Abstract
1. Whole-cell patch clamp and optical recording techniques were applied to the same in vitro salamander olfactory bulb preparations to study the postsynaptic responses of single mitral/tufted cells in the context of the surrounding neural activity in which they are embedded. Mitral/tufted cells were identified by intracellular filling with biocytin. 2. Single mitral/tufted cells were under a tonic GABAA receptor-mediated inhibitory influence as revealed by the recording of bicuculline methiodide (BMI)/picrotoxin-sensitive inhibitory postsynaptic currents (IPSCs) in symmetrical chloride conditions at a holding potential of -70 mV. Depolarizing voltage steps (100 ms) applied to single cells or electrical stimulation of the olfactory nerve or medial olfactory tract evoked a prolonged increase in the frequency of GABAergic IPSCs. 3. The frequency of spontaneous and driven IPSCs was reduced with application of the glutamate receptor antagonists 6-cyano-2,3-dihydroxy-7-nitro-quionoxaline (CNQX) or 2-amino-5-phosphonopentanoic acid (AP5) whereas olfactory nerve- or medial olfactory tract-driven IPSC frequency was enhanced with removal of bathing Mg2+, indicating that GABAergic interneurones were driven by mitral/tufted cells at both non-NMDA and NMDA receptors. 4. Olfactory nerve or medial olfactory tract stimulation evoked widely distributed changes in fluorescence in preparations stained with the voltage-sensitive dye RH414. The optical response predominantly consisted of a decrease in fluorescence, indicative of depolarization. The presence of the dye did not obviously affect mitral/tufted cell postsynaptic responses. 5. BMI enhanced the amplitude and duration of optical signals related to depolarization within the bulb and in regions central to the bulb. In the presence of BMI, depolarizing activity appeared to spread hundreds of micrometres into regions of the bulb not activated in control conditions showing explicitly that GABAA receptors in the bulb participate in lateral inhibition. 6. CNQX and AP5 attenuated the optical signals within the bulb supporting the contention that in these conditions, optical signals arise mainly from granule cell dendritic activity. Furthermore, AP5 or removal of bath Mg2+ reduced or enlarged the spatial distribution of activity respectively, suggesting that in some cases the NMDA receptor may be involved in generating or stabilizing spatial patterns of activity. 7. It is concluded that in the salamander olfactory bulb, both GABAA- and glutamate receptor-mediated synaptic transmission shape the different temporal and spatial patterns of neural activity associated with olfactory coding.
Full text
PDF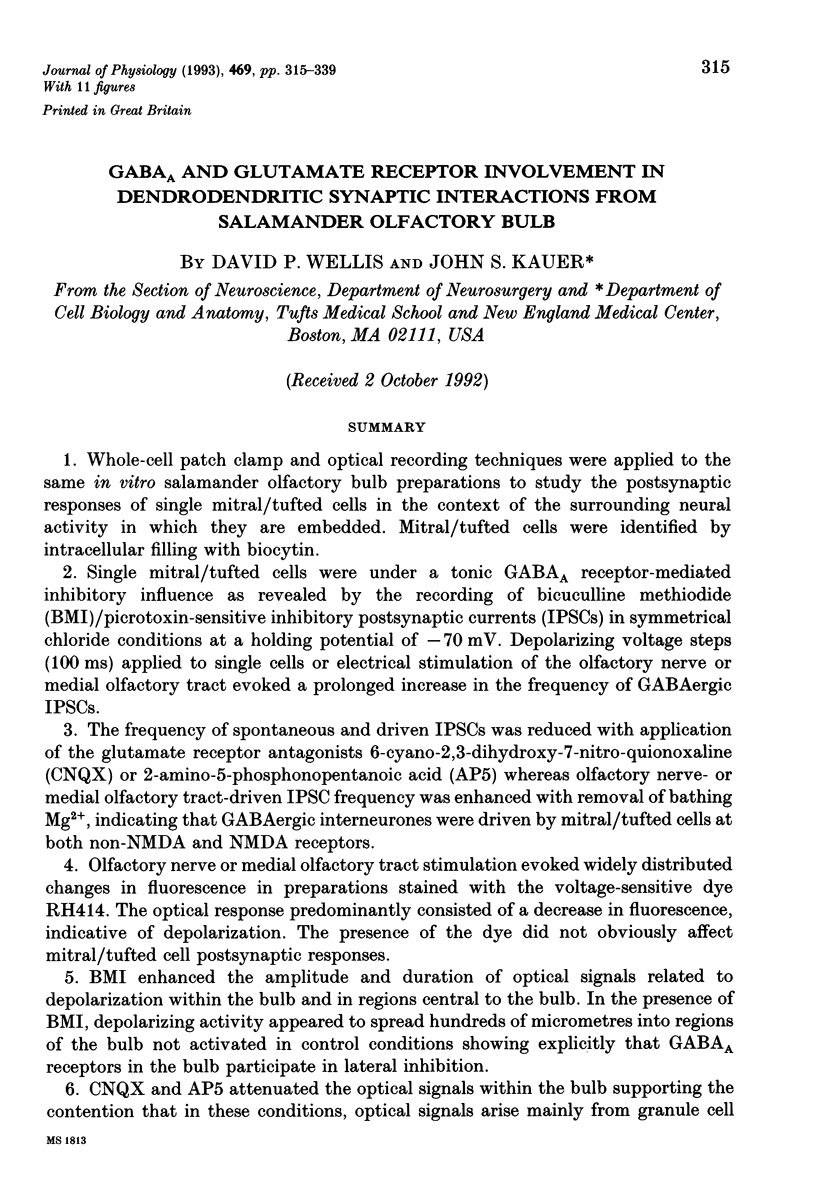
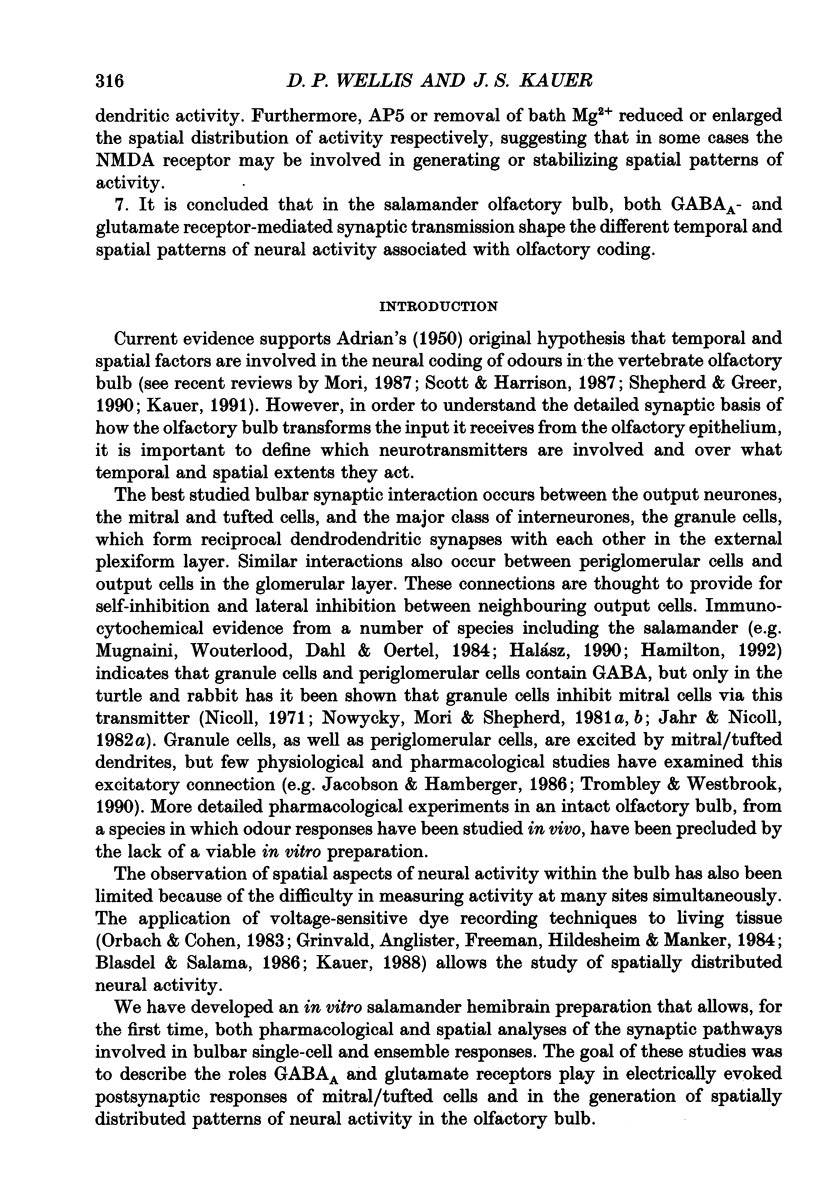
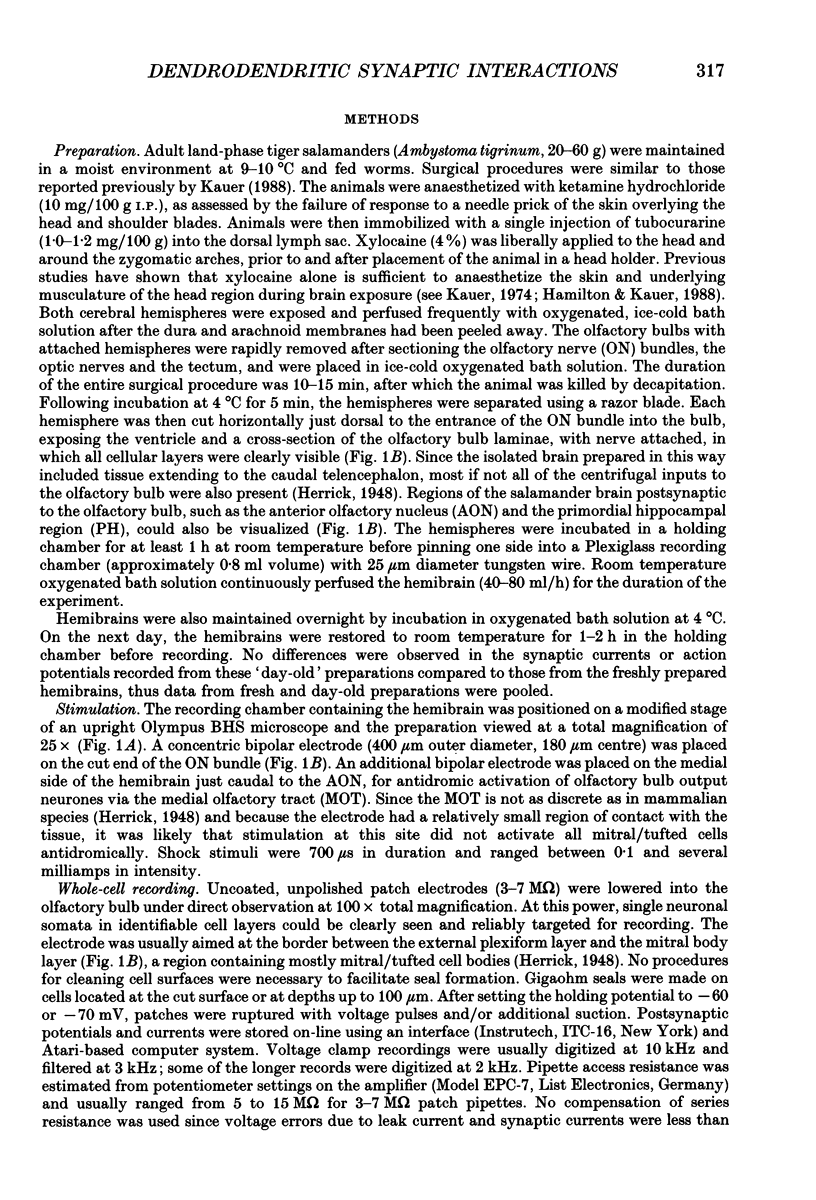
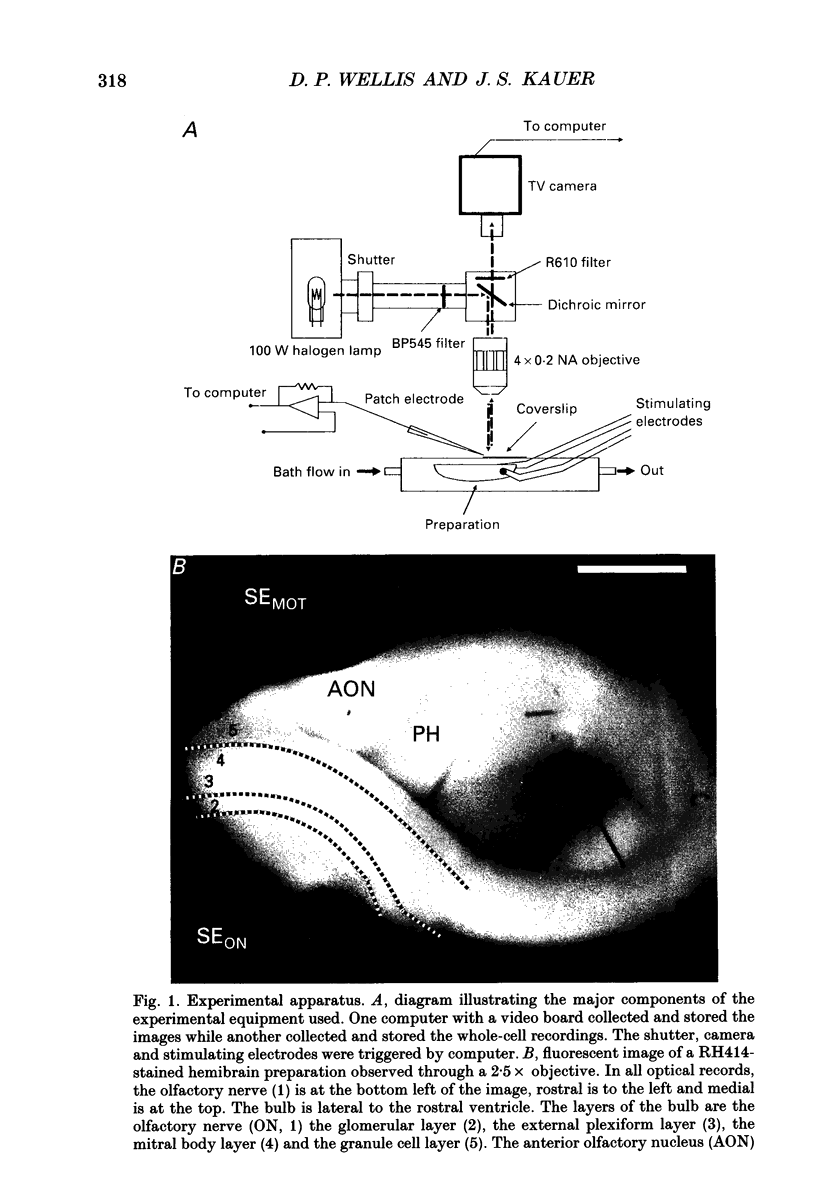
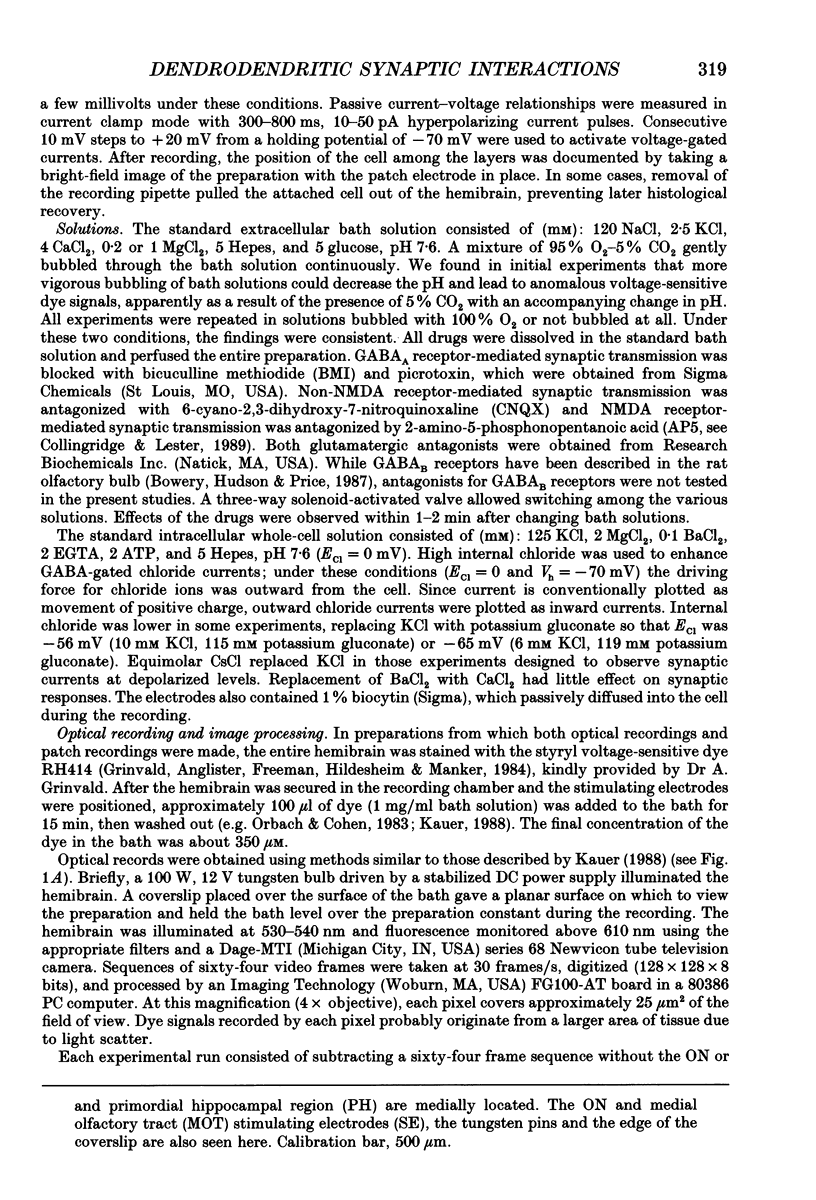
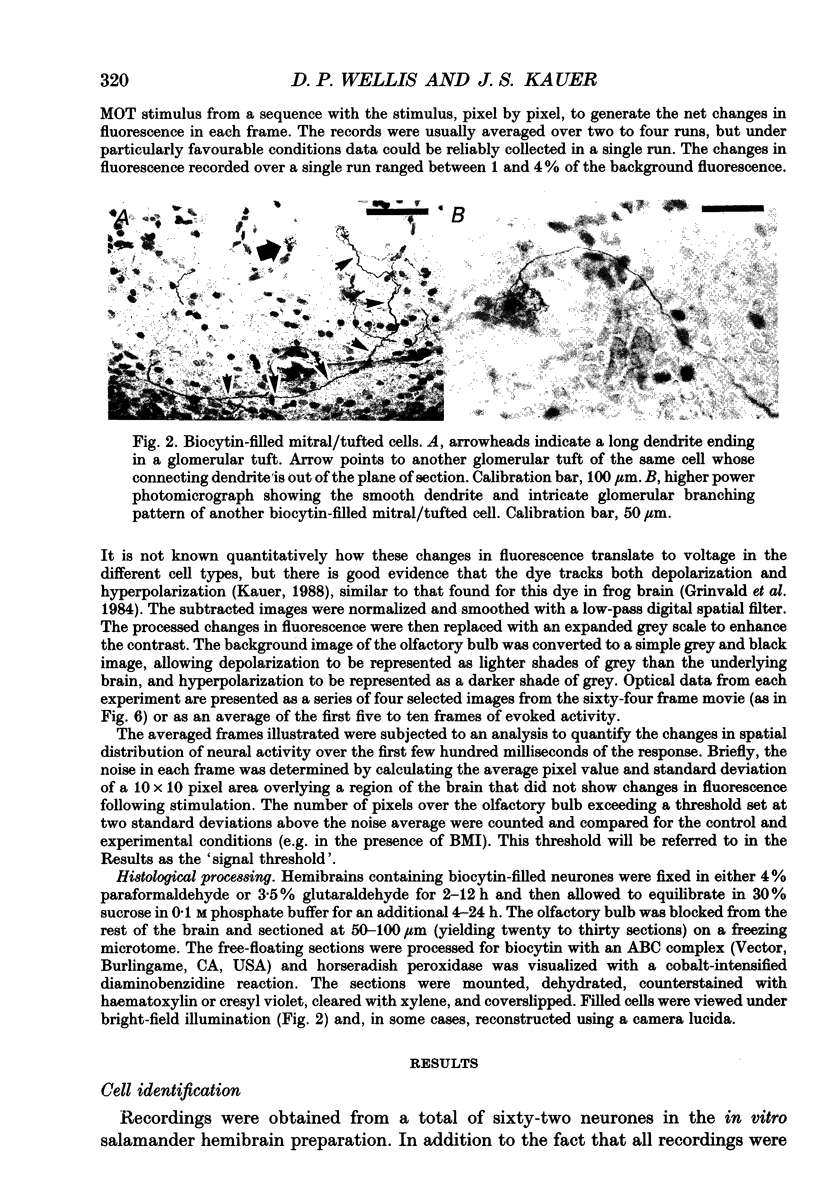
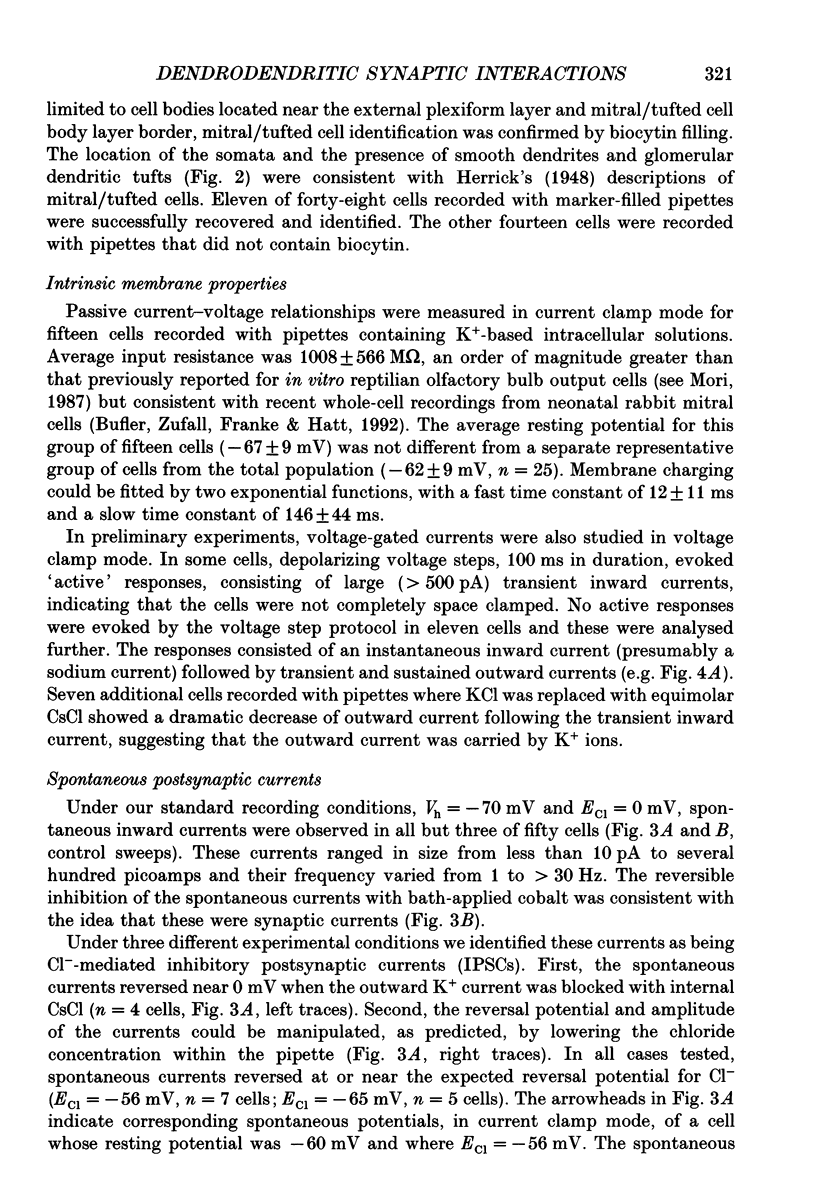
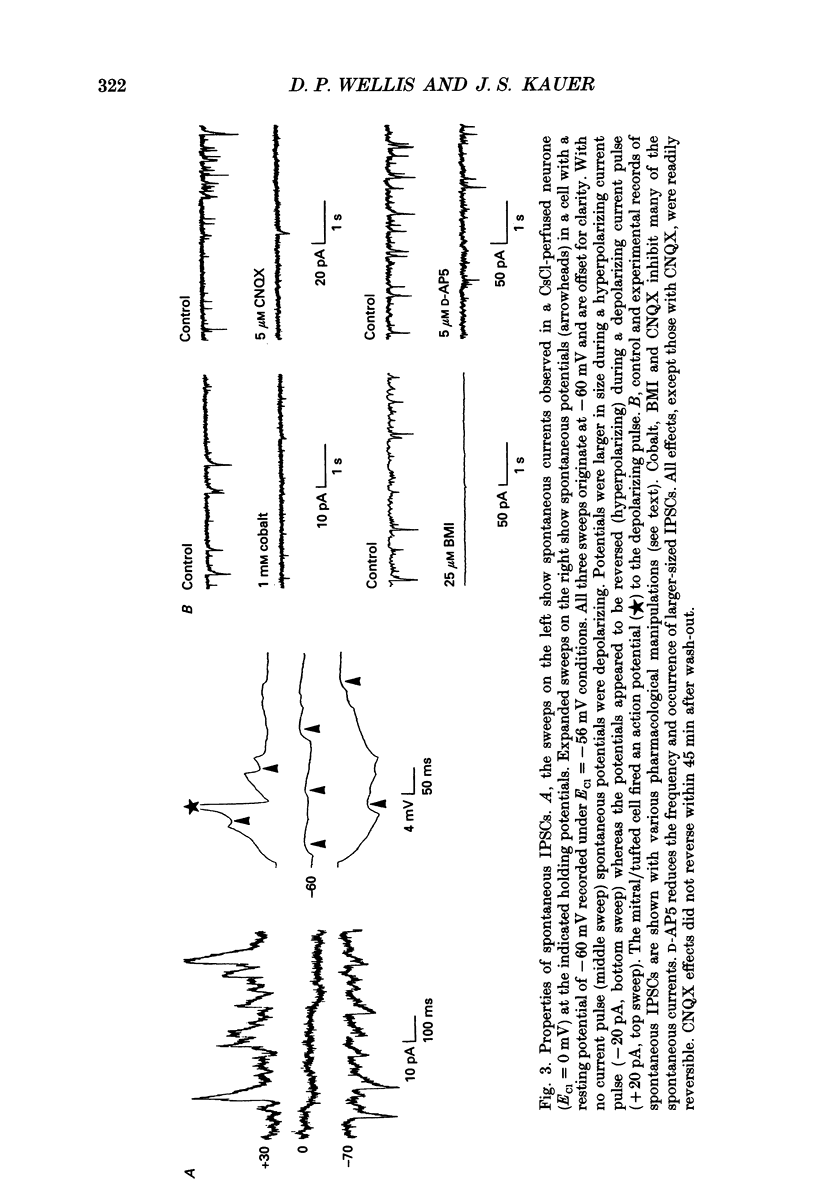
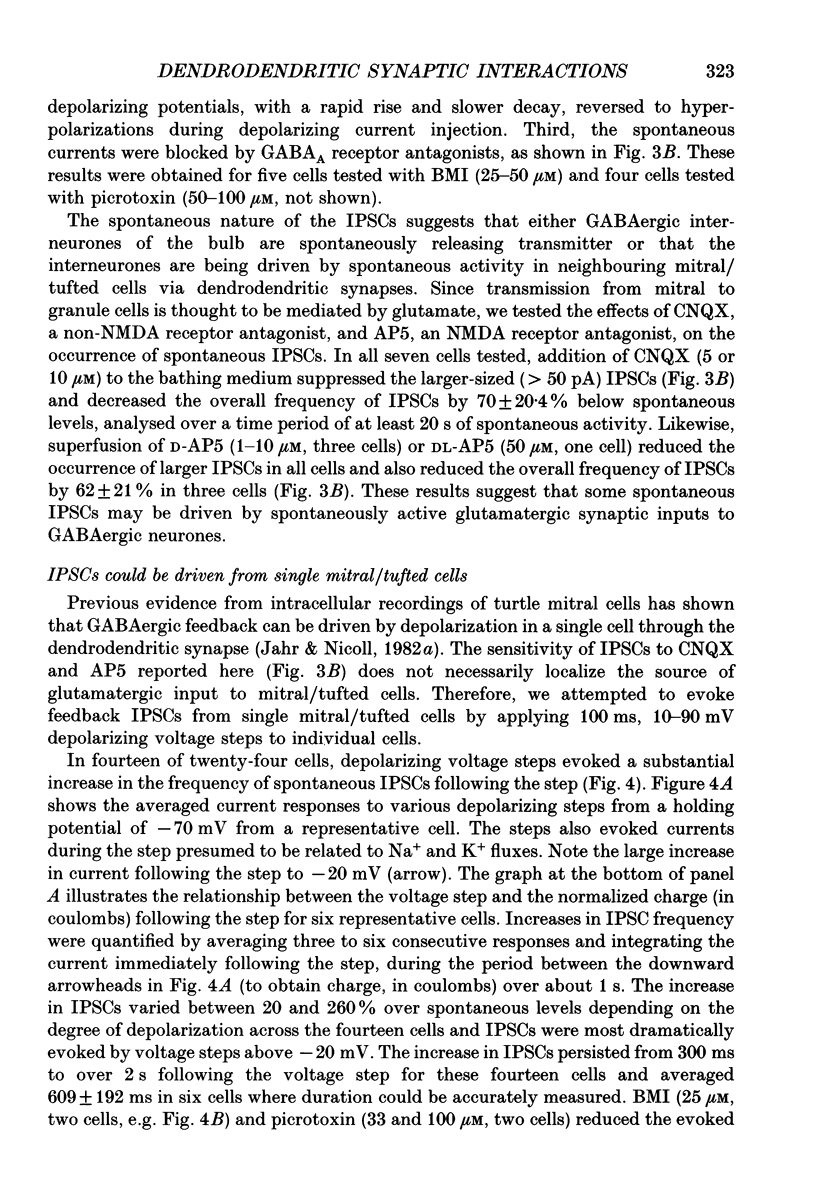
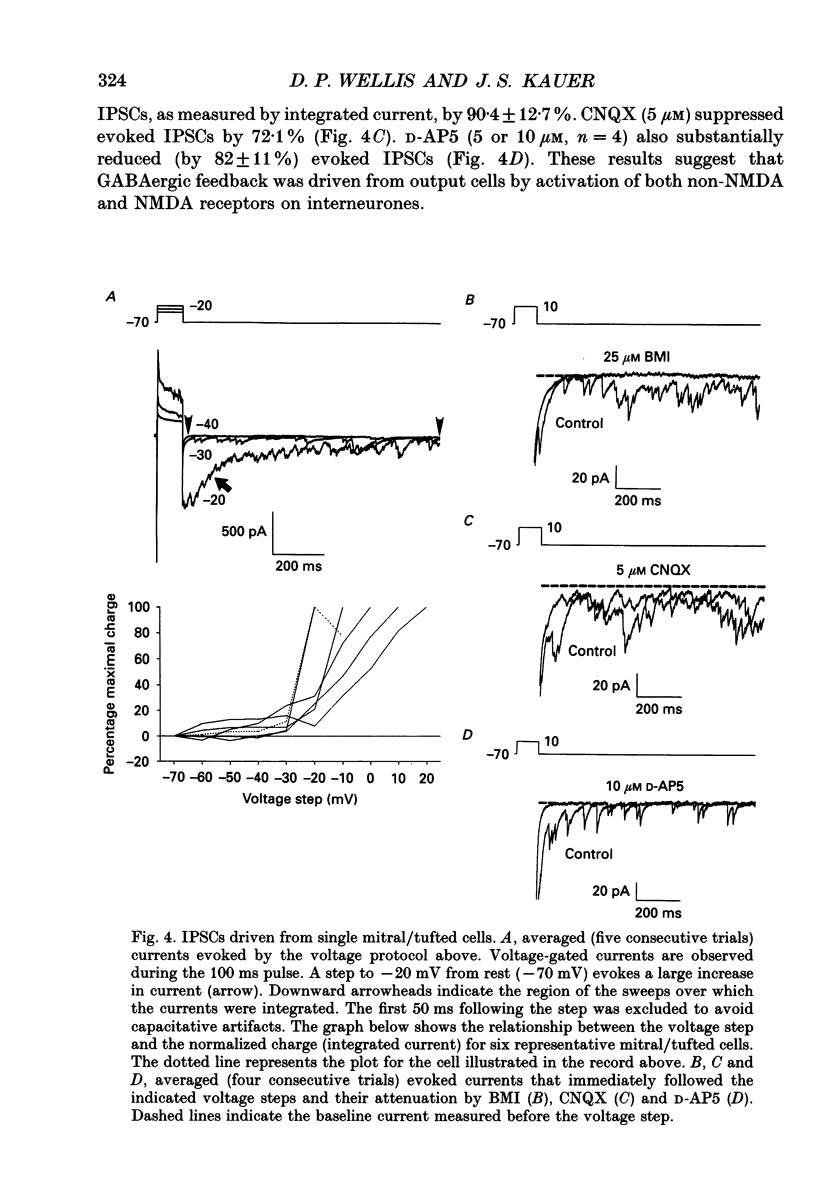
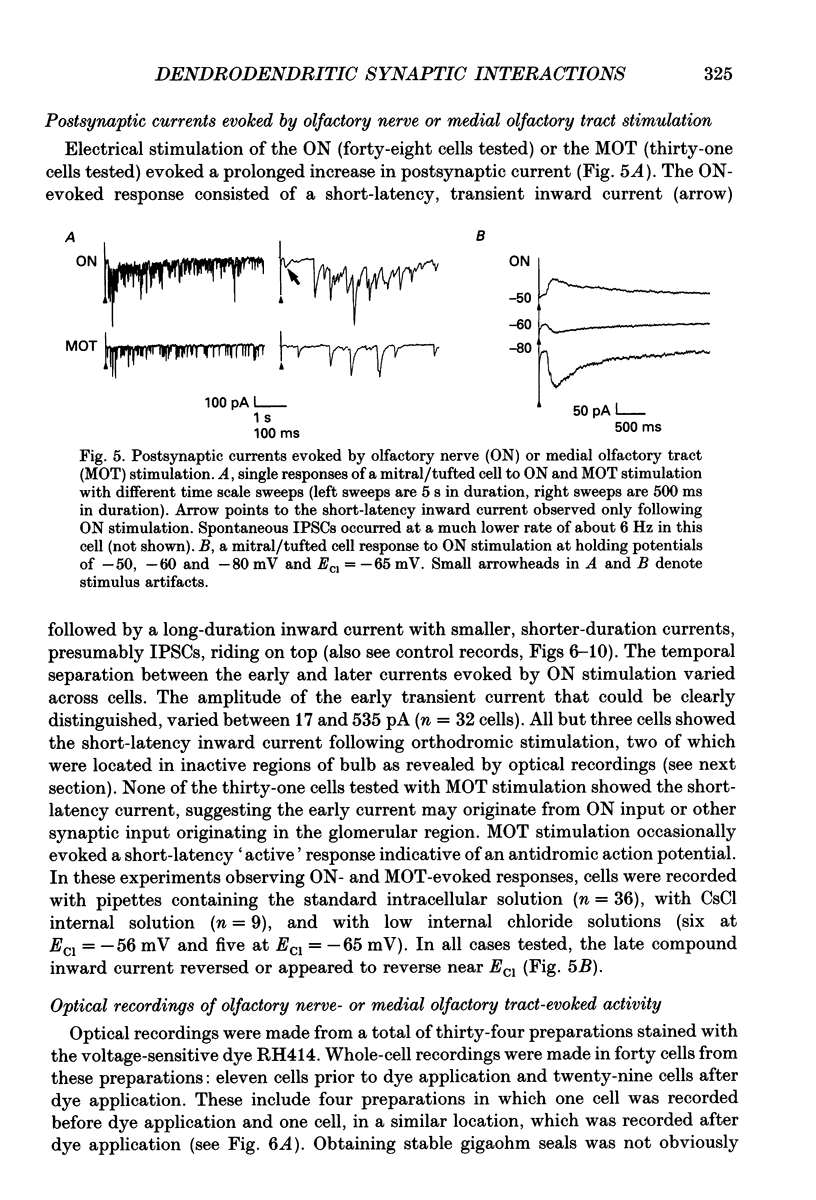
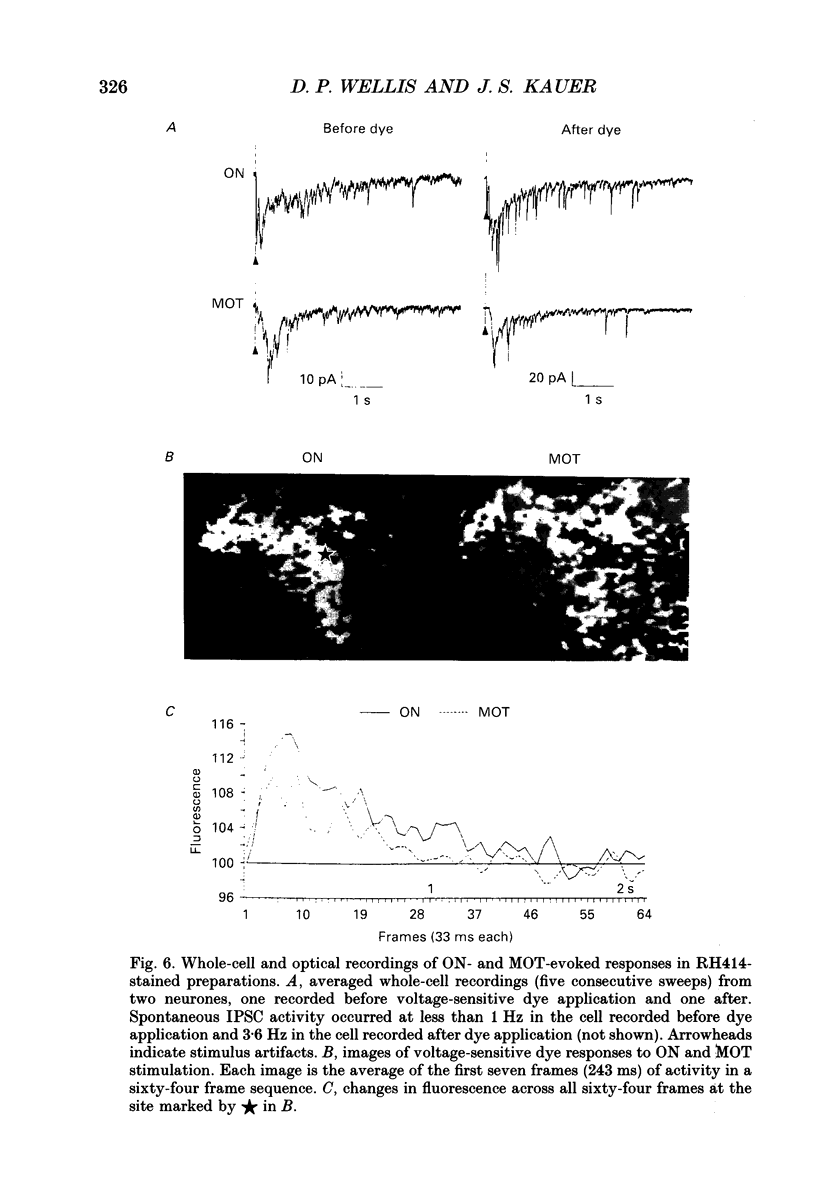
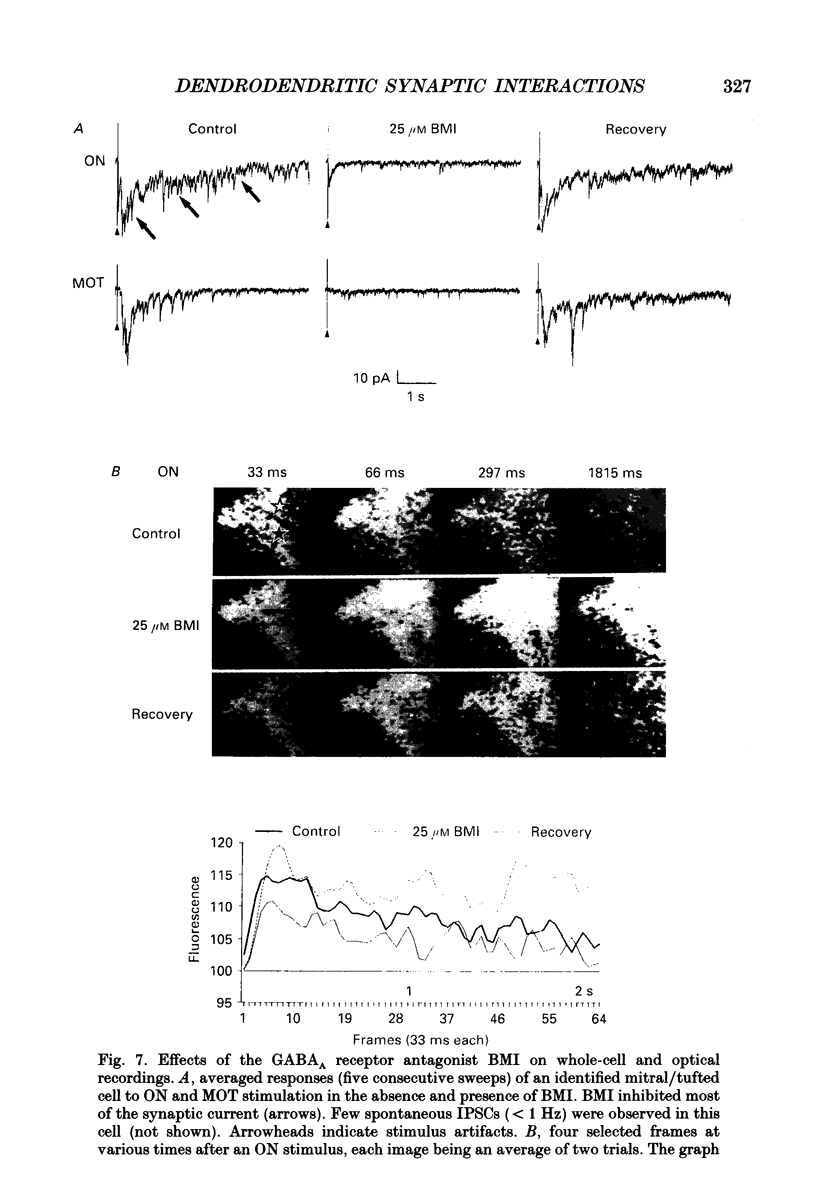
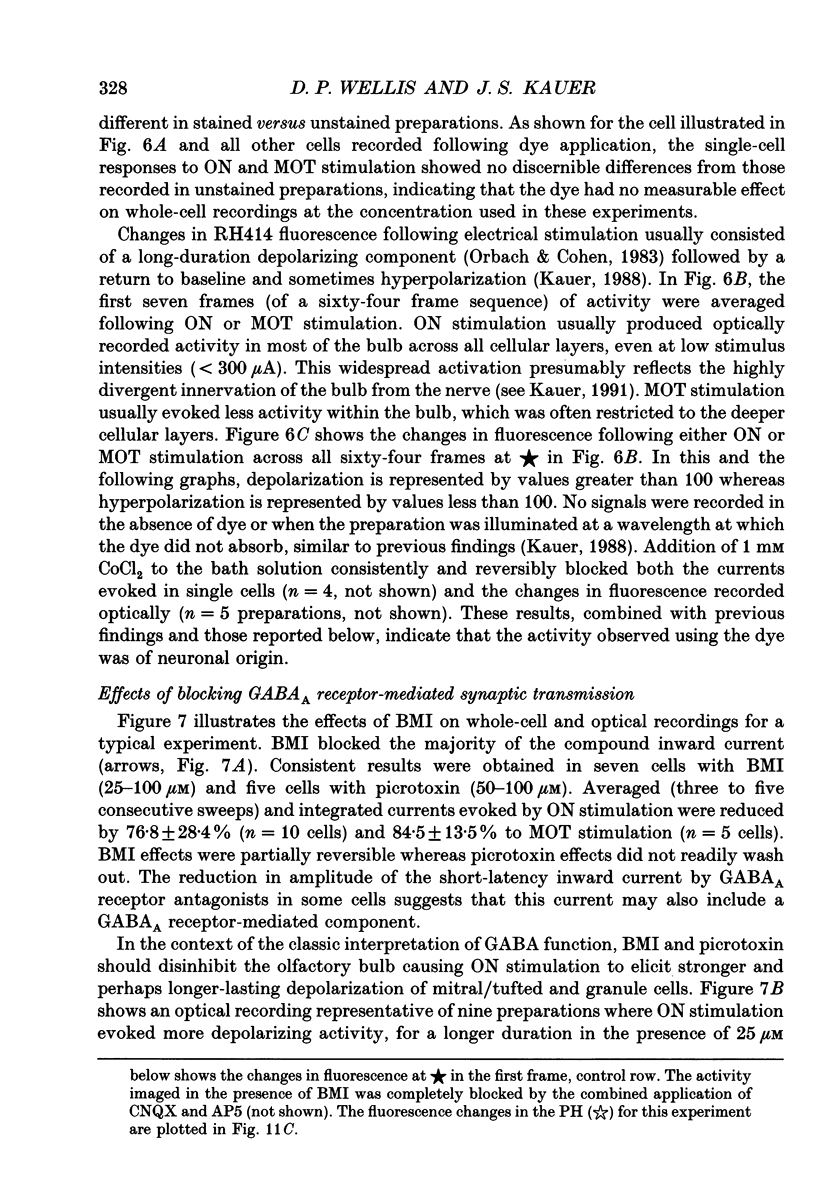
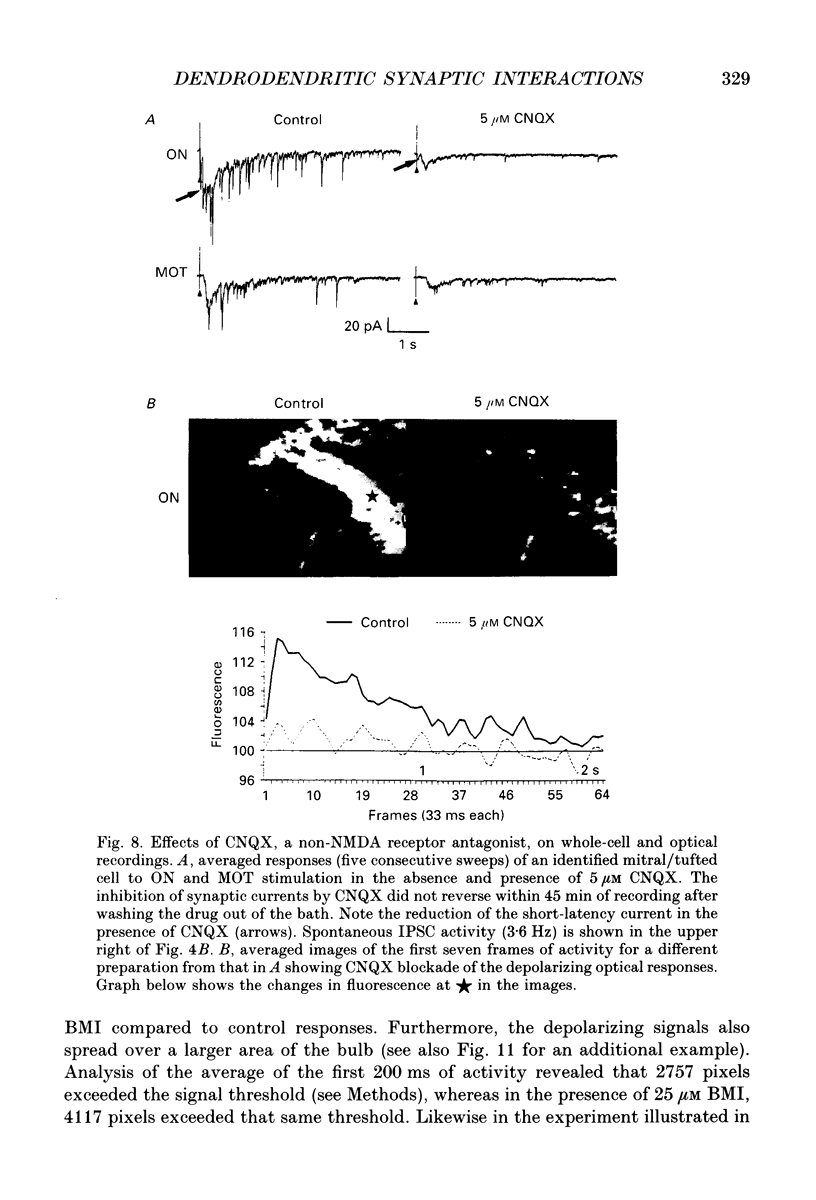
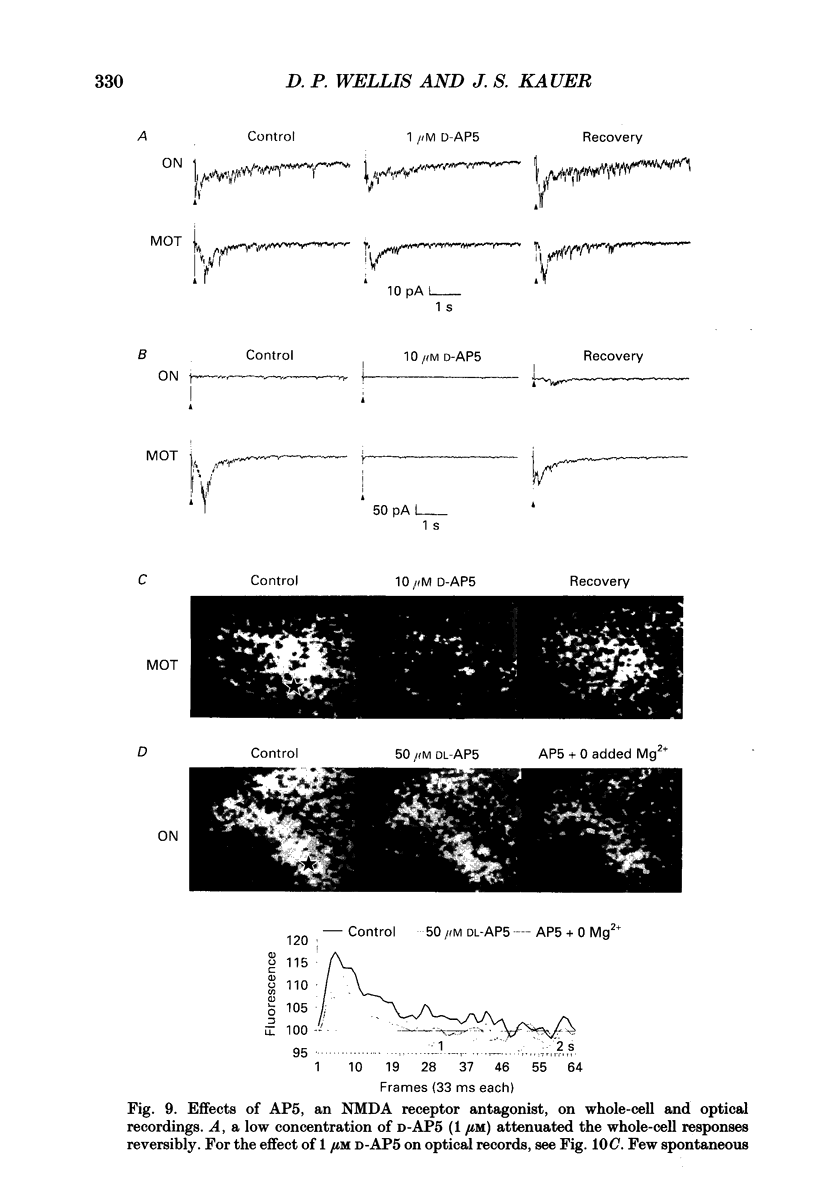
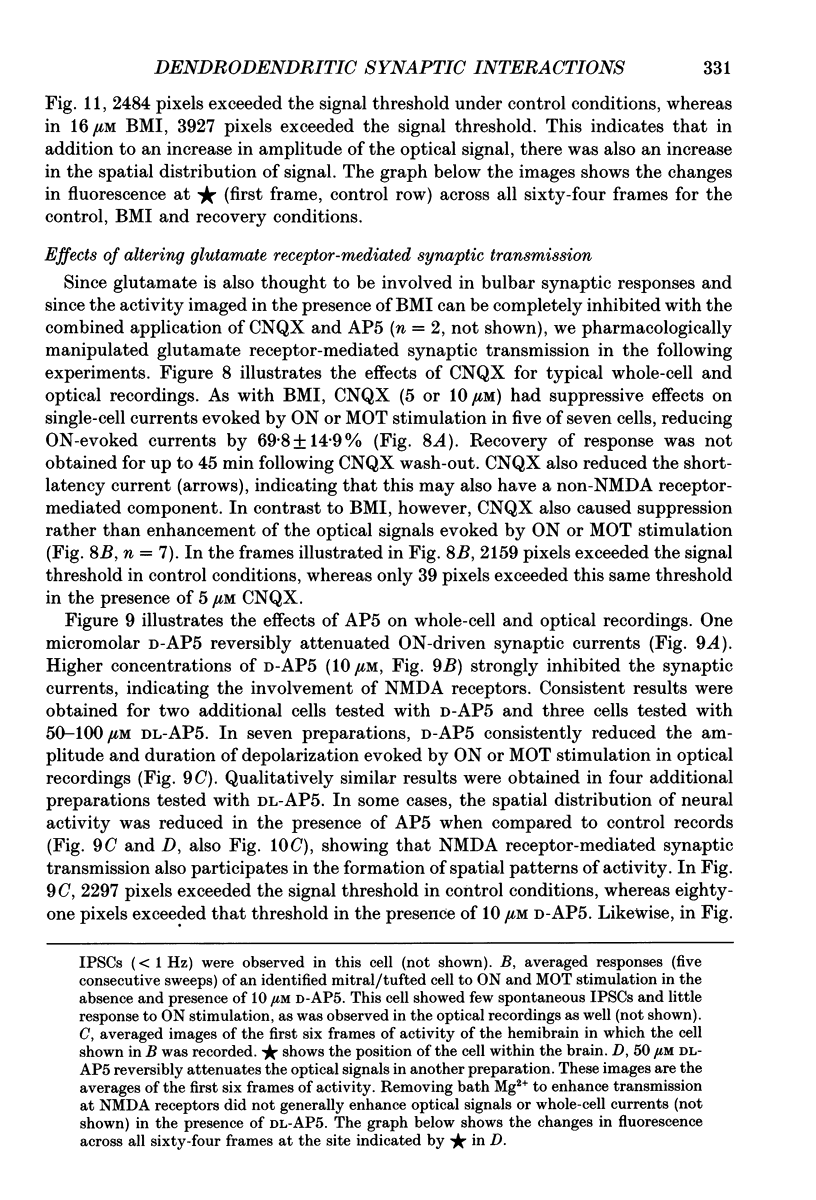
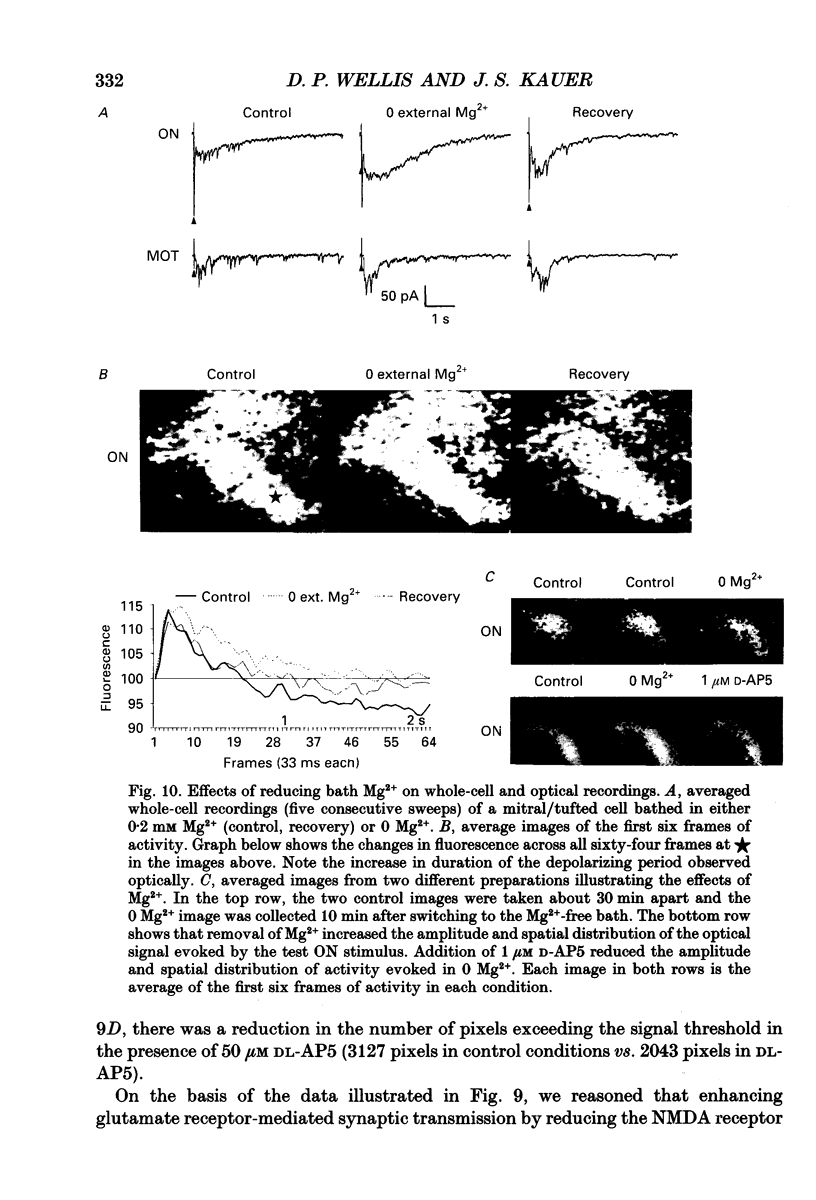
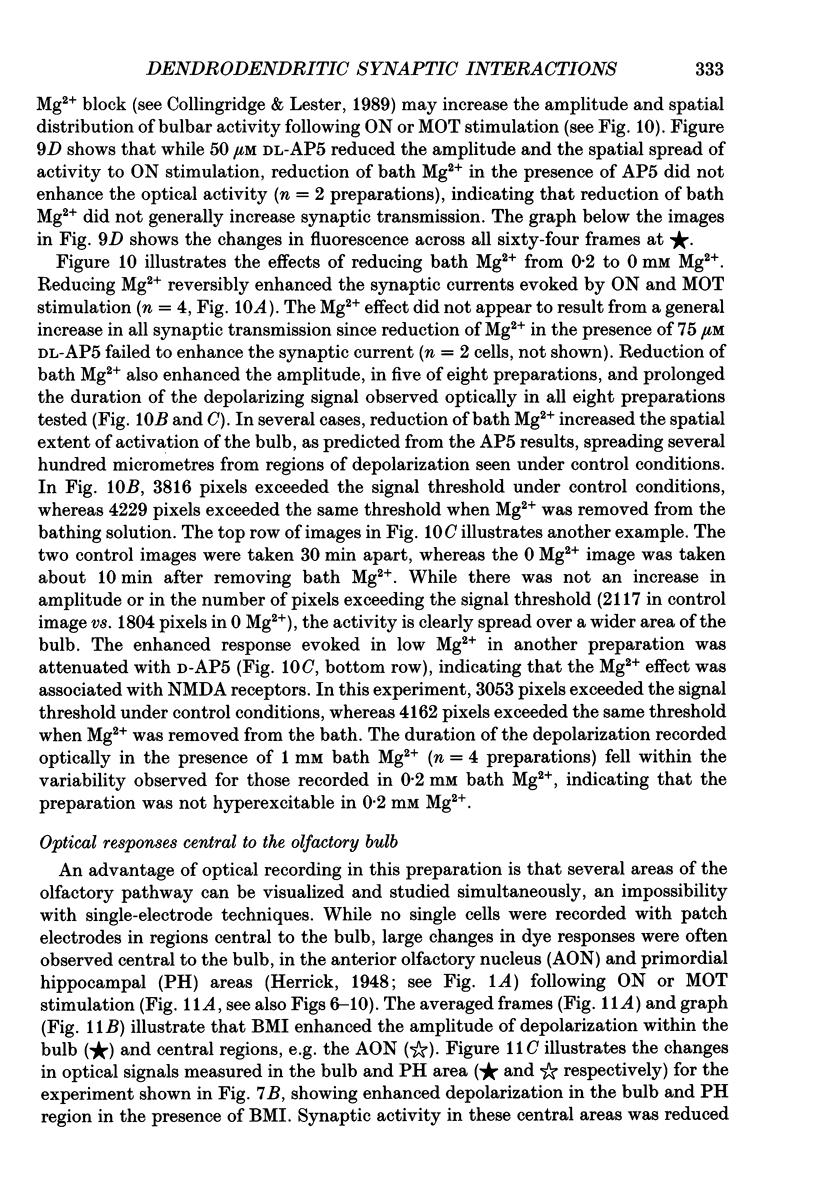
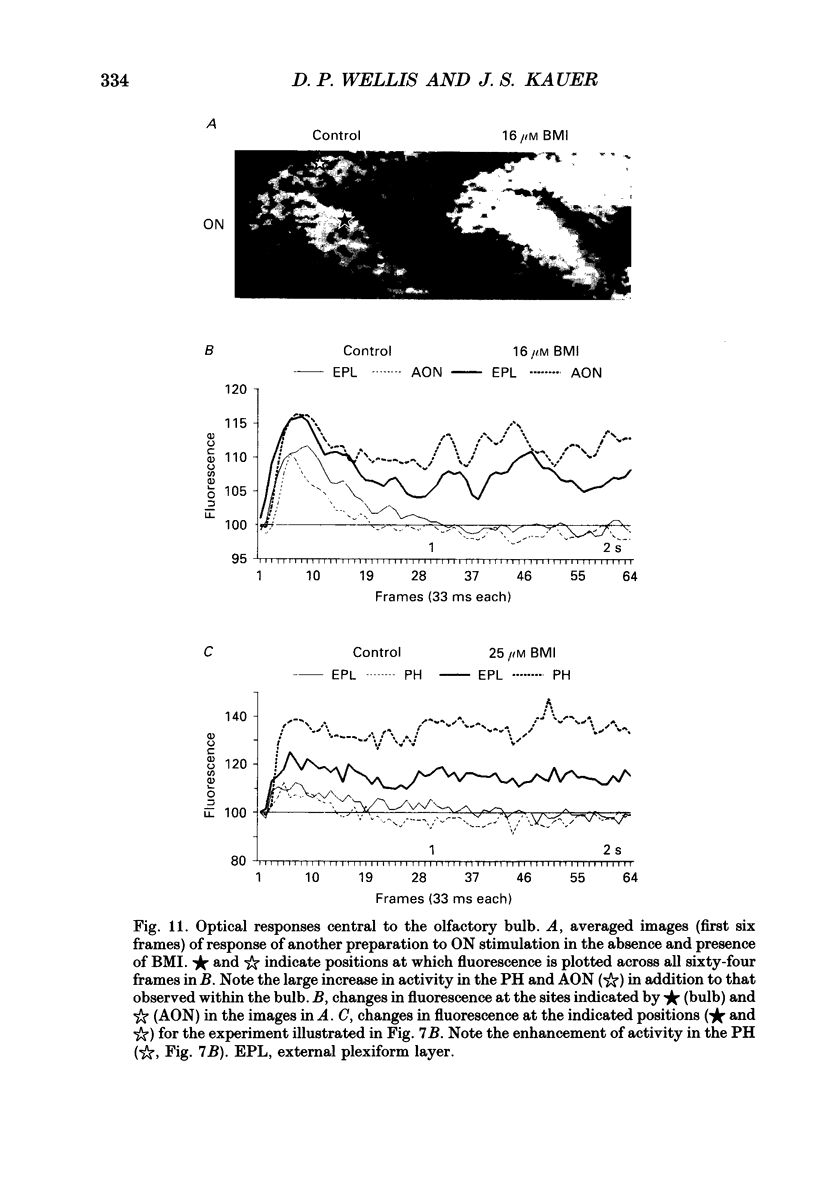
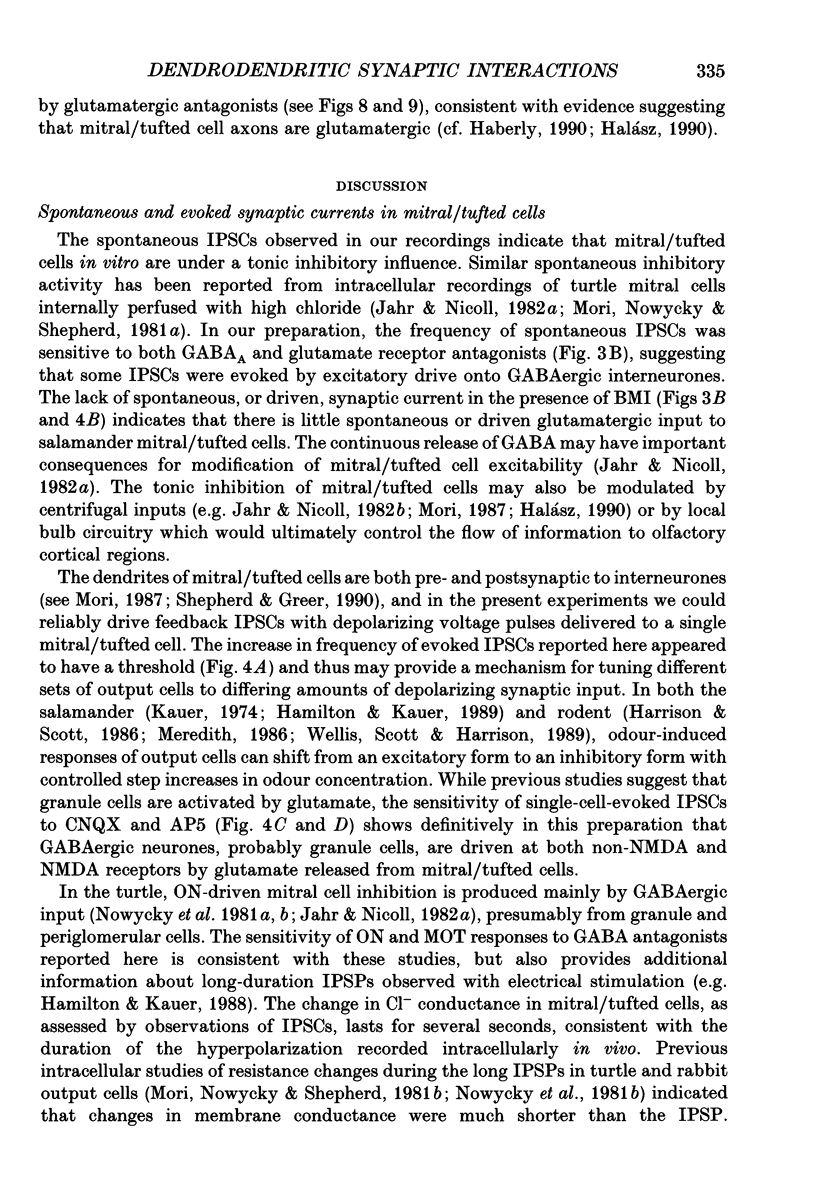
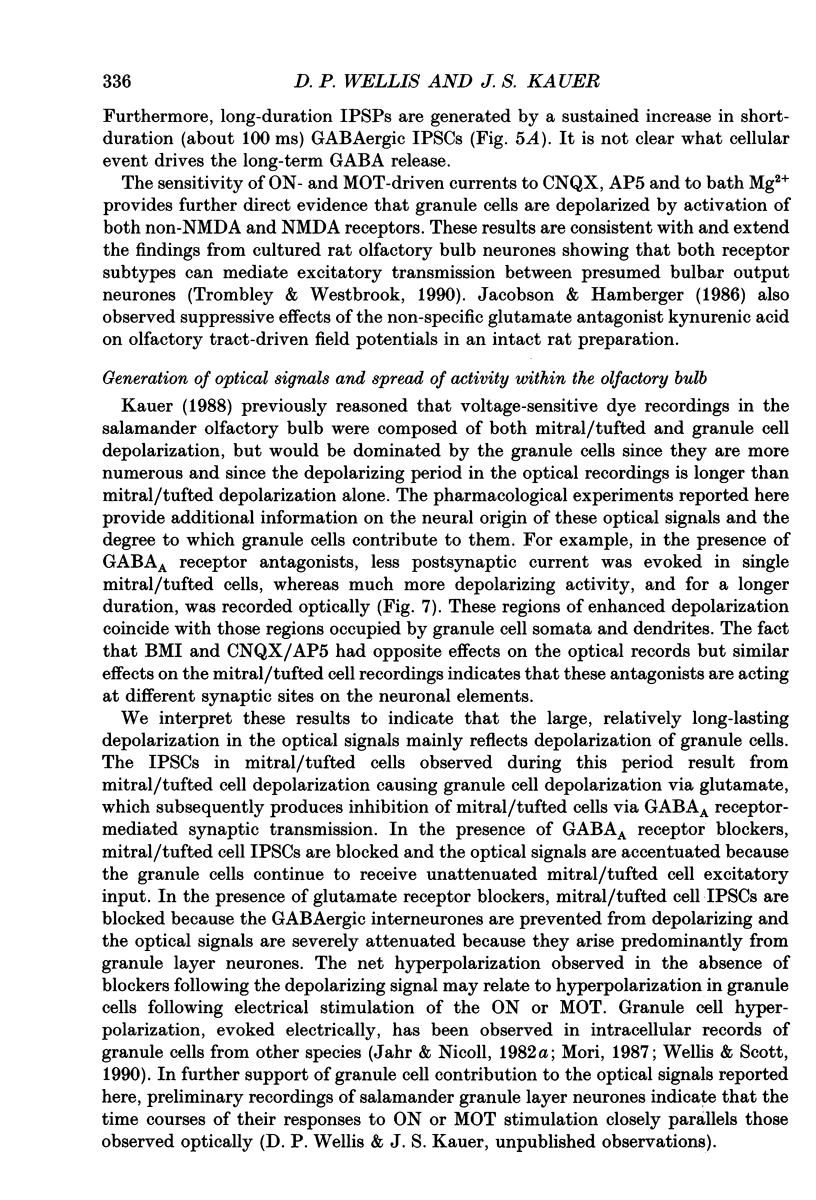
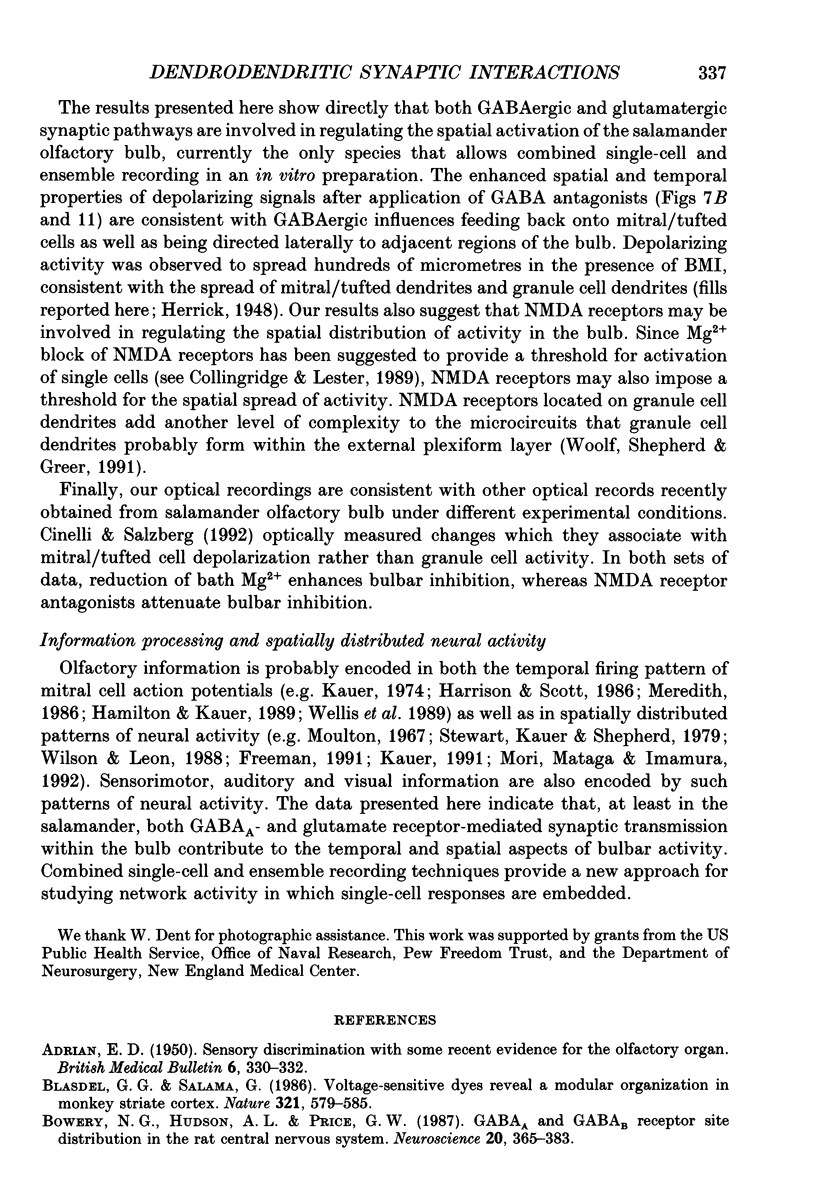
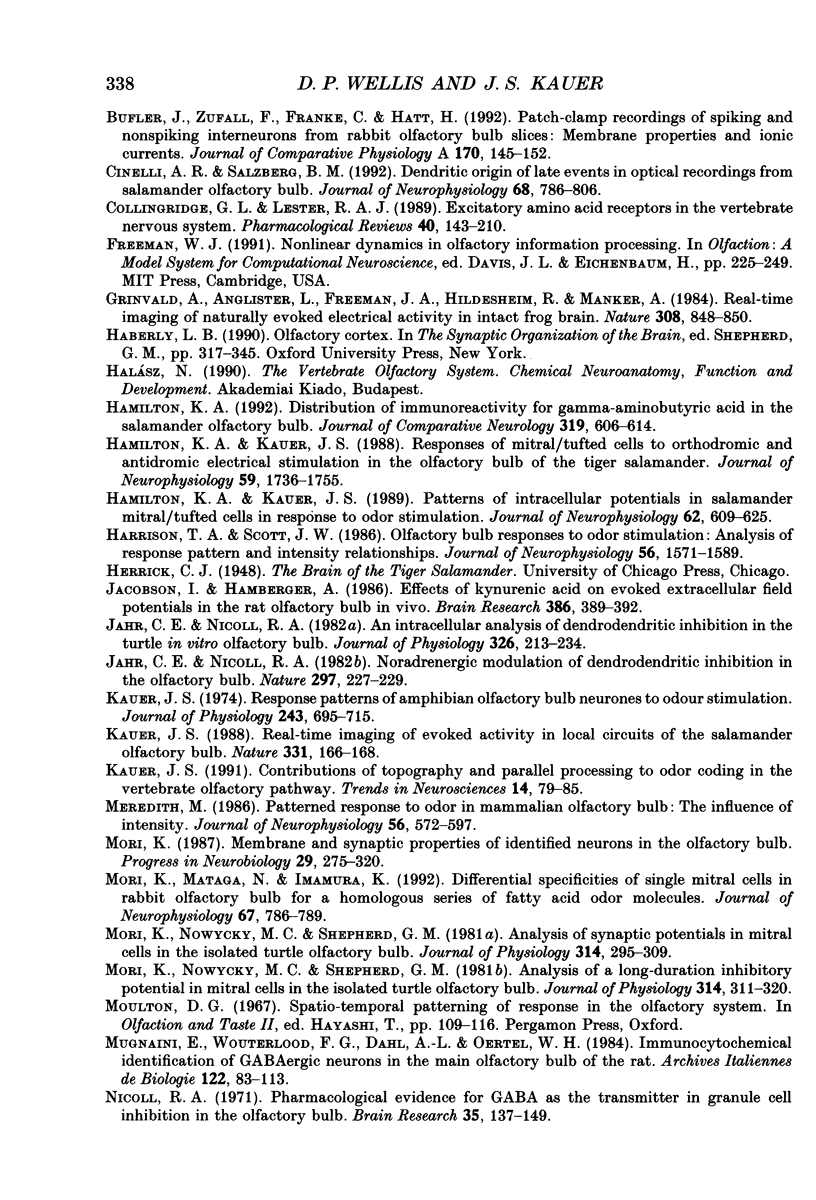
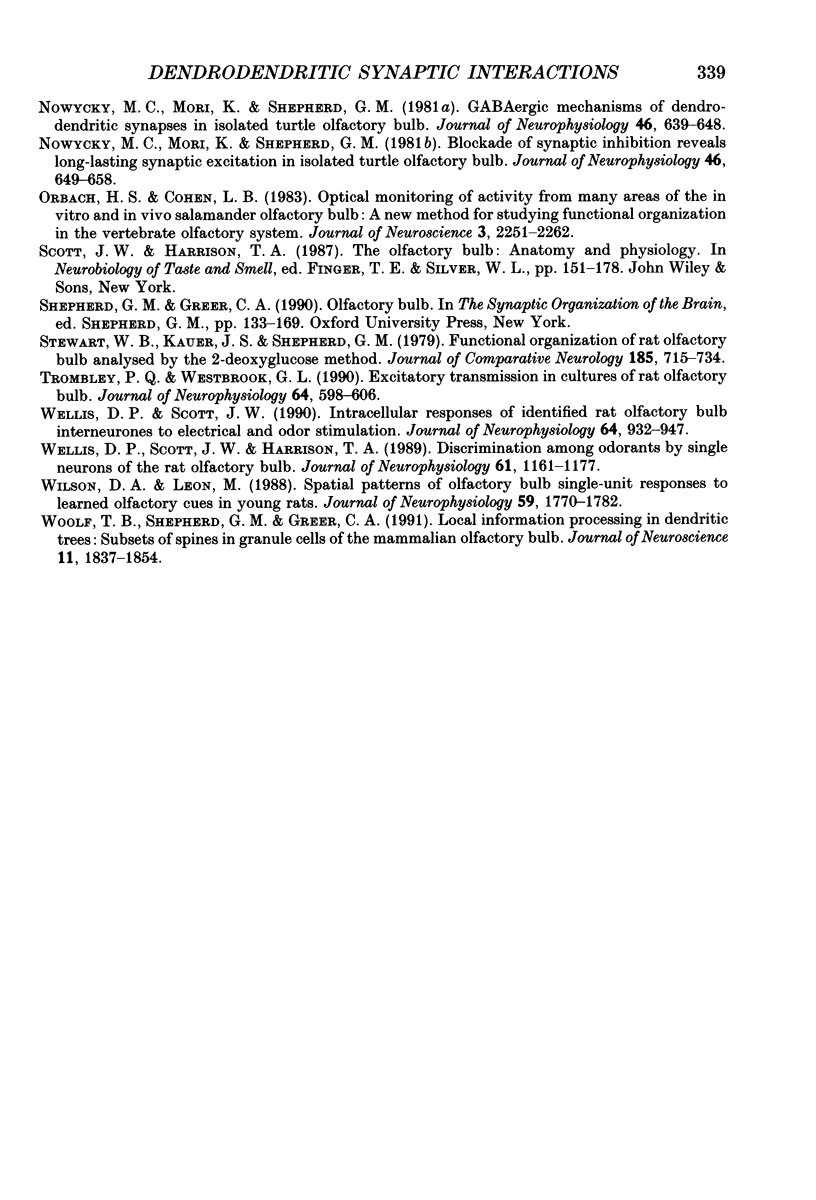
Images in this article
Selected References
These references are in PubMed. This may not be the complete list of references from this article.
- ADRIAN E. D. Sensory discrimination with some recent evidence from the olfactory organ. Br Med Bull. 1950;6(4):330–333. doi: 10.1093/oxfordjournals.bmb.a073625. [DOI] [PubMed] [Google Scholar]
- Blasdel G. G., Salama G. Voltage-sensitive dyes reveal a modular organization in monkey striate cortex. Nature. 1986 Jun 5;321(6070):579–585. doi: 10.1038/321579a0. [DOI] [PubMed] [Google Scholar]
- Bowery N. G., Hudson A. L., Price G. W. GABAA and GABAB receptor site distribution in the rat central nervous system. Neuroscience. 1987 Feb;20(2):365–383. doi: 10.1016/0306-4522(87)90098-4. [DOI] [PubMed] [Google Scholar]
- Bufler J., Zufall F., Franke C., Hatt H. Patch-clamp recordings of spiking and nonspiking interneurons from rabbit olfactory bulb slices: membrane properties and ionic currents. J Comp Physiol A. 1992 Feb;170(2):145–152. doi: 10.1007/BF00196896. [DOI] [PubMed] [Google Scholar]
- Cinelli A. R., Salzberg B. M. Dendritic origin of late events in optical recordings from salamander olfactory bulb. J Neurophysiol. 1992 Sep;68(3):786–806. doi: 10.1152/jn.1992.68.3.786. [DOI] [PubMed] [Google Scholar]
- Collingridge G. L., Lester R. A. Excitatory amino acid receptors in the vertebrate central nervous system. Pharmacol Rev. 1989 Jun;41(2):143–210. [PubMed] [Google Scholar]
- Grinvald A., Anglister L., Freeman J. A., Hildesheim R., Manker A. Real-time optical imaging of naturally evoked electrical activity in intact frog brain. 1984 Apr 26-May 2Nature. 308(5962):848–850. doi: 10.1038/308848a0. [DOI] [PubMed] [Google Scholar]
- Hamilton K. A. Distribution of immunoreactivity for gamma-aminobutyric acid in the salamander olfactory bulb. J Comp Neurol. 1992 May 22;319(4):606–614. doi: 10.1002/cne.903190410. [DOI] [PubMed] [Google Scholar]
- Hamilton K. A., Kauer J. S. Patterns of intracellular potentials in salamander mitral/tufted cells in response to odor stimulation. J Neurophysiol. 1989 Sep;62(3):609–625. doi: 10.1152/jn.1989.62.3.609. [DOI] [PubMed] [Google Scholar]
- Hamilton K. A., Kauer J. S. Responses of mitral/tufted cells to orthodromic and antidromic electrical stimulation in the olfactory bulb of the tiger salamander. J Neurophysiol. 1988 Jun;59(6):1736–1755. doi: 10.1152/jn.1988.59.6.1736. [DOI] [PubMed] [Google Scholar]
- Harrison T. A., Scott J. W. Olfactory bulb responses to odor stimulation: analysis of response pattern and intensity relationships. J Neurophysiol. 1986 Dec;56(6):1571–1589. doi: 10.1152/jn.1986.56.6.1571. [DOI] [PubMed] [Google Scholar]
- Jacobson I., Hamberger A. Effects of kynurenic acid on evoked extracellular field potentials in the rat olfactory bulb in vivo. Brain Res. 1986 Oct 29;386(1-2):389–392. doi: 10.1016/0006-8993(86)90177-0. [DOI] [PubMed] [Google Scholar]
- Jahr C. E., Nicoll R. A. An intracellular analysis of dendrodendritic inhibition in the turtle in vitro olfactory bulb. J Physiol. 1982 May;326:213–234. doi: 10.1113/jphysiol.1982.sp014187. [DOI] [PMC free article] [PubMed] [Google Scholar]
- Jahr C. E., Nicoll R. A. Noradrenergic modulation of dendrodendritic inhibition in the olfactory bulb. Nature. 1982 May 20;297(5863):227–229. doi: 10.1038/297227a0. [DOI] [PubMed] [Google Scholar]
- Kauer J. S. Contributions of topography and parallel processing to odor coding in the vertebrate olfactory pathway. Trends Neurosci. 1991 Feb;14(2):79–85. doi: 10.1016/0166-2236(91)90025-p. [DOI] [PubMed] [Google Scholar]
- Kauer J. S. Real-time imaging of evoked activity in local circuits of the salamander olfactory bulb. Nature. 1988 Jan 14;331(6152):166–168. doi: 10.1038/331166a0. [DOI] [PubMed] [Google Scholar]
- Kauer J. S. Response patterns of amphibian olfactory bulb neurones to odour stimulation. J Physiol. 1974 Dec;243(3):695–715. doi: 10.1113/jphysiol.1974.sp010772. [DOI] [PMC free article] [PubMed] [Google Scholar]
- Meredith M. Patterned response to odor in mammalian olfactory bulb: the influence of intensity. J Neurophysiol. 1986 Sep;56(3):572–597. doi: 10.1152/jn.1986.56.3.572. [DOI] [PubMed] [Google Scholar]
- Mori K., Mataga N., Imamura K. Differential specificities of single mitral cells in rabbit olfactory bulb for a homologous series of fatty acid odor molecules. J Neurophysiol. 1992 Mar;67(3):786–789. doi: 10.1152/jn.1992.67.3.786. [DOI] [PubMed] [Google Scholar]
- Mori K. Membrane and synaptic properties of identified neurons in the olfactory bulb. Prog Neurobiol. 1987;29(3):275–320. doi: 10.1016/0301-0082(87)90024-4. [DOI] [PubMed] [Google Scholar]
- Mori K., Nowycky M. C., Shepherd G. M. Analysis of a long-duration inhibitory potential in mitral cells in the isolated turtle olfactory bulb. J Physiol. 1981 May;314:311–320. doi: 10.1113/jphysiol.1981.sp013709. [DOI] [PMC free article] [PubMed] [Google Scholar]
- Mori K., Nowycky M. C., Shepherd G. M. Analysis of synaptic potentials in mitral cells in the isolated turtle olfactory bulb. J Physiol. 1981 May;314:295–309. doi: 10.1113/jphysiol.1981.sp013708. [DOI] [PMC free article] [PubMed] [Google Scholar]
- Mugnaini E., Wouterlood F. G., Dahl A. L., Oertel W. H. Immunocytochemical identification of GABAergic neurons in the main olfactory bulb of the rat. Arch Ital Biol. 1984 Jun;122(2):83–113. [PubMed] [Google Scholar]
- Nicoll R. A. Pharmacological evidence for GABA as the transmitter in granule cell inhibition in the olfactory bulb. Brain Res. 1971 Dec 10;35(1):137–149. doi: 10.1016/0006-8993(71)90600-7. [DOI] [PubMed] [Google Scholar]
- Nowycky M. C., Mori K., Shepherd G. M. Blockade of synaptic inhibition reveals long-lasting synaptic excitation in isolated turtle olfactory bulb. J Neurophysiol. 1981 Sep;46(3):649–658. doi: 10.1152/jn.1981.46.3.649. [DOI] [PubMed] [Google Scholar]
- Nowycky M. C., Mori K., Shepherd G. M. GABAergic mechanisms of dendrodendritic synapses in isolated turtle olfactory bulb. J Neurophysiol. 1981 Sep;46(3):639–648. doi: 10.1152/jn.1981.46.3.639. [DOI] [PubMed] [Google Scholar]
- Orbach H. S., Cohen L. B. Optical monitoring of activity from many areas of the in vitro and in vivo salamander olfactory bulb: a new method for studying functional organization in the vertebrate central nervous system. J Neurosci. 1983 Nov;3(11):2251–2262. doi: 10.1523/JNEUROSCI.03-11-02251.1983. [DOI] [PMC free article] [PubMed] [Google Scholar]
- Stewart W. B., Kauer J. S., Shepherd G. M. Functional organization of rat olfactory bulb analysed by the 2-deoxyglucose method. J Comp Neurol. 1979 Jun 15;185(4):715–734. doi: 10.1002/cne.901850407. [DOI] [PubMed] [Google Scholar]
- Trombley P. Q., Westbrook G. L. Excitatory synaptic transmission in cultures of rat olfactory bulb. J Neurophysiol. 1990 Aug;64(2):598–606. doi: 10.1152/jn.1990.64.2.598. [DOI] [PubMed] [Google Scholar]
- Wellis D. P., Scott J. W., Harrison T. A. Discrimination among odorants by single neurons of the rat olfactory bulb. J Neurophysiol. 1989 Jun;61(6):1161–1177. doi: 10.1152/jn.1989.61.6.1161. [DOI] [PubMed] [Google Scholar]
- Wellis D. P., Scott J. W. Intracellular responses of identified rat olfactory bulb interneurons to electrical and odor stimulation. J Neurophysiol. 1990 Sep;64(3):932–947. doi: 10.1152/jn.1990.64.3.932. [DOI] [PubMed] [Google Scholar]
- Wilson D. A., Leon M. Spatial patterns of olfactory bulb single-unit responses to learned olfactory cues in young rats. J Neurophysiol. 1988 Jun;59(6):1770–1782. doi: 10.1152/jn.1988.59.6.1770. [DOI] [PubMed] [Google Scholar]
- Woolf T. B., Shepherd G. M., Greer C. A. Local information processing in dendritic trees: subsets of spines in granule cells of the mammalian olfactory bulb. J Neurosci. 1991 Jun;11(6):1837–1854. doi: 10.1523/JNEUROSCI.11-06-01837.1991. [DOI] [PMC free article] [PubMed] [Google Scholar]