Abstract
1. The electrophysiological properties of neurones of the reticular thalamic (RE) nucleus were studied in acutely prepared cats under urethane anaesthesia. 2. Two main types of neuronal firing were recorded. At the resting membrane potential (-60 to -65 mV) tonic repetitive firing was elicited when the cell was activated synaptically or by current injection. From membrane potentials more negative than -75 mV, synaptic or direct stimulation generated a burst of action potentials. 3. The burst of RE cells consisted of a discharge of four to eight spikes riding on a slowly growing and decaying depolarization. The discharge rate during the burst showed a characteristic increase, followed by a decrease in frequency. 4. The burst response behaved as a graded phenomenon, as its magnitude was modulated by changing the intensity of the synaptic volley or the intensity of the injected current. 5. Spike-like small potentials presumably of dendritic origin occurred spontaneously and were triggered by synaptic or direct stimulation. They were all-or-none, voltage-dependent events. We postulate that these spikes originate in several hot spots in the dendritic arbor, with no reciprocal refractoriness and may generate multi-component depolarizations at the somatic level. 6. Excitatory postsynaptic potentials (EPSPs) evoked by internal capsule stimulation consisted of two components, the late one being blocked by hyperpolarization. Such compound EPSPs were followed by a period of decreased excitability during which a second response was diminished in amplitude. 7. A series of depolarizing waves at the frequency range of spindle oscillations was triggered by internal capsule stimulation. The individual depolarizing waves constituting the spindle oscillation gradually decreased in amplitude when decreasing the intensity of the stimulation. 8. These results, showing that RE cells are endowed with an excitable dendritic tree and a graded bursting behaviour, support the proposed role of RE nucleus as the generator and synchronizer of spindle rhythmicity.
Full text
PDF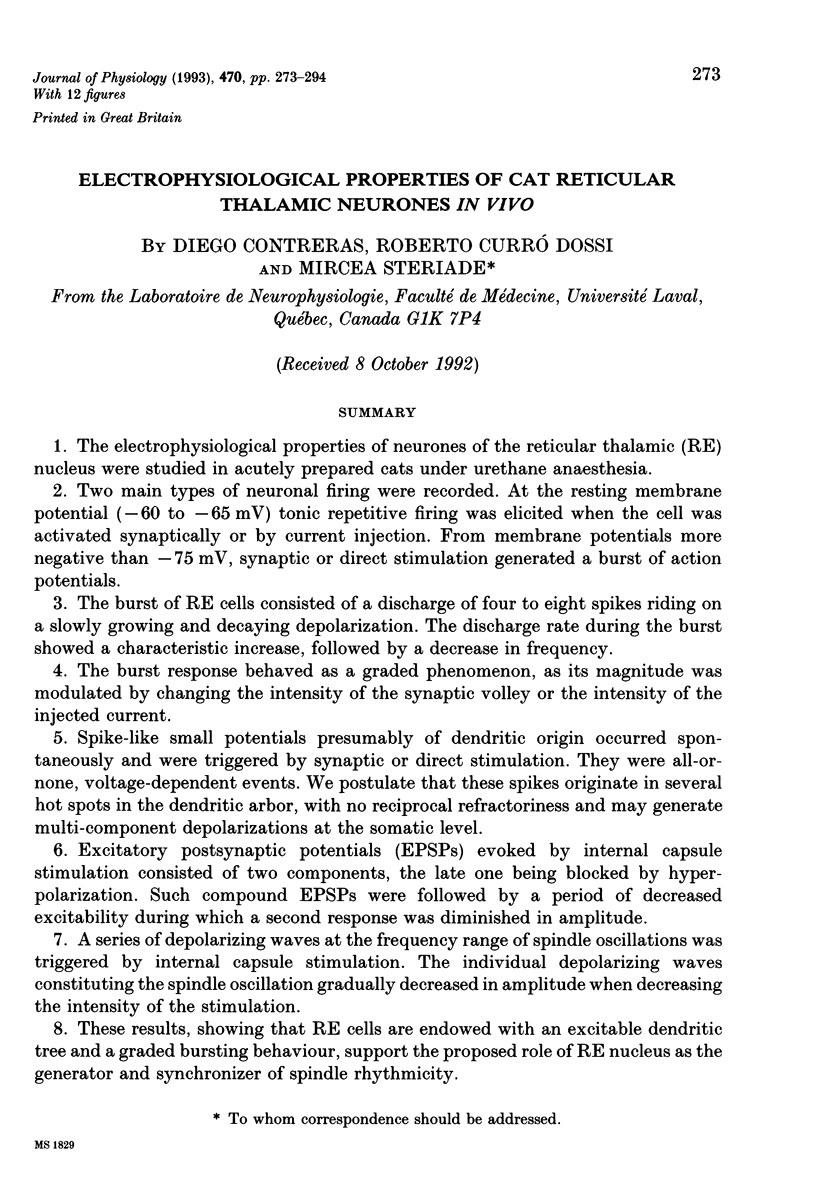
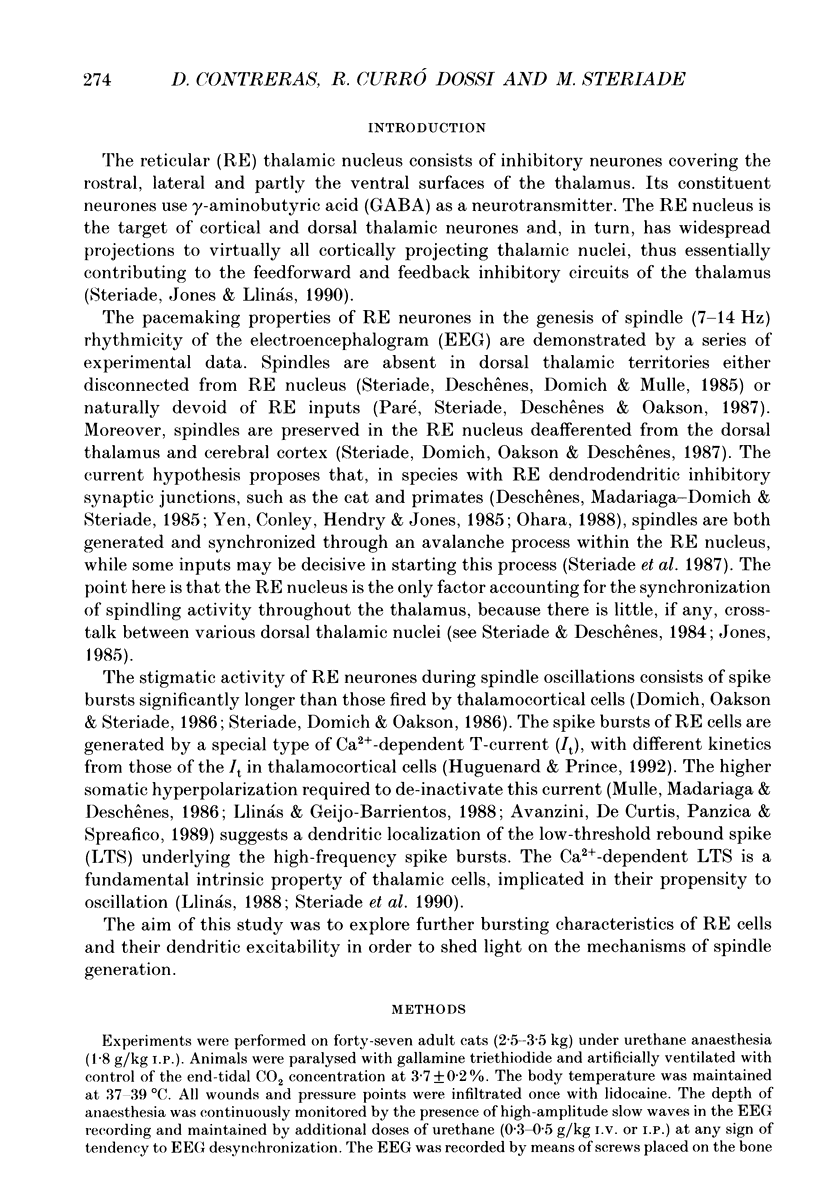
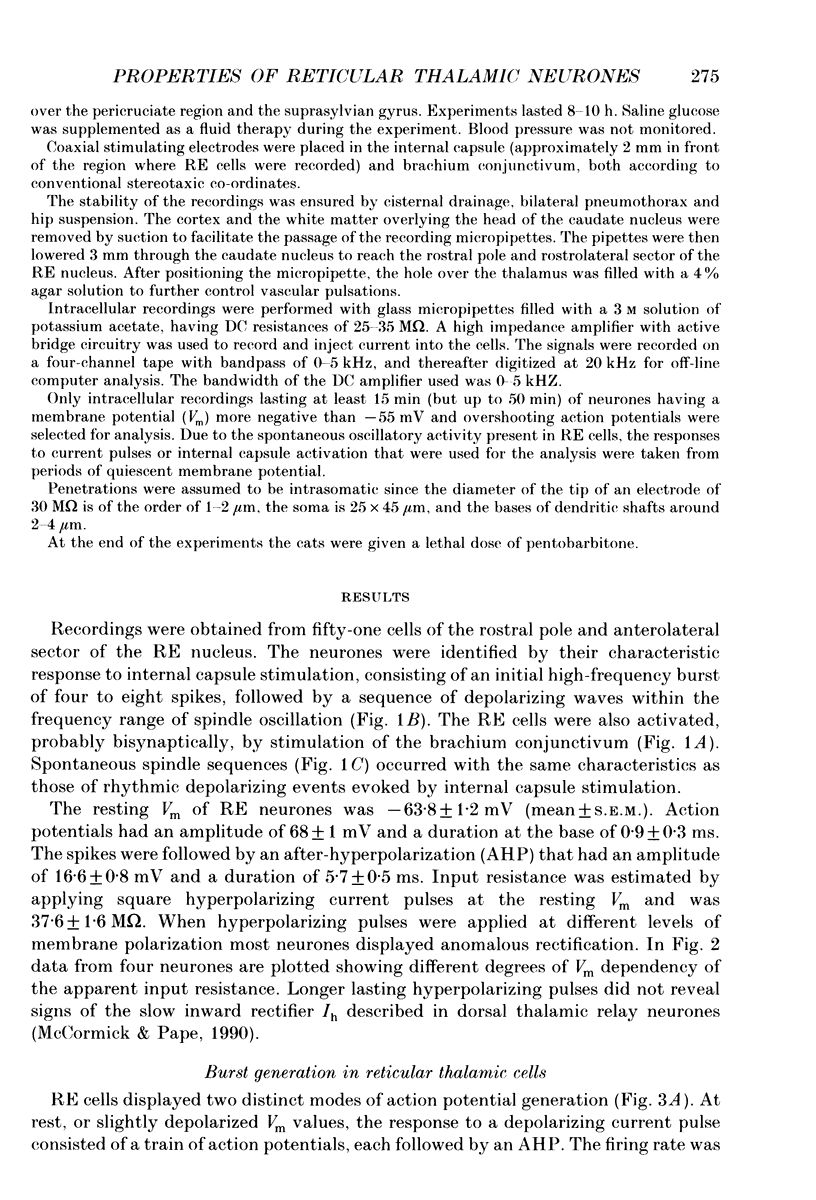
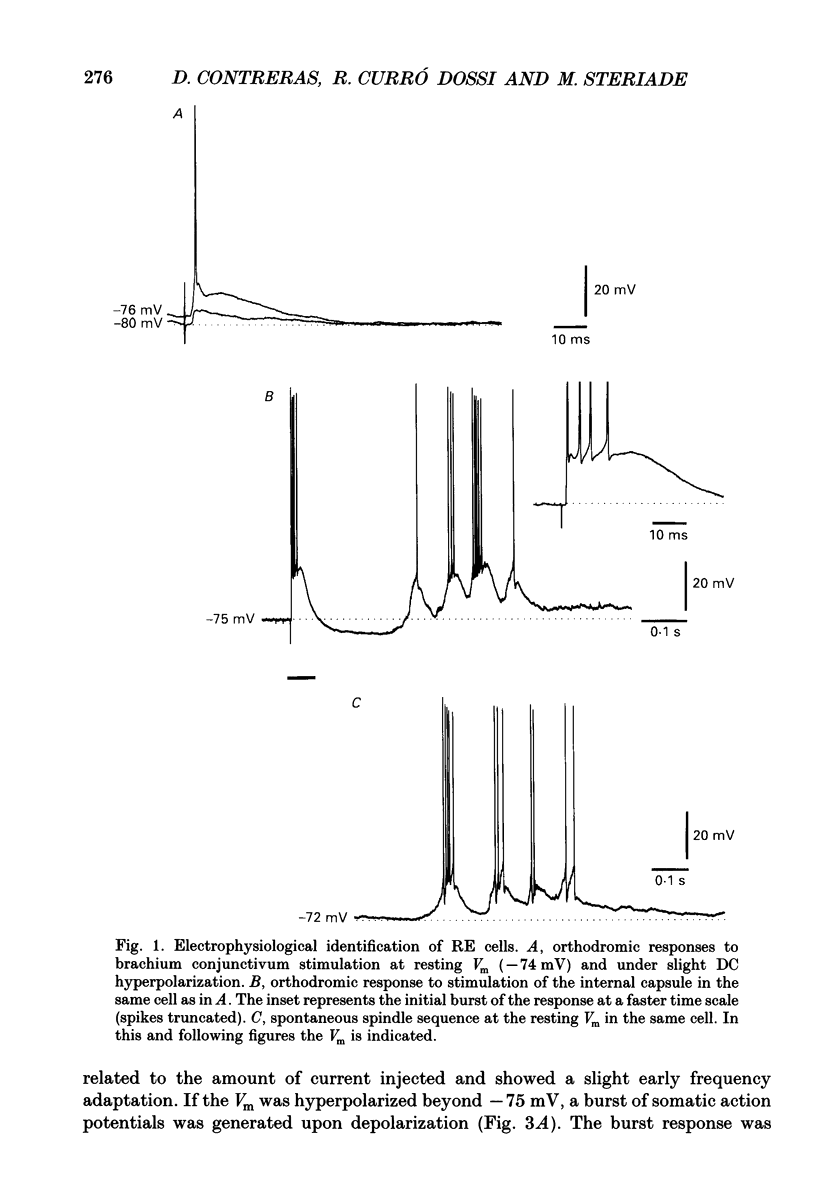
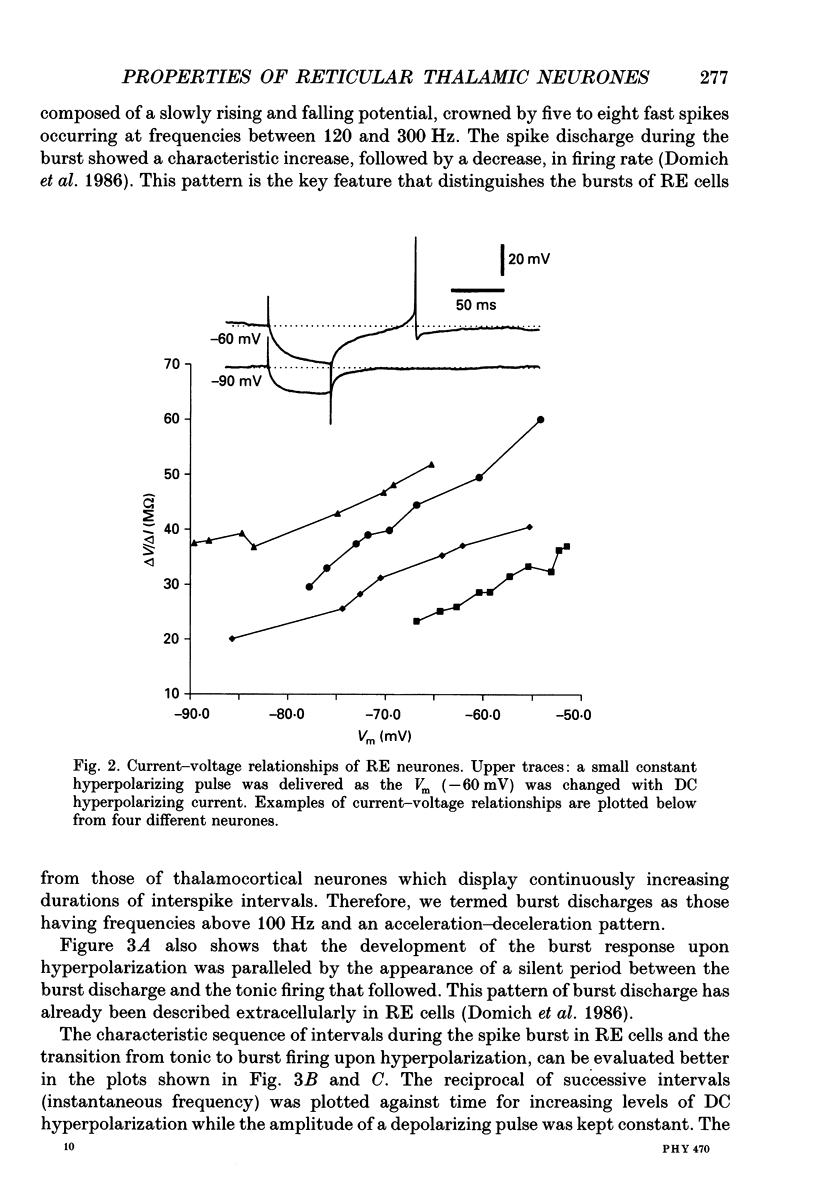
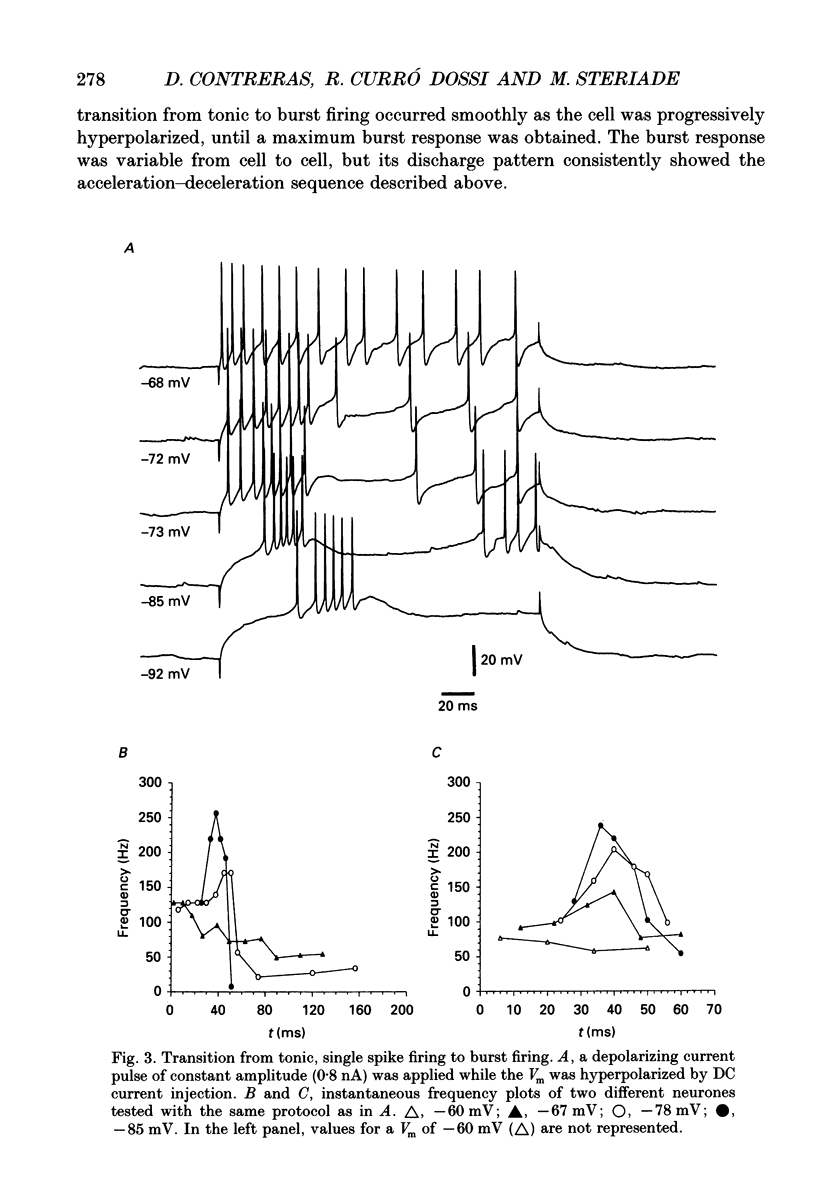
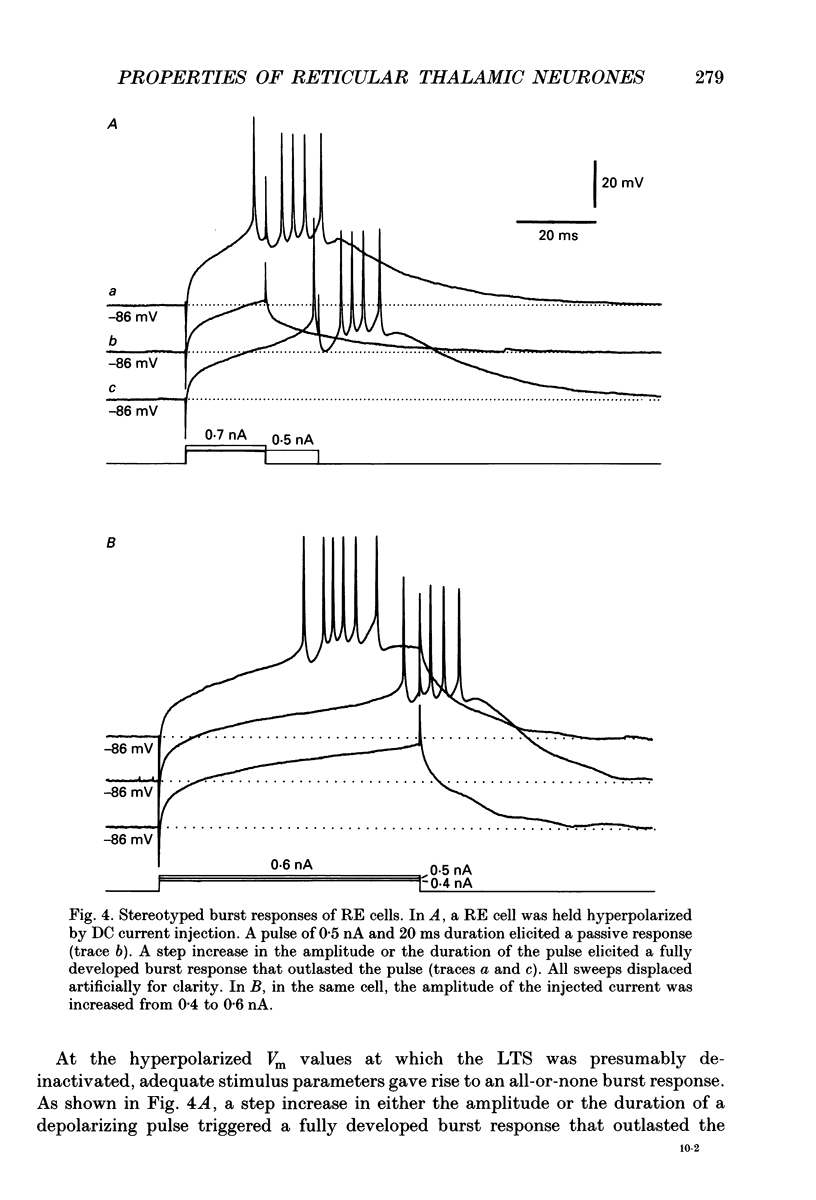
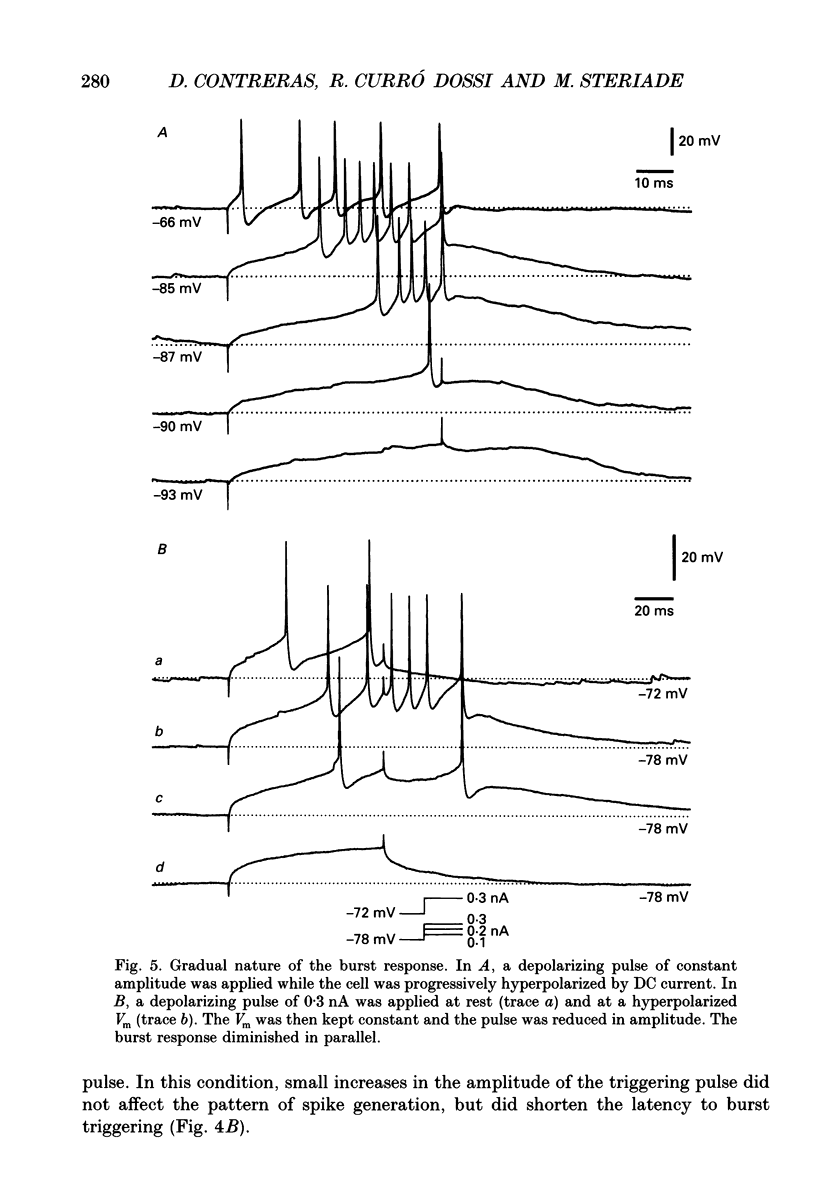
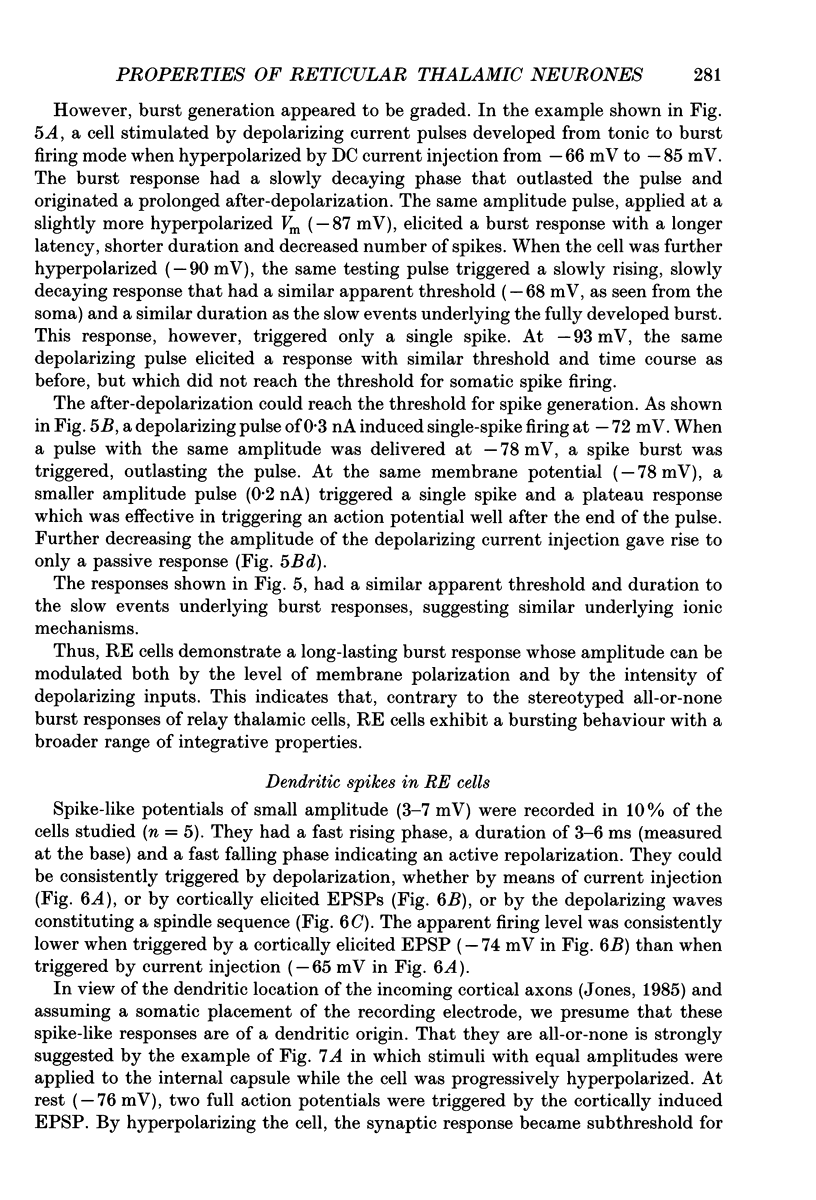
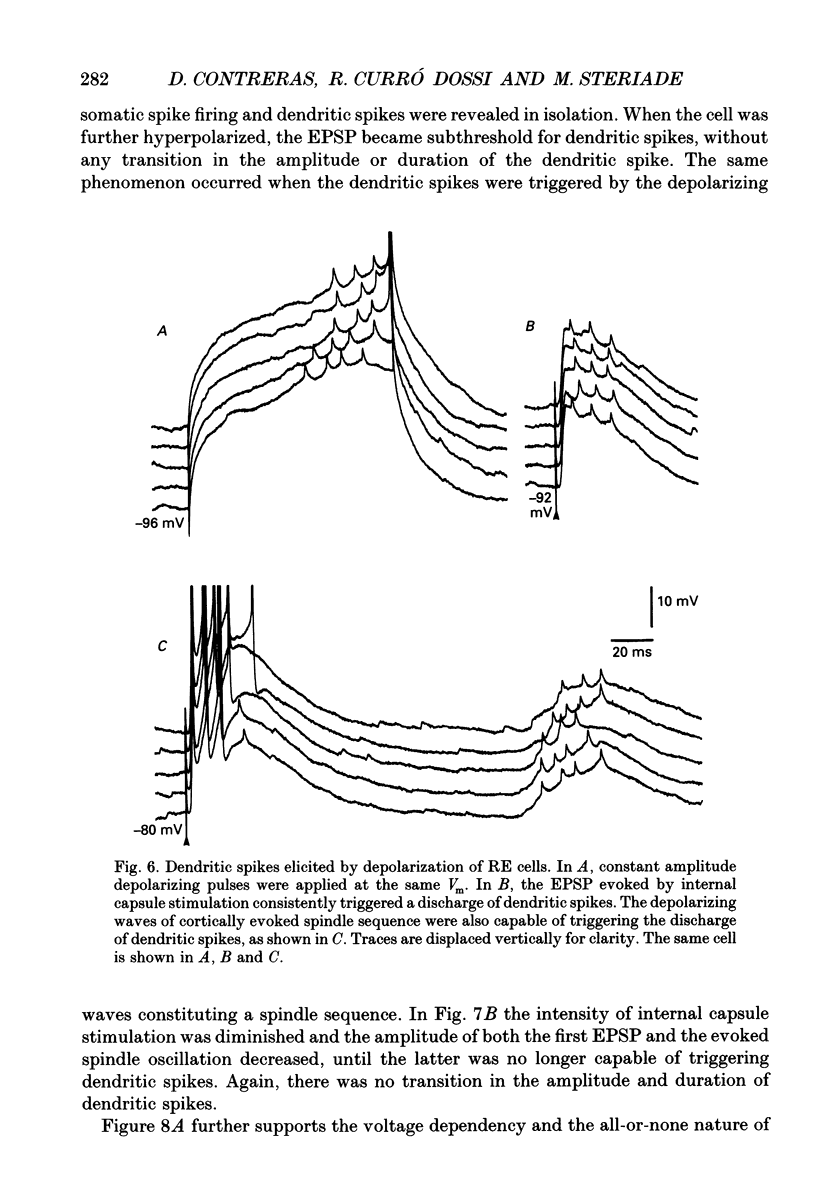
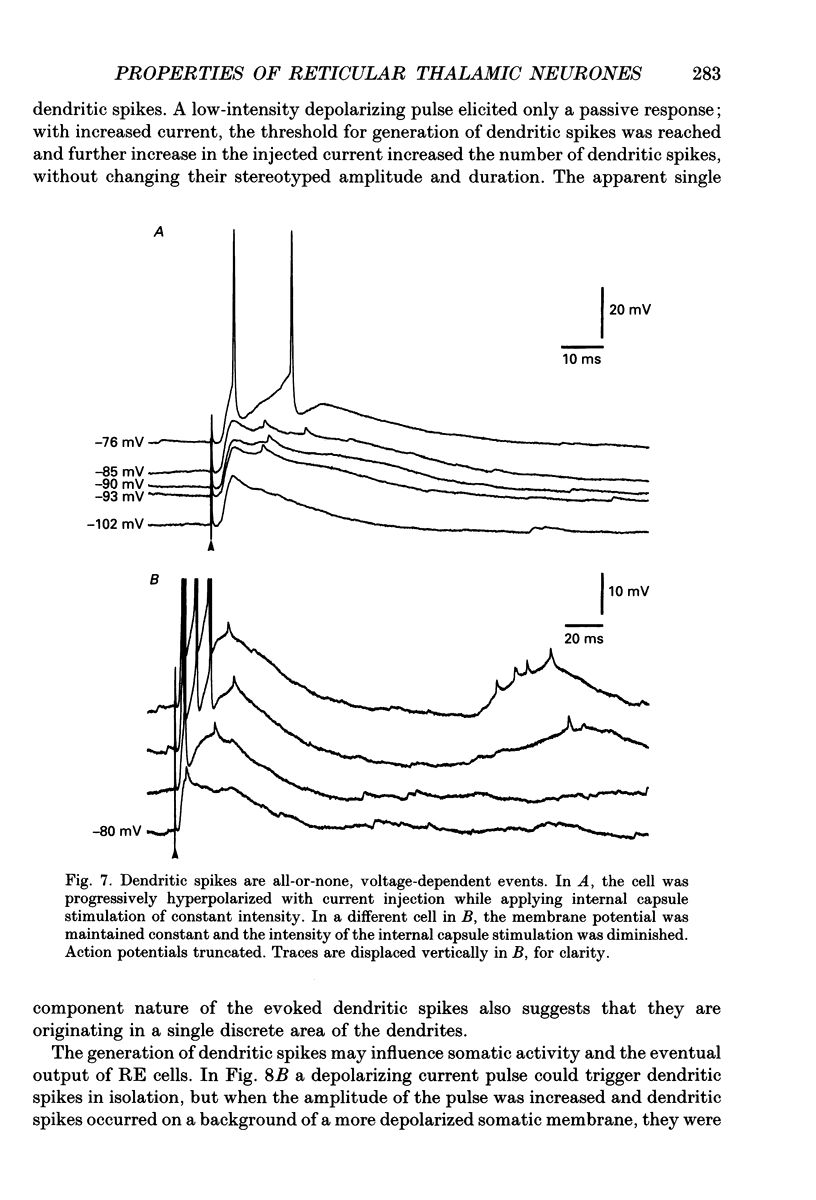
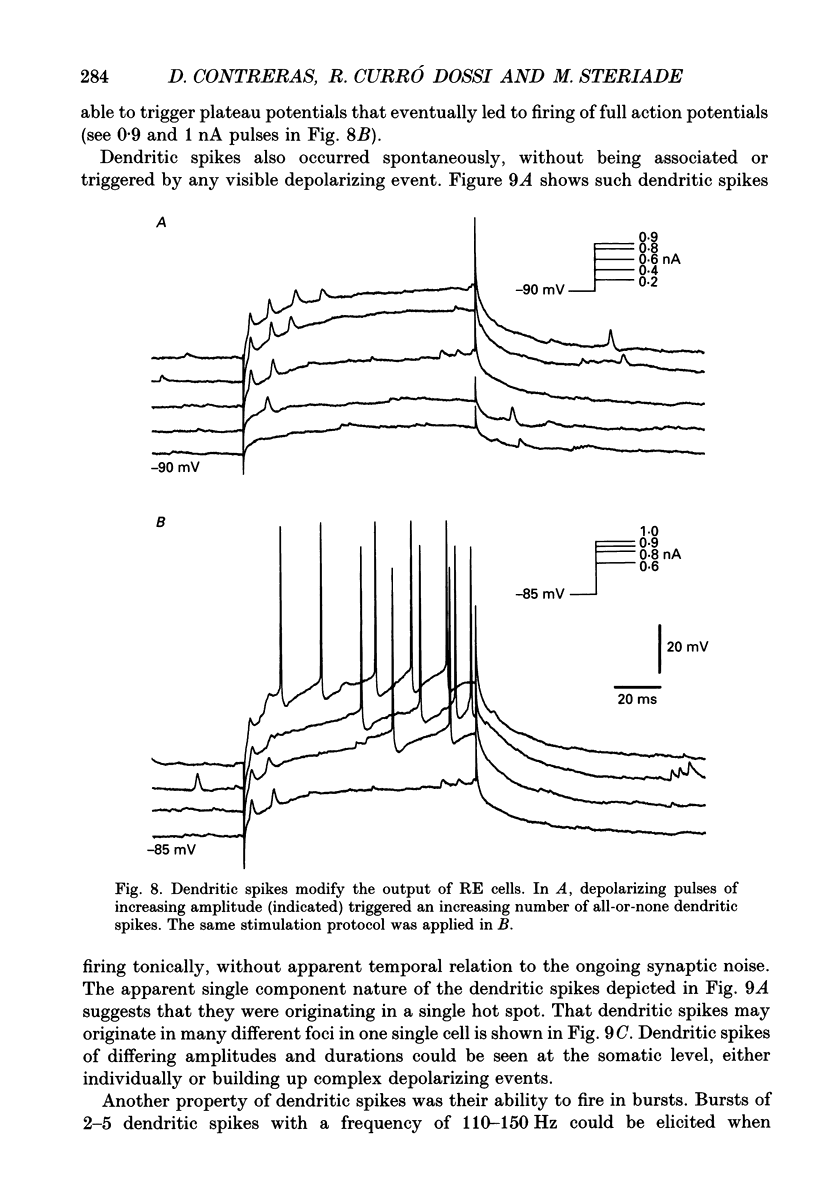
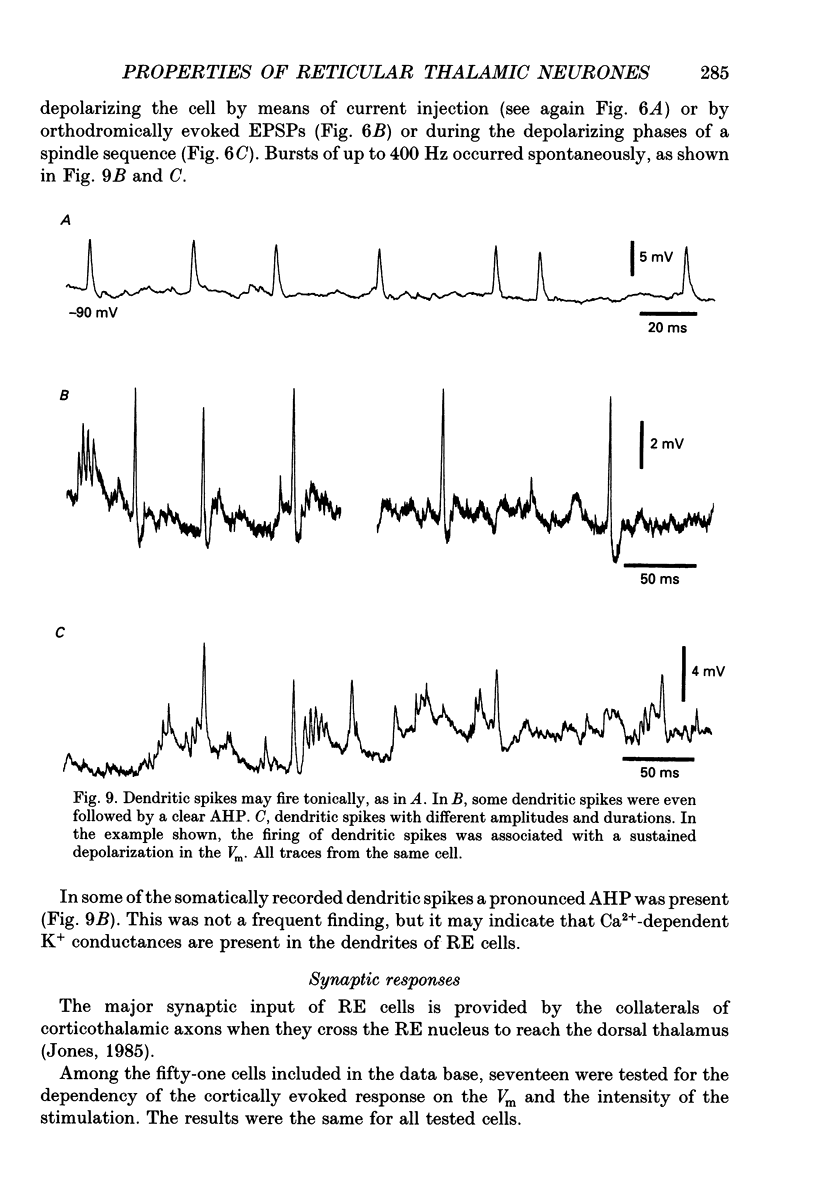
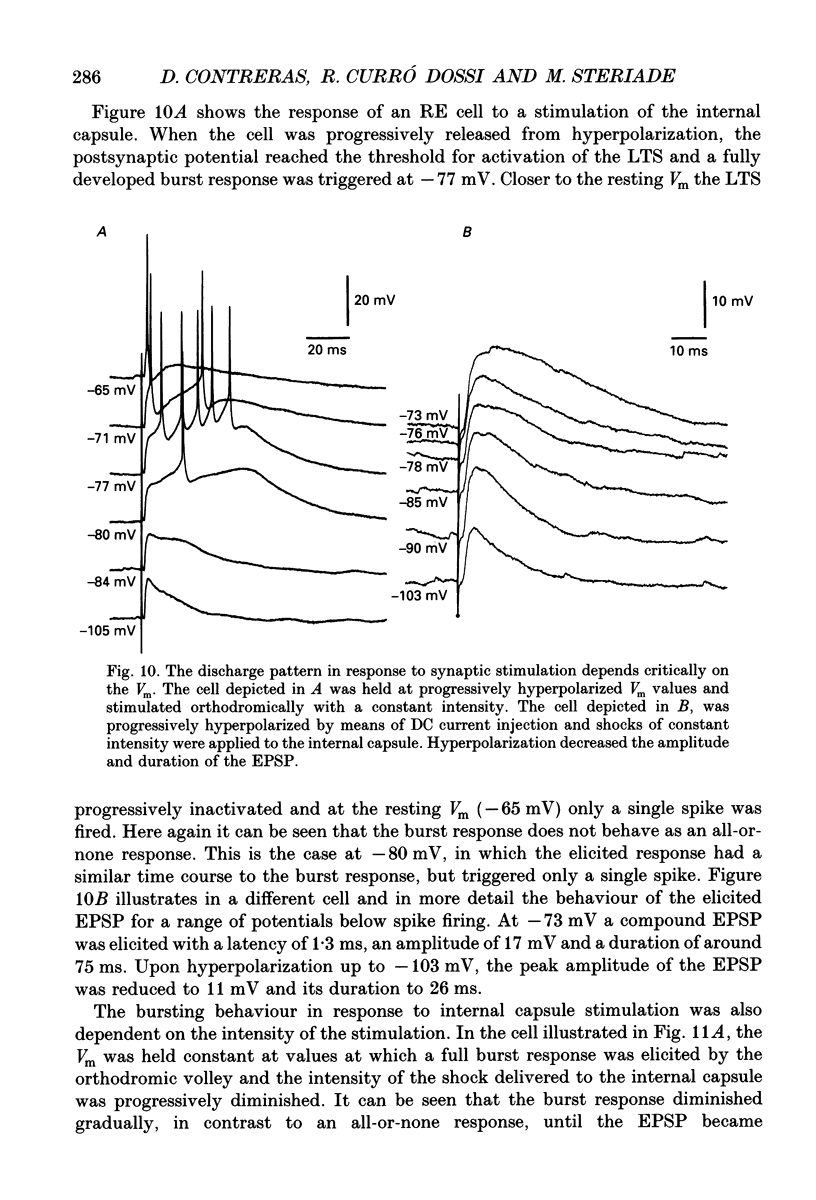
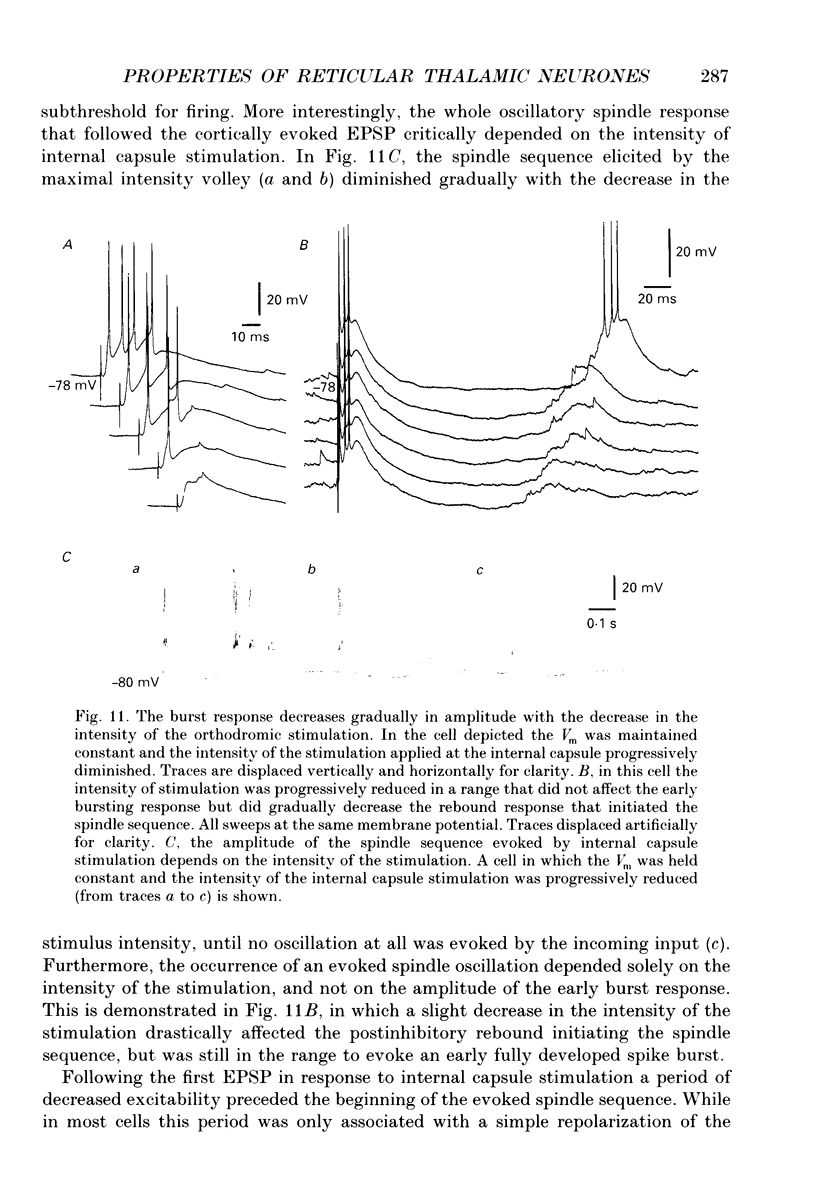
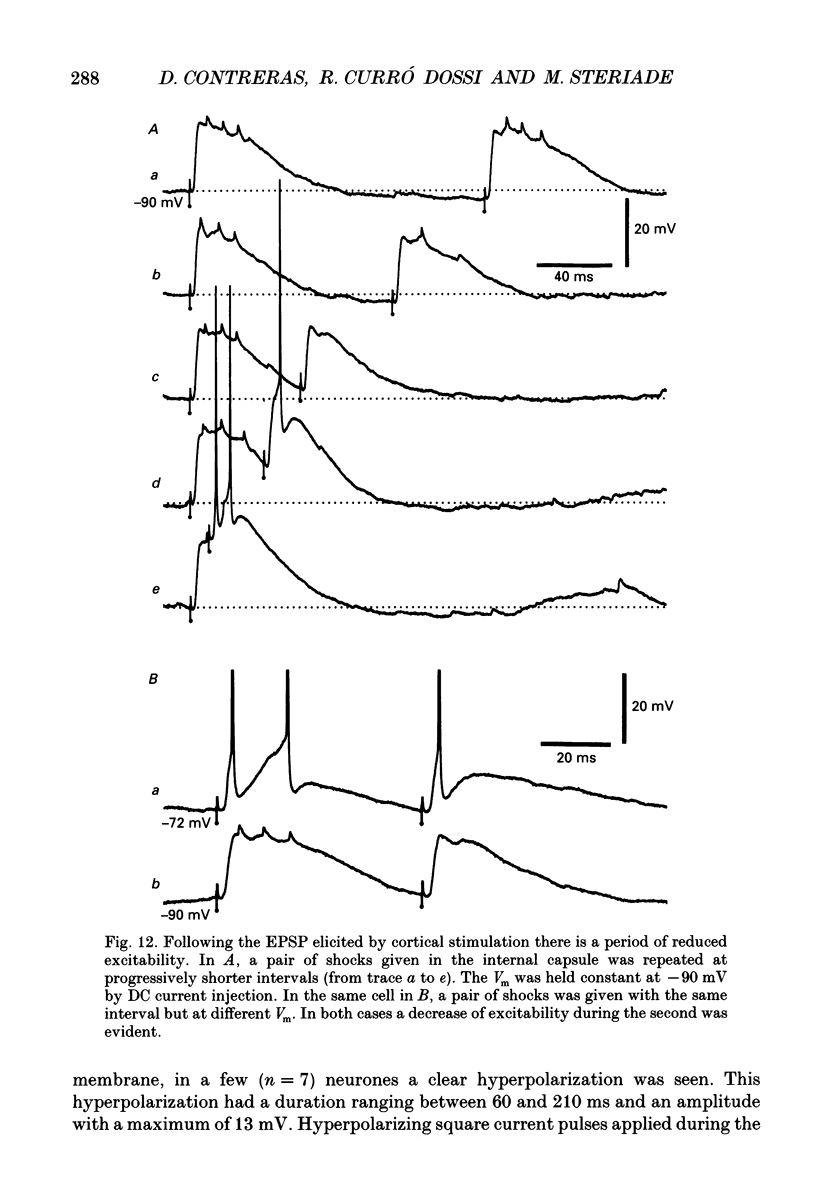
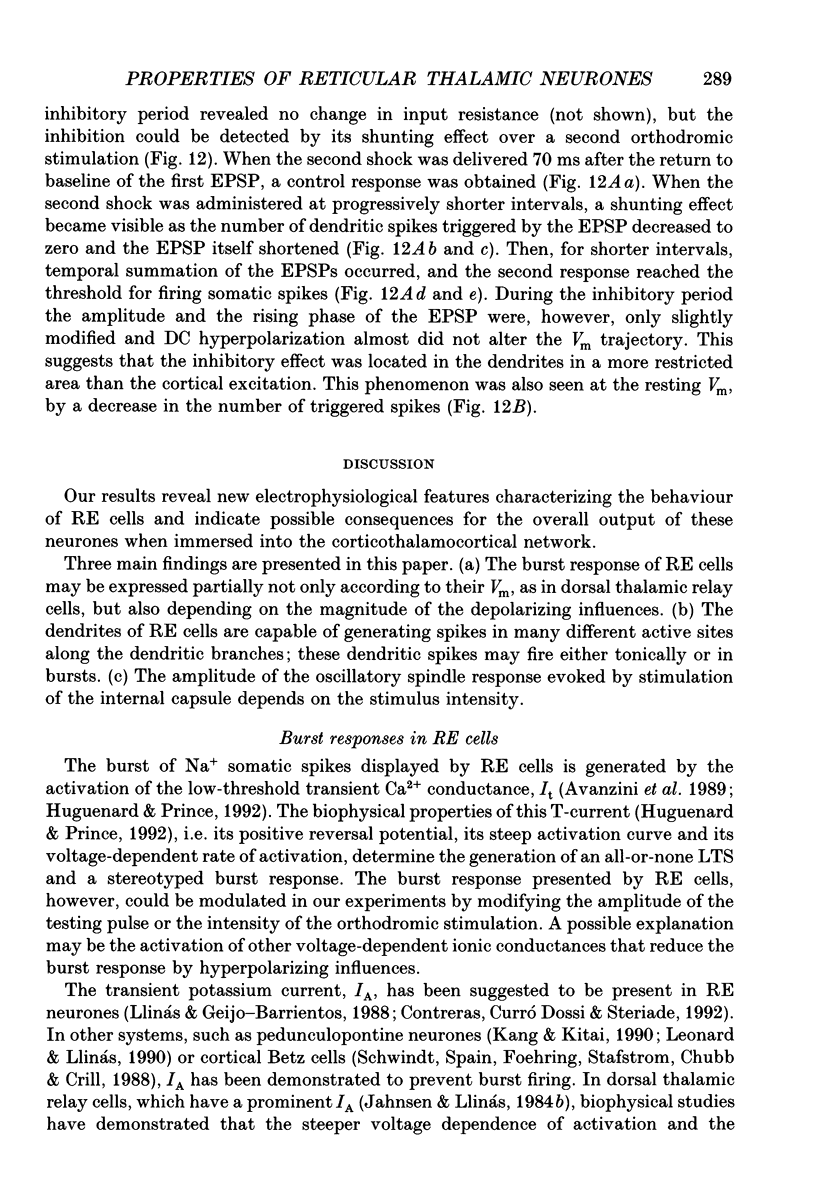
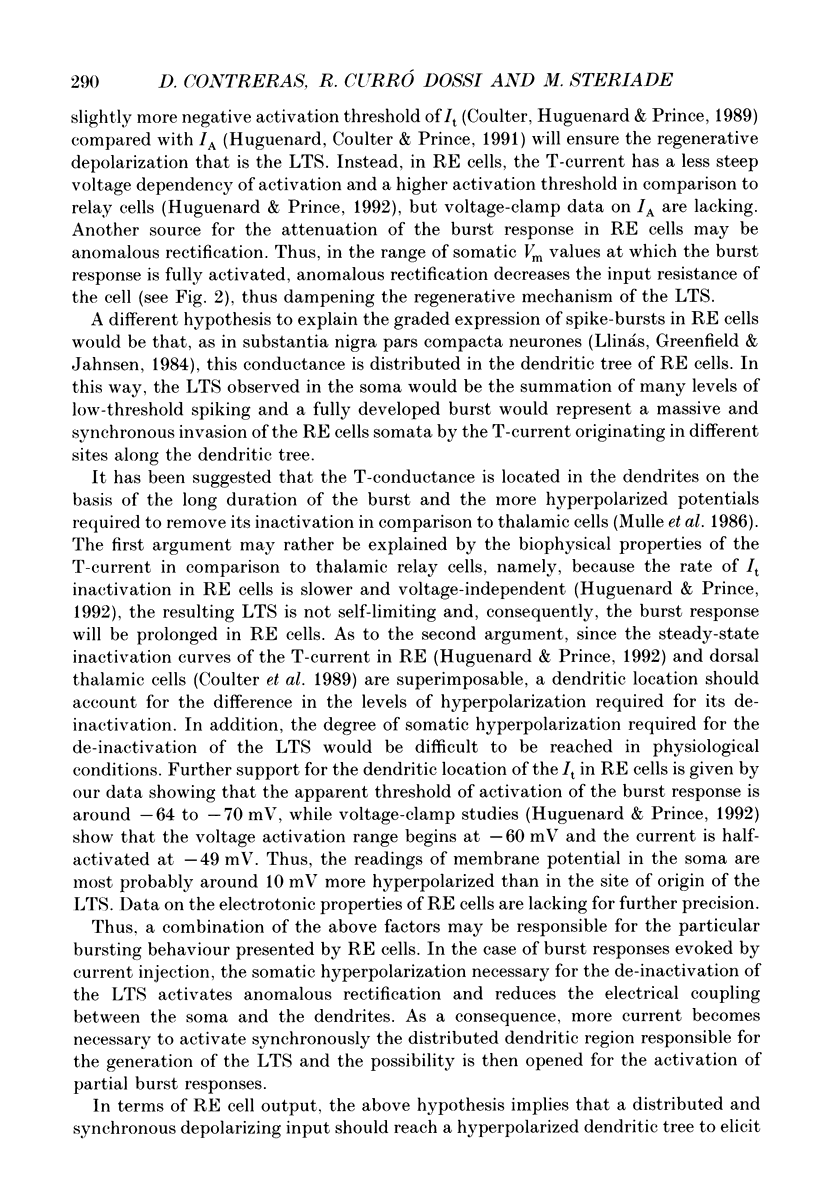
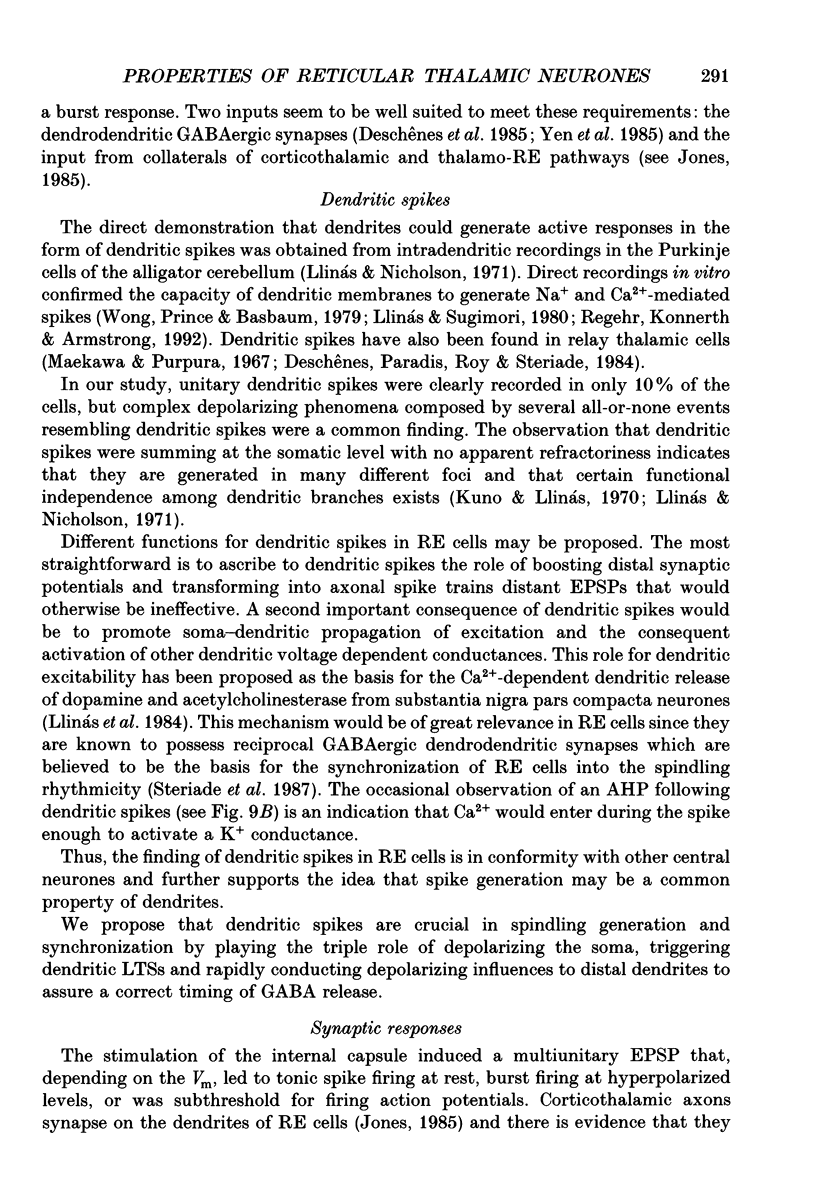
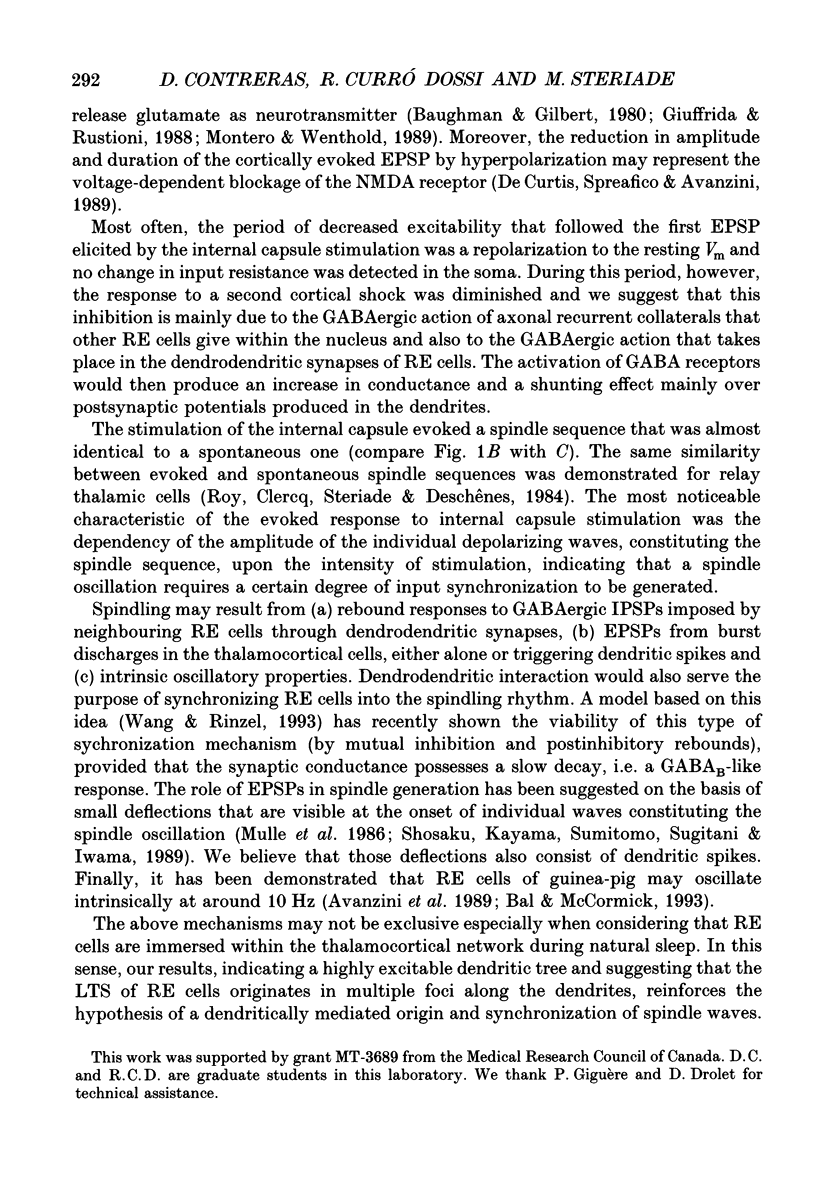
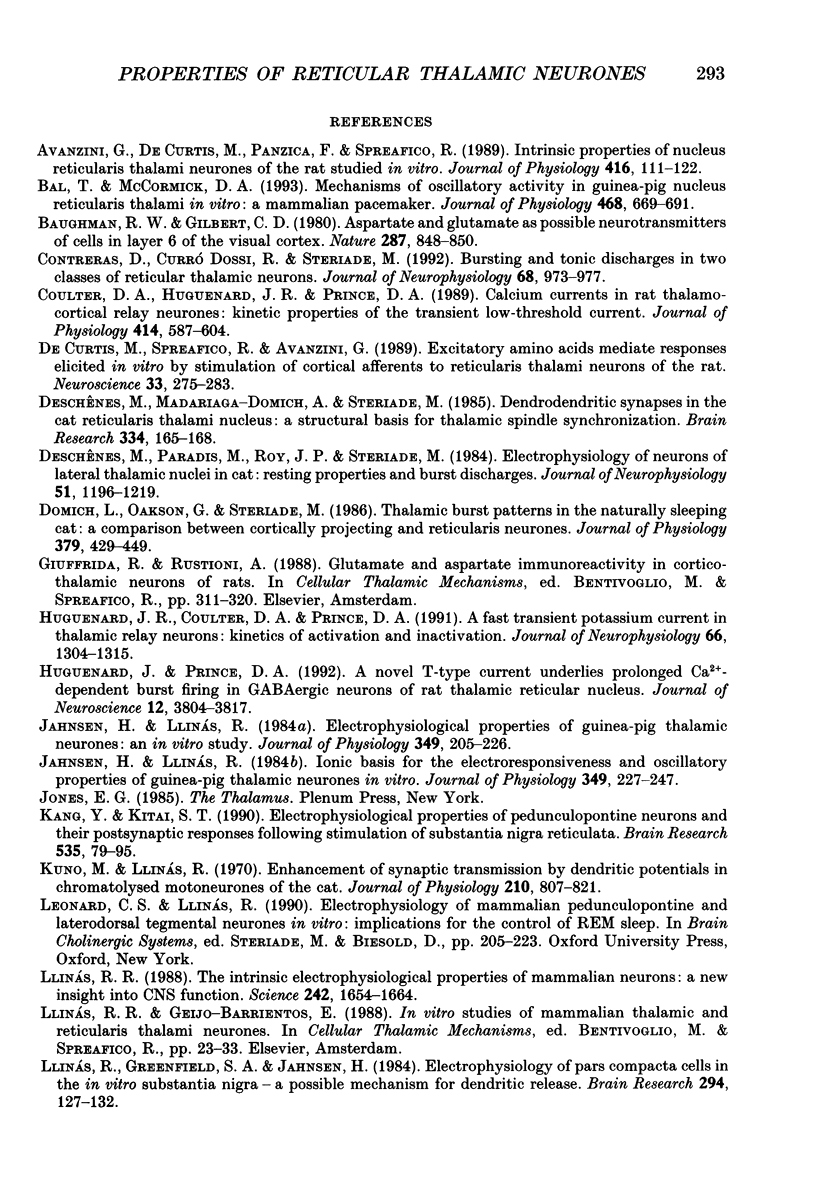
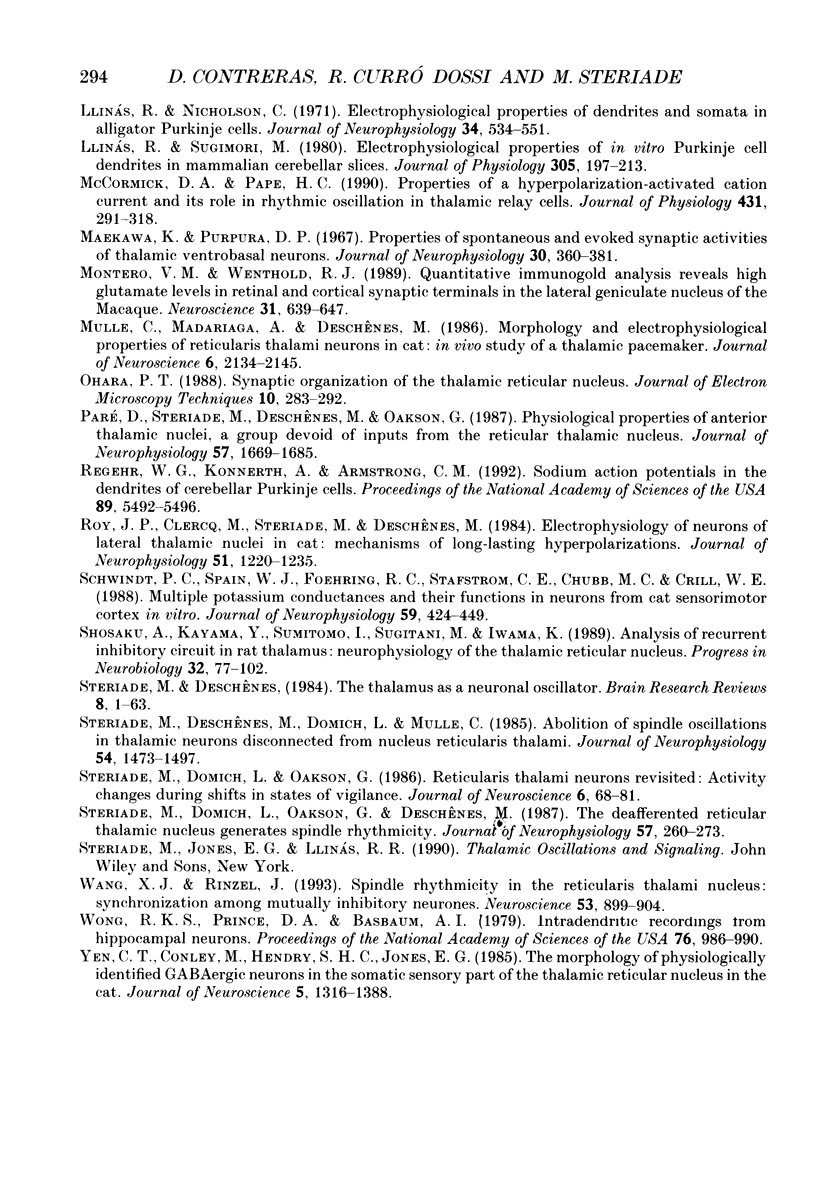
Selected References
These references are in PubMed. This may not be the complete list of references from this article.
- Avanzini G., de Curtis M., Panzica F., Spreafico R. Intrinsic properties of nucleus reticularis thalami neurones of the rat studied in vitro. J Physiol. 1989 Sep;416:111–122. doi: 10.1113/jphysiol.1989.sp017752. [DOI] [PMC free article] [PubMed] [Google Scholar]
- Bal T., McCormick D. A. Mechanisms of oscillatory activity in guinea-pig nucleus reticularis thalami in vitro: a mammalian pacemaker. J Physiol. 1993 Aug;468:669–691. doi: 10.1113/jphysiol.1993.sp019794. [DOI] [PMC free article] [PubMed] [Google Scholar]
- Baughman R. W., Gilbert C. D. Aspartate and glutamate as possible neurotransmitters of cells in layer 6 of the visual cortex. Nature. 1980 Oct 30;287(5785):848–850. doi: 10.1038/287848a0. [DOI] [PubMed] [Google Scholar]
- Contreras D., Curró Dossi R., Steriade M. Bursting and tonic discharges in two classes of reticular thalamic neurons. J Neurophysiol. 1992 Sep;68(3):973–977. doi: 10.1152/jn.1992.68.3.973. [DOI] [PubMed] [Google Scholar]
- Coulter D. A., Huguenard J. R., Prince D. A. Calcium currents in rat thalamocortical relay neurones: kinetic properties of the transient, low-threshold current. J Physiol. 1989 Jul;414:587–604. doi: 10.1113/jphysiol.1989.sp017705. [DOI] [PMC free article] [PubMed] [Google Scholar]
- Deschênes M., Madariaga-Domich A., Steriade M. Dendrodendritic synapses in the cat reticularis thalami nucleus: a structural basis for thalamic spindle synchronization. Brain Res. 1985 May 13;334(1):165–168. doi: 10.1016/0006-8993(85)90580-3. [DOI] [PubMed] [Google Scholar]
- Deschênes M., Paradis M., Roy J. P., Steriade M. Electrophysiology of neurons of lateral thalamic nuclei in cat: resting properties and burst discharges. J Neurophysiol. 1984 Jun;51(6):1196–1219. doi: 10.1152/jn.1984.51.6.1196. [DOI] [PubMed] [Google Scholar]
- Domich L., Oakson G., Steriade M. Thalamic burst patterns in the naturally sleeping cat: a comparison between cortically projecting and reticularis neurones. J Physiol. 1986 Oct;379:429–449. doi: 10.1113/jphysiol.1986.sp016262. [DOI] [PMC free article] [PubMed] [Google Scholar]
- Huguenard J. R., Coulter D. A., Prince D. A. A fast transient potassium current in thalamic relay neurons: kinetics of activation and inactivation. J Neurophysiol. 1991 Oct;66(4):1304–1315. doi: 10.1152/jn.1991.66.4.1304. [DOI] [PubMed] [Google Scholar]
- Huguenard J. R., Prince D. A. A novel T-type current underlies prolonged Ca(2+)-dependent burst firing in GABAergic neurons of rat thalamic reticular nucleus. J Neurosci. 1992 Oct;12(10):3804–3817. doi: 10.1523/JNEUROSCI.12-10-03804.1992. [DOI] [PMC free article] [PubMed] [Google Scholar]
- Jahnsen H., Llinás R. Electrophysiological properties of guinea-pig thalamic neurones: an in vitro study. J Physiol. 1984 Apr;349:205–226. doi: 10.1113/jphysiol.1984.sp015153. [DOI] [PMC free article] [PubMed] [Google Scholar]
- Jahnsen H., Llinás R. Ionic basis for the electro-responsiveness and oscillatory properties of guinea-pig thalamic neurones in vitro. J Physiol. 1984 Apr;349:227–247. doi: 10.1113/jphysiol.1984.sp015154. [DOI] [PMC free article] [PubMed] [Google Scholar]
- Kang Y., Kitai S. T. Electrophysiological properties of pedunculopontine neurons and their postsynaptic responses following stimulation of substantia nigra reticulata. Brain Res. 1990 Dec 3;535(1):79–95. doi: 10.1016/0006-8993(90)91826-3. [DOI] [PubMed] [Google Scholar]
- Kuno M., Llinás R. Enhancement of synaptic transmission by dendritic potentials in chromatolysed motoneurones of the cat. J Physiol. 1970 Nov;210(4):807–821. doi: 10.1113/jphysiol.1970.sp009243. [DOI] [PMC free article] [PubMed] [Google Scholar]
- Llinas R., Nicholson C. Electrophysiological properties of dendrites and somata in alligator Purkinje cells. J Neurophysiol. 1971 Jul;34(4):532–551. doi: 10.1152/jn.1971.34.4.532. [DOI] [PubMed] [Google Scholar]
- Llinás R. R. The intrinsic electrophysiological properties of mammalian neurons: insights into central nervous system function. Science. 1988 Dec 23;242(4886):1654–1664. doi: 10.1126/science.3059497. [DOI] [PubMed] [Google Scholar]
- Llinás R., Greenfield S. A., Jahnsen H. Electrophysiology of pars compacta cells in the in vitro substantia nigra--a possible mechanism for dendritic release. Brain Res. 1984 Feb 27;294(1):127–132. doi: 10.1016/0006-8993(84)91316-7. [DOI] [PubMed] [Google Scholar]
- Llinás R., Sugimori M. Electrophysiological properties of in vitro Purkinje cell dendrites in mammalian cerebellar slices. J Physiol. 1980 Aug;305:197–213. doi: 10.1113/jphysiol.1980.sp013358. [DOI] [PMC free article] [PubMed] [Google Scholar]
- Maekawa K., Purpura D. P. Properties of spontaneous and evoked synaptic activities of thalamic ventrobasal neurons. J Neurophysiol. 1967 Mar;30(2):360–381. doi: 10.1152/jn.1967.30.2.360. [DOI] [PubMed] [Google Scholar]
- McCormick D. A., Pape H. C. Properties of a hyperpolarization-activated cation current and its role in rhythmic oscillation in thalamic relay neurones. J Physiol. 1990 Dec;431:291–318. doi: 10.1113/jphysiol.1990.sp018331. [DOI] [PMC free article] [PubMed] [Google Scholar]
- Montero V. M., Wenthold R. J. Quantitative immunogold analysis reveals high glutamate levels in retinal and cortical synaptic terminals in the lateral geniculate nucleus of the macaque. Neuroscience. 1989;31(3):639–647. doi: 10.1016/0306-4522(89)90429-6. [DOI] [PubMed] [Google Scholar]
- Mulle C., Madariaga A., Deschênes M. Morphology and electrophysiological properties of reticularis thalami neurons in cat: in vivo study of a thalamic pacemaker. J Neurosci. 1986 Aug;6(8):2134–2145. doi: 10.1523/JNEUROSCI.06-08-02134.1986. [DOI] [PMC free article] [PubMed] [Google Scholar]
- Ohara P. T. Synaptic organization of the thalamic reticular nucleus. J Electron Microsc Tech. 1988 Nov;10(3):283–292. doi: 10.1002/jemt.1060100306. [DOI] [PubMed] [Google Scholar]
- Paré D., Steriade M., Deschênes M., Oakson G. Physiological characteristics of anterior thalamic nuclei, a group devoid of inputs from reticular thalamic nucleus. J Neurophysiol. 1987 Jun;57(6):1669–1685. doi: 10.1152/jn.1987.57.6.1669. [DOI] [PubMed] [Google Scholar]
- Regehr W. G., Konnerth A., Armstrong C. M. Sodium action potentials in the dendrites of cerebellar Purkinje cells. Proc Natl Acad Sci U S A. 1992 Jun 15;89(12):5492–5496. doi: 10.1073/pnas.89.12.5492. [DOI] [PMC free article] [PubMed] [Google Scholar]
- Roy J. P., Clercq M., Steriade M., Deschênes M. Electrophysiology of neurons of lateral thalamic nuclei in cat: mechanisms of long-lasting hyperpolarizations. J Neurophysiol. 1984 Jun;51(6):1220–1235. doi: 10.1152/jn.1984.51.6.1220. [DOI] [PubMed] [Google Scholar]
- Schwindt P. C., Spain W. J., Foehring R. C., Stafstrom C. E., Chubb M. C., Crill W. E. Multiple potassium conductances and their functions in neurons from cat sensorimotor cortex in vitro. J Neurophysiol. 1988 Feb;59(2):424–449. doi: 10.1152/jn.1988.59.2.424. [DOI] [PubMed] [Google Scholar]
- Shosaku A., Kayama Y., Sumitomo I., Sugitani M., Iwama K. Analysis of recurrent inhibitory circuit in rat thalamus: neurophysiology of the thalamic reticular nucleus. Prog Neurobiol. 1989;32(2):77–102. doi: 10.1016/0301-0082(89)90011-7. [DOI] [PubMed] [Google Scholar]
- Steriade M., Deschenes M. The thalamus as a neuronal oscillator. Brain Res. 1984 Nov;320(1):1–63. doi: 10.1016/0165-0173(84)90017-1. [DOI] [PubMed] [Google Scholar]
- Steriade M., Deschênes M., Domich L., Mulle C. Abolition of spindle oscillations in thalamic neurons disconnected from nucleus reticularis thalami. J Neurophysiol. 1985 Dec;54(6):1473–1497. doi: 10.1152/jn.1985.54.6.1473. [DOI] [PubMed] [Google Scholar]
- Steriade M., Domich L., Oakson G., Deschênes M. The deafferented reticular thalamic nucleus generates spindle rhythmicity. J Neurophysiol. 1987 Jan;57(1):260–273. doi: 10.1152/jn.1987.57.1.260. [DOI] [PubMed] [Google Scholar]
- Steriade M., Domich L., Oakson G. Reticularis thalami neurons revisited: activity changes during shifts in states of vigilance. J Neurosci. 1986 Jan;6(1):68–81. doi: 10.1523/JNEUROSCI.06-01-00068.1986. [DOI] [PMC free article] [PubMed] [Google Scholar]
- Wang X. J., Rinzel J. Spindle rhythmicity in the reticularis thalami nucleus: synchronization among mutually inhibitory neurons. Neuroscience. 1993 Apr;53(4):899–904. doi: 10.1016/0306-4522(93)90474-t. [DOI] [PubMed] [Google Scholar]
- Wong R. K., Prince D. A., Basbaum A. I. Intradendritic recordings from hippocampal neurons. Proc Natl Acad Sci U S A. 1979 Feb;76(2):986–990. doi: 10.1073/pnas.76.2.986. [DOI] [PMC free article] [PubMed] [Google Scholar]
- Yen C. T., Conley M., Jones E. G. Morphological and functional types of neurons in cat ventral posterior thalamic nucleus. J Neurosci. 1985 May;5(5):1316–1338. doi: 10.1523/JNEUROSCI.05-05-01316.1985. [DOI] [PMC free article] [PubMed] [Google Scholar]
- de Curtis M., Spreafico R., Avanzini G. Excitatory amino acids mediate responses elicited in vitro by stimulation of cortical afferents to reticularis thalami neurons of the rat. Neuroscience. 1989;33(2):275–283. doi: 10.1016/0306-4522(89)90207-8. [DOI] [PubMed] [Google Scholar]