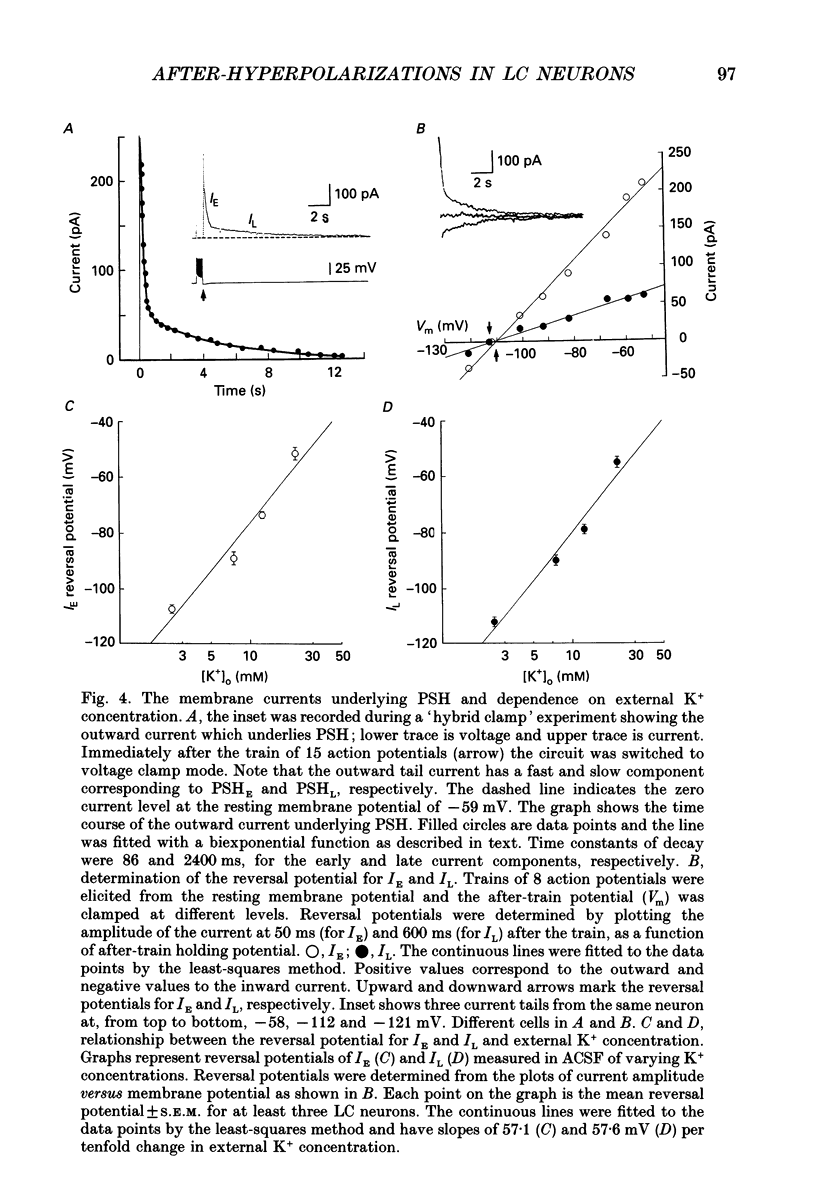
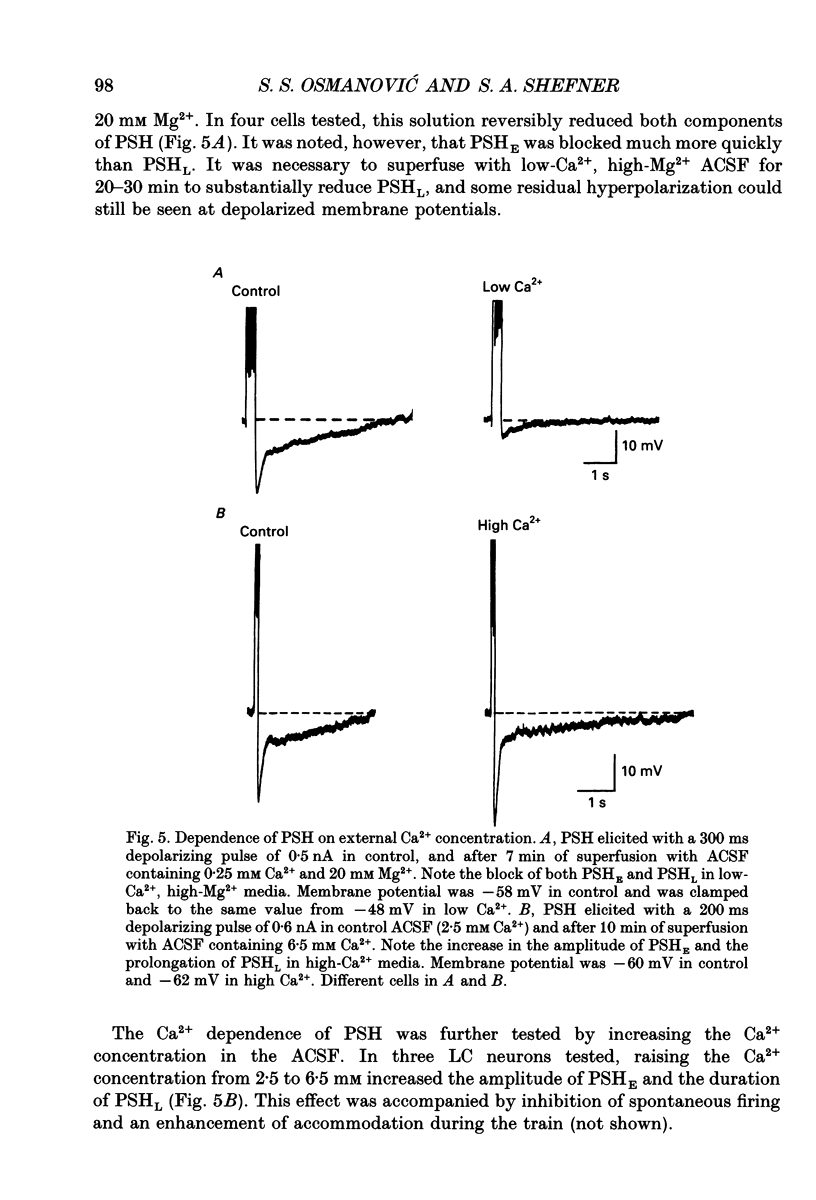
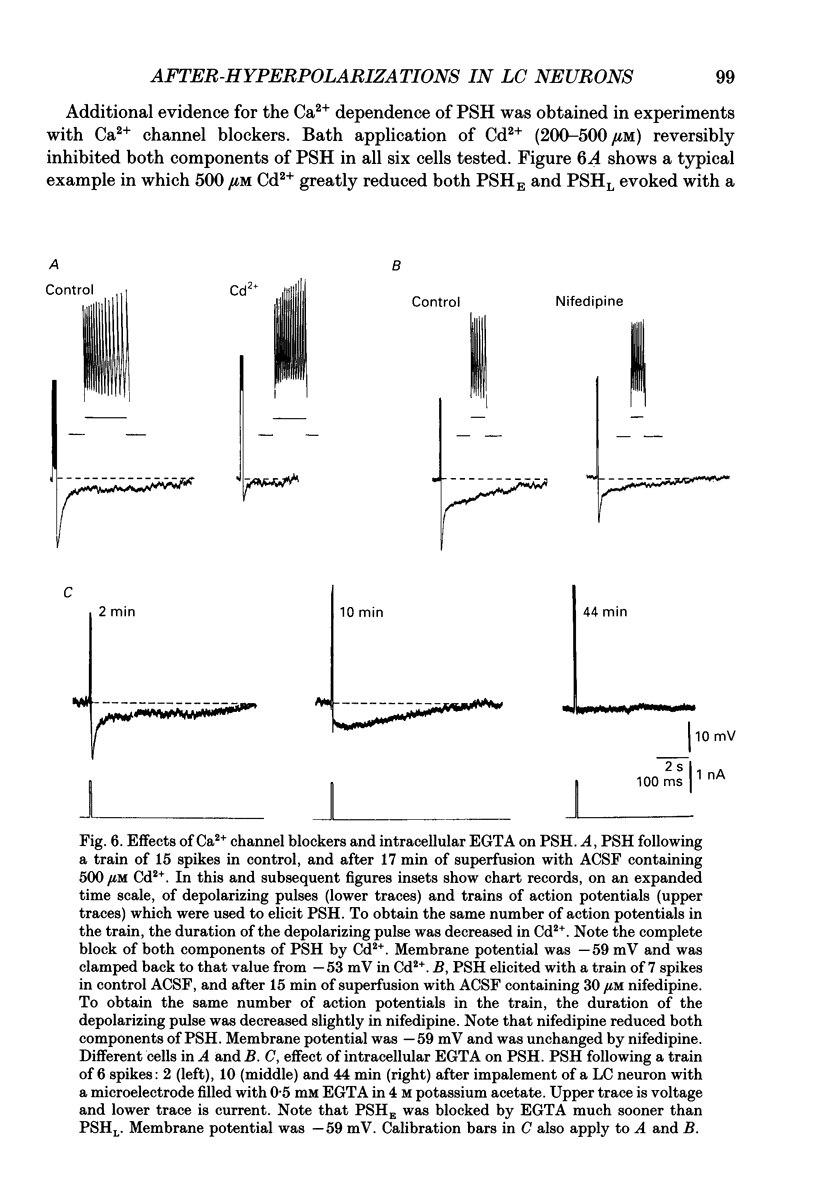
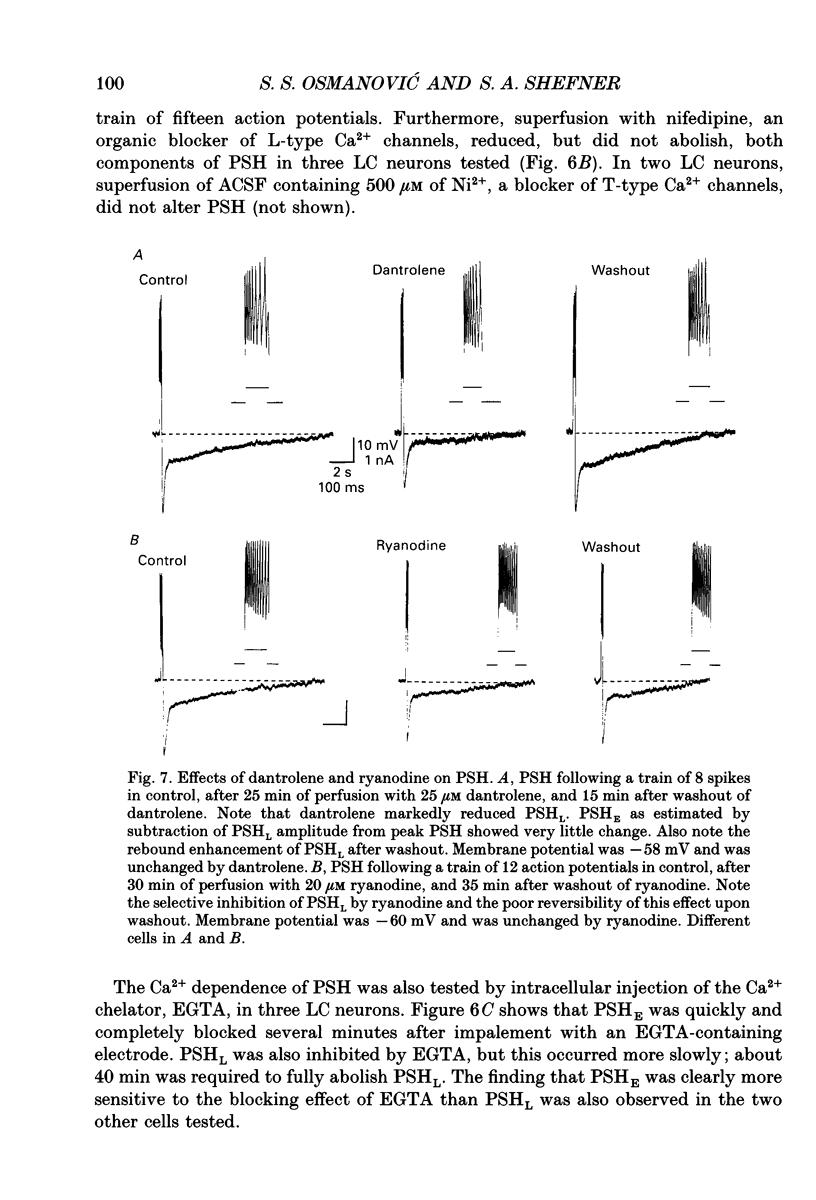
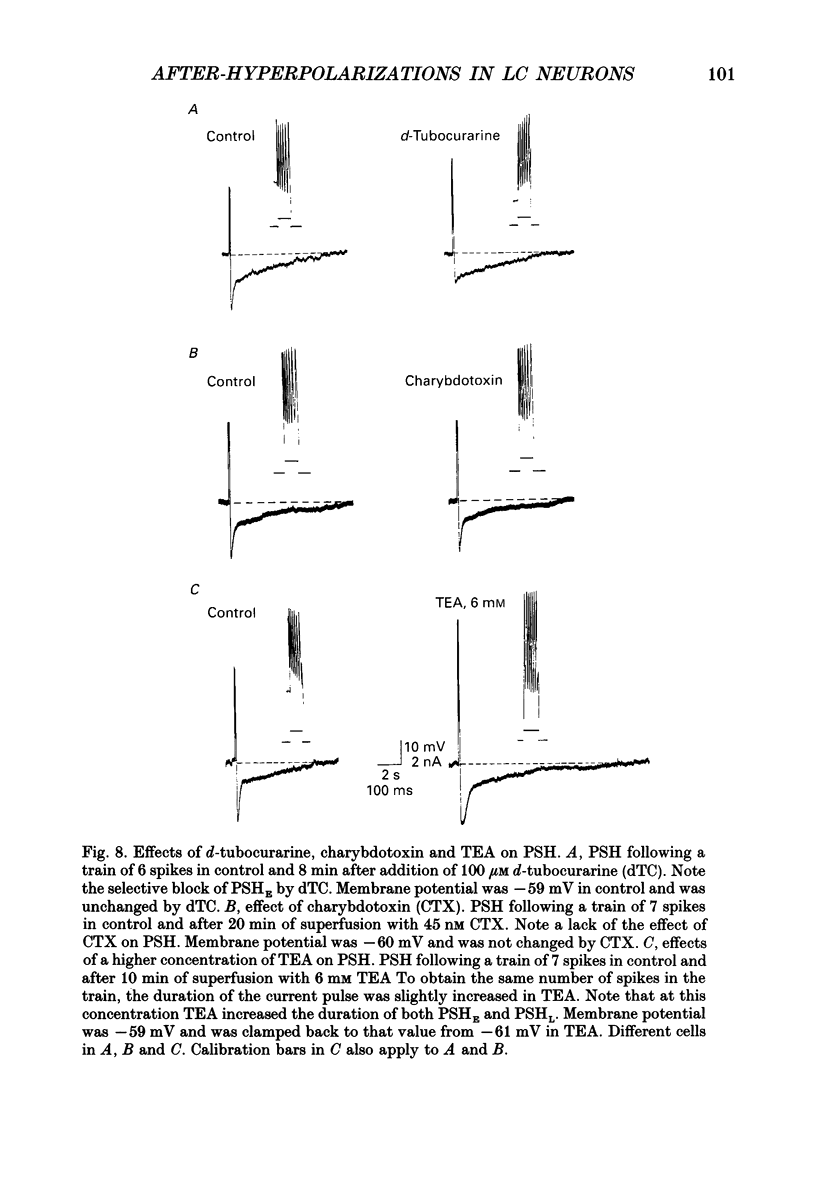
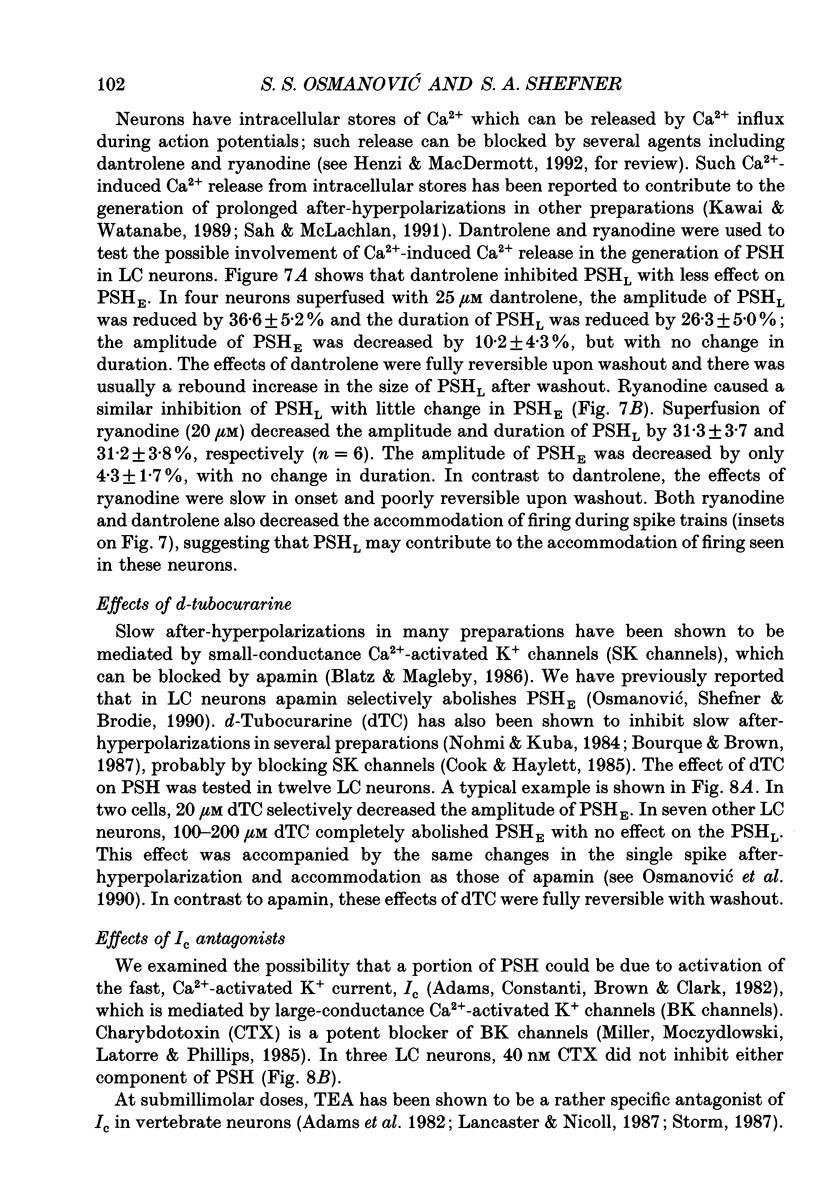
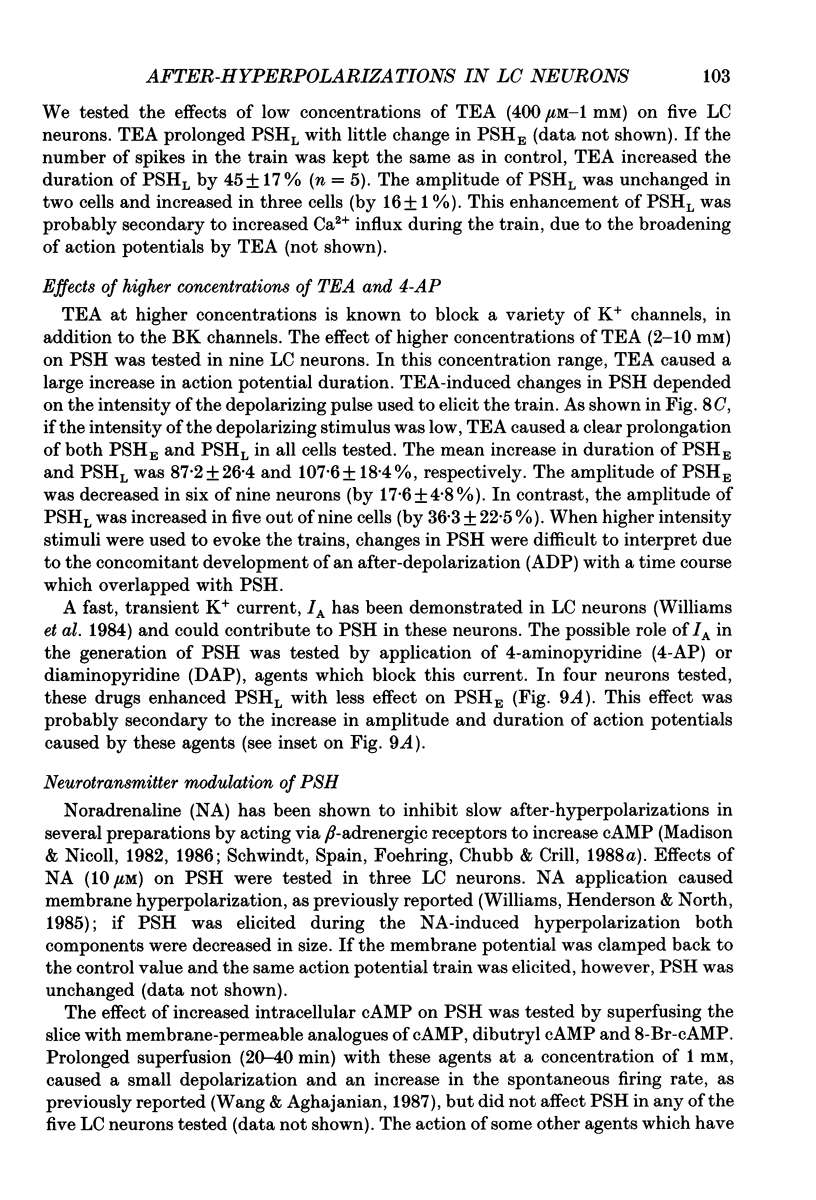
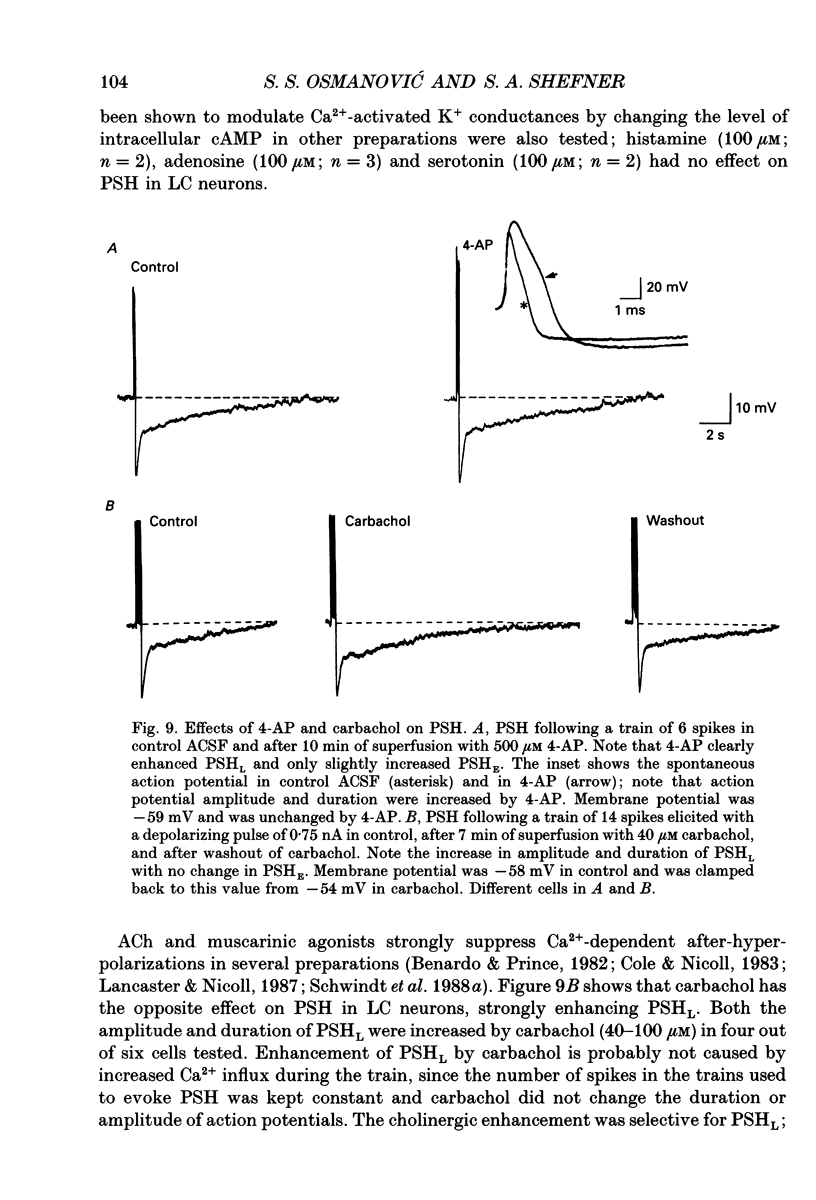
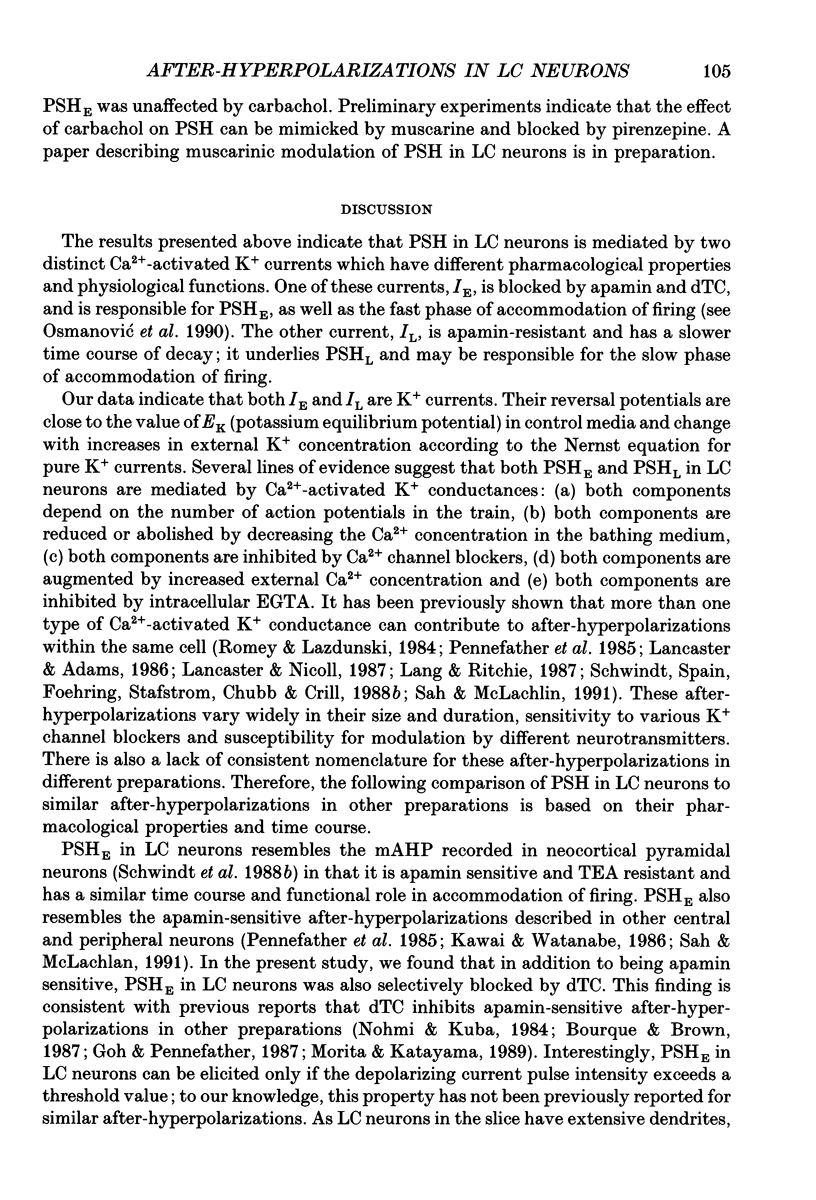
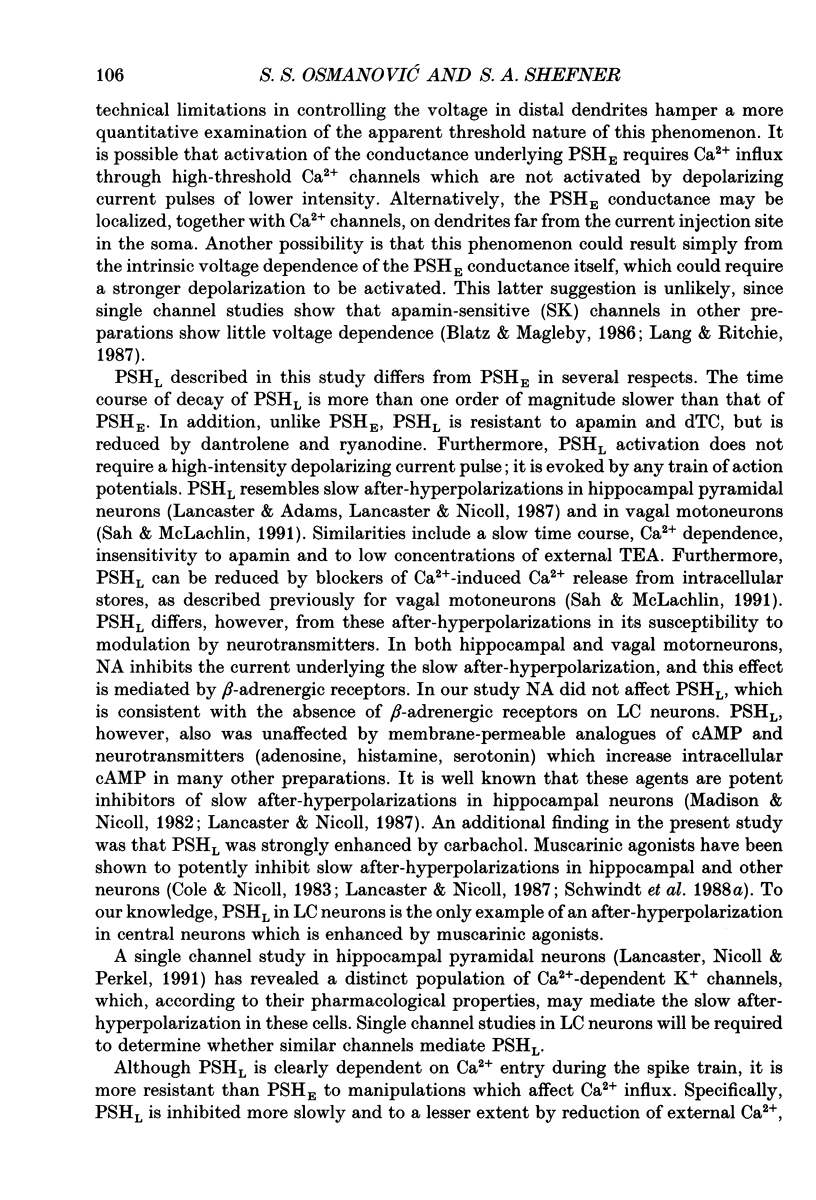
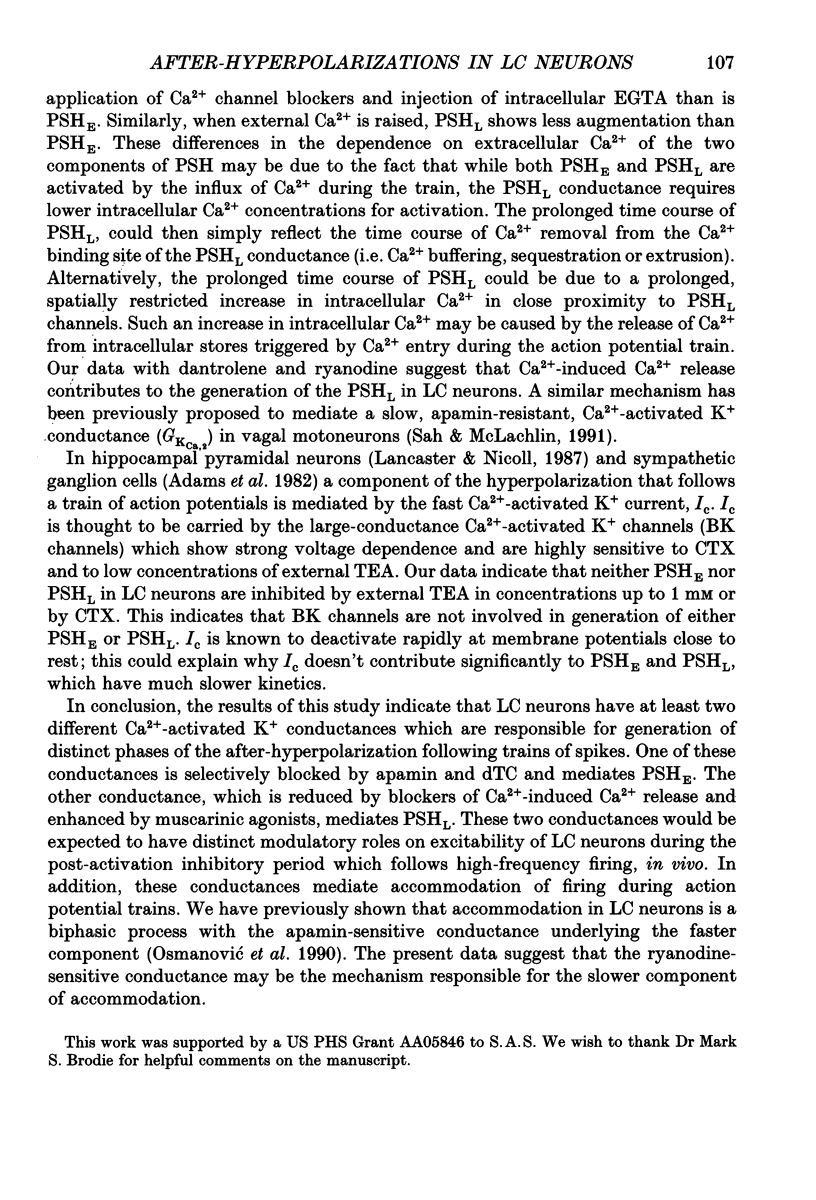
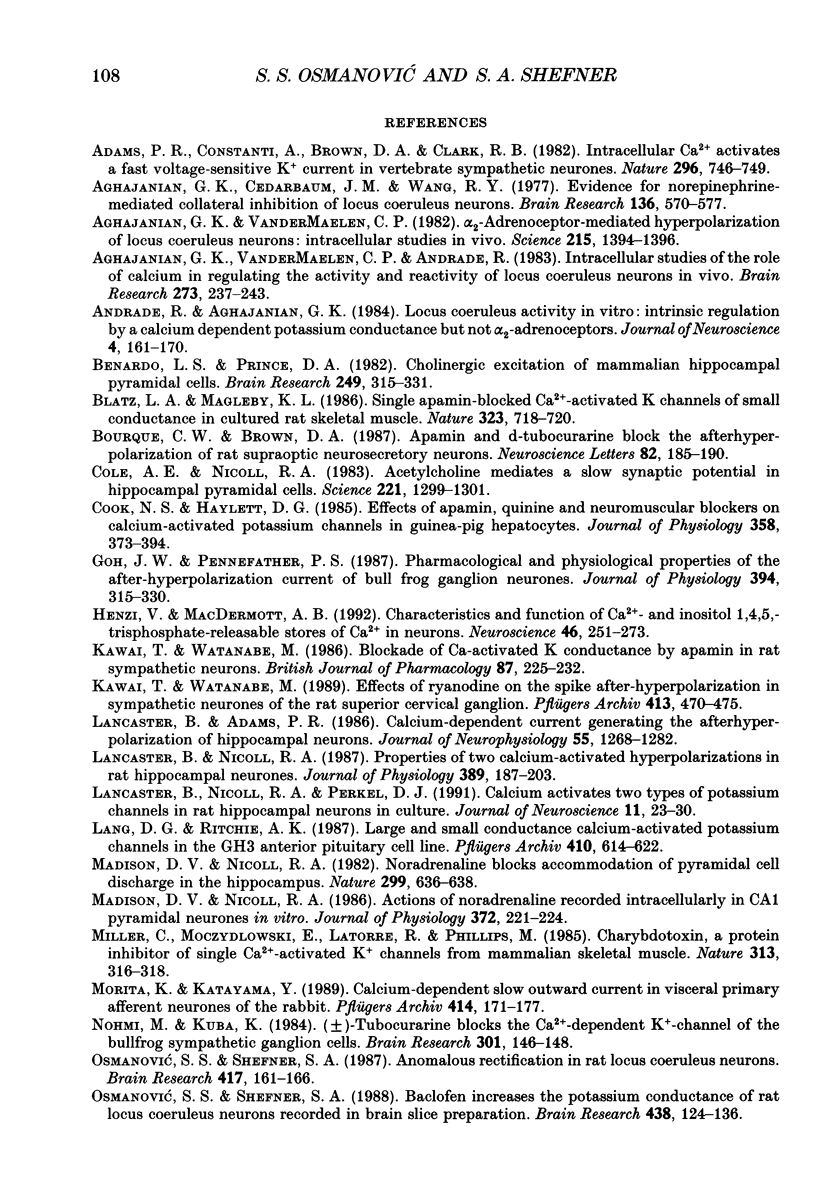
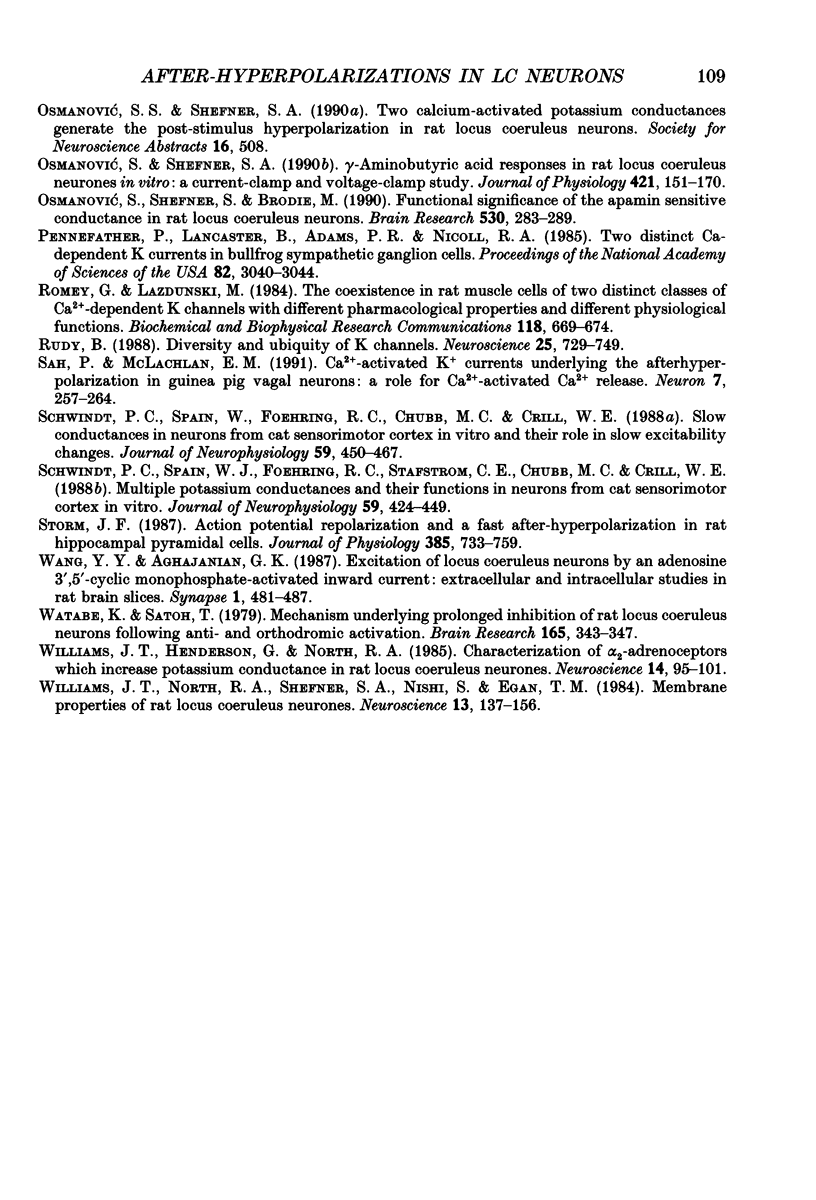
Selected References
These references are in PubMed. This may not be the complete list of references from this article.
- Adams P. R., Constanti A., Brown D. A., Clark R. B. Intracellular Ca2+ activates a fast voltage-sensitive K+ current in vertebrate sympathetic neurones. Nature. 1982 Apr 22;296(5859):746–749. doi: 10.1038/296746a0. [DOI] [PubMed] [Google Scholar]
- Aghajanian G. K., Cedarbaum J. M., Wang R. Y. Evidence for norepinephrine-mediated collateral inhibition of locus coeruleus neurons. Brain Res. 1977 Nov 18;136(3):570–577. doi: 10.1016/0006-8993(77)90083-x. [DOI] [PubMed] [Google Scholar]
- Aghajanian G. K., VanderMaelen C. P. alpha 2-adrenoceptor-mediated hyperpolarization of locus coeruleus neurons: intracellular studies in vivo. Science. 1982 Mar 12;215(4538):1394–1396. doi: 10.1126/science.6278591. [DOI] [PubMed] [Google Scholar]
- Aghajanian G. K., Vandermaelen C. P., Andrade R. Intracellular studies on the role of calcium in regulating the activity and reactivity of locus coeruleus neurons in vivo. Brain Res. 1983 Aug 29;273(2):237–243. doi: 10.1016/0006-8993(83)90848-x. [DOI] [PubMed] [Google Scholar]
- Andrade R., Aghajanian G. K. Locus coeruleus activity in vitro: intrinsic regulation by a calcium-dependent potassium conductance but not alpha 2-adrenoceptors. J Neurosci. 1984 Jan;4(1):161–170. doi: 10.1523/JNEUROSCI.04-01-00161.1984. [DOI] [PMC free article] [PubMed] [Google Scholar]
- Benardo L. S., Prince D. A. Cholinergic excitation of mammalian hippocampal pyramidal cells. Brain Res. 1982 Oct 14;249(2):315–331. doi: 10.1016/0006-8993(82)90066-x. [DOI] [PubMed] [Google Scholar]
- Blatz A. L., Magleby K. L. Single apamin-blocked Ca-activated K+ channels of small conductance in cultured rat skeletal muscle. Nature. 1986 Oct 23;323(6090):718–720. doi: 10.1038/323718a0. [DOI] [PubMed] [Google Scholar]
- Bourque C. W., Brown D. A. Apamin and d-tubocurarine block the afterhyperpolarization of rat supraoptic neurosecretory neurons. Neurosci Lett. 1987 Nov 23;82(2):185–190. doi: 10.1016/0304-3940(87)90127-3. [DOI] [PubMed] [Google Scholar]
- Cole A. E., Nicoll R. A. Acetylcholine mediates a slow synaptic potential in hippocampal pyramidal cells. Science. 1983 Sep 23;221(4617):1299–1301. doi: 10.1126/science.6612345. [DOI] [PubMed] [Google Scholar]
- Cook N. S., Haylett D. G. Effects of apamin, quinine and neuromuscular blockers on calcium-activated potassium channels in guinea-pig hepatocytes. J Physiol. 1985 Jan;358:373–394. doi: 10.1113/jphysiol.1985.sp015556. [DOI] [PMC free article] [PubMed] [Google Scholar]
- Goh J. W., Pennefather P. S. Pharmacological and physiological properties of the after-hyperpolarization current of bullfrog ganglion neurones. J Physiol. 1987 Dec;394:315–330. doi: 10.1113/jphysiol.1987.sp016872. [DOI] [PMC free article] [PubMed] [Google Scholar]
- Henzi V., MacDermott A. B. Characteristics and function of Ca(2+)- and inositol 1,4,5-trisphosphate-releasable stores of Ca2+ in neurons. Neuroscience. 1992;46(2):251–273. doi: 10.1016/0306-4522(92)90049-8. [DOI] [PubMed] [Google Scholar]
- Kawai T., Watanabe M. Blockade of Ca-activated K conductance by apamin in rat sympathetic neurones. Br J Pharmacol. 1986 Jan;87(1):225–232. doi: 10.1111/j.1476-5381.1986.tb10175.x. [DOI] [PMC free article] [PubMed] [Google Scholar]
- Kawai T., Watanabe M. Effects of ryanodine on the spike after-hyperpolarization in sympathetic neurones of the rat superior cervical ganglion. Pflugers Arch. 1989 Mar;413(5):470–475. doi: 10.1007/BF00594175. [DOI] [PubMed] [Google Scholar]
- Lancaster B., Adams P. R. Calcium-dependent current generating the afterhyperpolarization of hippocampal neurons. J Neurophysiol. 1986 Jun;55(6):1268–1282. doi: 10.1152/jn.1986.55.6.1268. [DOI] [PubMed] [Google Scholar]
- Lancaster B., Nicoll R. A., Perkel D. J. Calcium activates two types of potassium channels in rat hippocampal neurons in culture. J Neurosci. 1991 Jan;11(1):23–30. doi: 10.1523/JNEUROSCI.11-01-00023.1991. [DOI] [PMC free article] [PubMed] [Google Scholar]
- Lancaster B., Nicoll R. A. Properties of two calcium-activated hyperpolarizations in rat hippocampal neurones. J Physiol. 1987 Aug;389:187–203. doi: 10.1113/jphysiol.1987.sp016653. [DOI] [PMC free article] [PubMed] [Google Scholar]
- Lang D. G., Ritchie A. K. Large and small conductance calcium-activated potassium channels in the GH3 anterior pituitary cell line. Pflugers Arch. 1987 Dec;410(6):614–622. doi: 10.1007/BF00581321. [DOI] [PubMed] [Google Scholar]
- Madison D. V., Nicoll R. A. Actions of noradrenaline recorded intracellularly in rat hippocampal CA1 pyramidal neurones, in vitro. J Physiol. 1986 Mar;372:221–244. doi: 10.1113/jphysiol.1986.sp016006. [DOI] [PMC free article] [PubMed] [Google Scholar]
- Madison D. V., Nicoll R. A. Noradrenaline blocks accommodation of pyramidal cell discharge in the hippocampus. Nature. 1982 Oct 14;299(5884):636–638. doi: 10.1038/299636a0. [DOI] [PubMed] [Google Scholar]
- Miller C., Moczydlowski E., Latorre R., Phillips M. Charybdotoxin, a protein inhibitor of single Ca2+-activated K+ channels from mammalian skeletal muscle. Nature. 1985 Jan 24;313(6000):316–318. doi: 10.1038/313316a0. [DOI] [PubMed] [Google Scholar]
- Morita K., Katayama Y. Calcium-dependent slow outward current in visceral primary afferent neurones of the rabbit. Pflugers Arch. 1989 Jun;414(2):171–177. doi: 10.1007/BF00580960. [DOI] [PubMed] [Google Scholar]
- Nohmi M., Kuba K. (+)-Tubocurarine blocks the Ca2+-dependent K+-channel of the bullfrog sympathetic ganglion cell. Brain Res. 1984 May 28;301(1):146–148. doi: 10.1016/0006-8993(84)90412-8. [DOI] [PubMed] [Google Scholar]
- Osmanović S. S., Shefner S. A. Anomalous rectification in rat locus coeruleus neurons. Brain Res. 1987 Aug 4;417(1):161–166. doi: 10.1016/0006-8993(87)90193-4. [DOI] [PubMed] [Google Scholar]
- Osmanović S. S., Shefner S. A. Baclofen increases the potassium conductance of rat locus coeruleus neurons recorded in brain slices. Brain Res. 1988 Jan 12;438(1-2):124–136. doi: 10.1016/0006-8993(88)91331-5. [DOI] [PubMed] [Google Scholar]
- Osmanović S. S., Shefner S. A., Brodie M. S. Functional significance of the apamin-sensitive conductance in rat locus coeruleus neurons. Brain Res. 1990 Oct 22;530(2):283–289. doi: 10.1016/0006-8993(90)91296-s. [DOI] [PubMed] [Google Scholar]
- Osmanović S. S., Shefner S. A. gamma-Aminobutyric acid responses in rat locus coeruleus neurones in vitro: a current-clamp and voltage-clamp study. J Physiol. 1990 Feb;421:151–170. doi: 10.1113/jphysiol.1990.sp017938. [DOI] [PMC free article] [PubMed] [Google Scholar]
- Pennefather P., Lancaster B., Adams P. R., Nicoll R. A. Two distinct Ca-dependent K currents in bullfrog sympathetic ganglion cells. Proc Natl Acad Sci U S A. 1985 May;82(9):3040–3044. doi: 10.1073/pnas.82.9.3040. [DOI] [PMC free article] [PubMed] [Google Scholar]
- Romey G., Lazdunski M. The coexistence in rat muscle cells of two distinct classes of Ca2+-dependent K+ channels with different pharmacological properties and different physiological functions. Biochem Biophys Res Commun. 1984 Jan 30;118(2):669–674. doi: 10.1016/0006-291x(84)91355-x. [DOI] [PubMed] [Google Scholar]
- Rudy B. Diversity and ubiquity of K channels. Neuroscience. 1988 Jun;25(3):729–749. doi: 10.1016/0306-4522(88)90033-4. [DOI] [PubMed] [Google Scholar]
- Sah P., McLachlan E. M. Ca(2+)-activated K+ currents underlying the afterhyperpolarization in guinea pig vagal neurons: a role for Ca(2+)-activated Ca2+ release. Neuron. 1991 Aug;7(2):257–264. doi: 10.1016/0896-6273(91)90264-z. [DOI] [PubMed] [Google Scholar]
- Schwindt P. C., Spain W. J., Foehring R. C., Chubb M. C., Crill W. E. Slow conductances in neurons from cat sensorimotor cortex in vitro and their role in slow excitability changes. J Neurophysiol. 1988 Feb;59(2):450–467. doi: 10.1152/jn.1988.59.2.450. [DOI] [PubMed] [Google Scholar]
- Schwindt P. C., Spain W. J., Foehring R. C., Stafstrom C. E., Chubb M. C., Crill W. E. Multiple potassium conductances and their functions in neurons from cat sensorimotor cortex in vitro. J Neurophysiol. 1988 Feb;59(2):424–449. doi: 10.1152/jn.1988.59.2.424. [DOI] [PubMed] [Google Scholar]
- Storm J. F. Action potential repolarization and a fast after-hyperpolarization in rat hippocampal pyramidal cells. J Physiol. 1987 Apr;385:733–759. doi: 10.1113/jphysiol.1987.sp016517. [DOI] [PMC free article] [PubMed] [Google Scholar]
- Wang Y. Y., Aghajanian G. K. Excitation of locus coeruleus neurons by an adenosine 3',5'-cyclic monophosphate-activated inward current: extracellular and intracellular studies in rat brain slices. Synapse. 1987;1(5):481–487. doi: 10.1002/syn.890010512. [DOI] [PubMed] [Google Scholar]
- Watabe K., Satoh T. Mechanism underlying prolonged inhibition of rat locus coeruleus neurons following anti- and orthodromic activation. Brain Res. 1979 Apr 13;165(2):343–347. doi: 10.1016/0006-8993(79)90567-5. [DOI] [PubMed] [Google Scholar]
- Williams J. T., Henderson G., North R. A. Characterization of alpha 2-adrenoceptors which increase potassium conductance in rat locus coeruleus neurones. Neuroscience. 1985 Jan;14(1):95–101. doi: 10.1016/0306-4522(85)90166-6. [DOI] [PubMed] [Google Scholar]
- Williams J. T., North R. A., Shefner S. A., Nishi S., Egan T. M. Membrane properties of rat locus coeruleus neurones. Neuroscience. 1984 Sep;13(1):137–156. doi: 10.1016/0306-4522(84)90265-3. [DOI] [PubMed] [Google Scholar]