Abstract
1. Microdialysis was used to gain insight into the substrate exchanges in the interstitial space of skeletal muscle and adipose tissue. Probes were inserted in the quadriceps femoris muscle and para-umbilical subcutaneous adipose tissue of thirteen subjects and microdialysis was performed at different flow rates (1-4 microliters min-1) and during changes in tissue blood flow. 2. When ethanol (5 mM) is included in the perfusion solution, the ethanol clearance from the probe is a measure of tissue blood flow. Blood flow changes induced by adenosine or vasopressin perfusion, by exercise or by circulatory occlusion resulted in ethanol clearance values of 69-139% of the basal level. The ethanol clearance was higher in skeletal muscle than in adipose tissue (32-62%, P < 0.001), a difference compatible with a higher blood flow in muscle tissue. 3. The fraction of the interstitial glucose concentration that was recovered with the microdialysis was similar in skeletal muscle (the absolute values being 1.70 +/- 0.14 mM at 1 microliter min-1 and 0.59 +/- 0.05 mM at 4 microliters min-1) and adipose tissue (1.89 +/- 0.20 mM at 1 microliter min-1; 0.54 +/- 0.05 mM at 4 microliters min-1) and correlated inversely with the tissue ethanol clearance, both in the basal state and during changes in tissue blood flow (muscle: r = -0.56 to -0.67; adipose tissue r = -0.72 to -0.95). Coefficients of variation were 6-8% (glucose) and 11-16% (lactate) and were similar during isometric exercise. The reproducibility of the technique (comparison of two contralateral probes; perfusion flow rate 4 microliters min-1) was 5.3-8.3% (ethanol) and 23.9-20.8% (glucose) in muscle (n = 6) and adipose tissue (n = 4) respectively. 4. The skeletal muscle dialysate lactate concentration (1 microliter min-1: 1.16 +/- 0.2 mM) was higher than in adipose tissue (0.76 +/- 0.08 mM, P < 0.05), where the absolute amount of lactate that could be removed from the tissue (at 4 microliters min-1) was only half of that in skeletal muscle (0.8 +/- 0.11 vs. 1.76 +/- 0.23 nmol min-1, P < 0.05). The dialysate lactate level was not affected in either tissue by large changes in the interstitial glucose concentration indicating that in neither tissue is blood glucose a significant source of lactate formation. 5. The blood flow effects on the dialysate glucose concentration are the likely consequence of probe glucose drainage artificially shifting the balance between the supply and consumption of interstitial glucose.(ABSTRACT TRUNCATED AT 400 WORDS)
Full text
PDF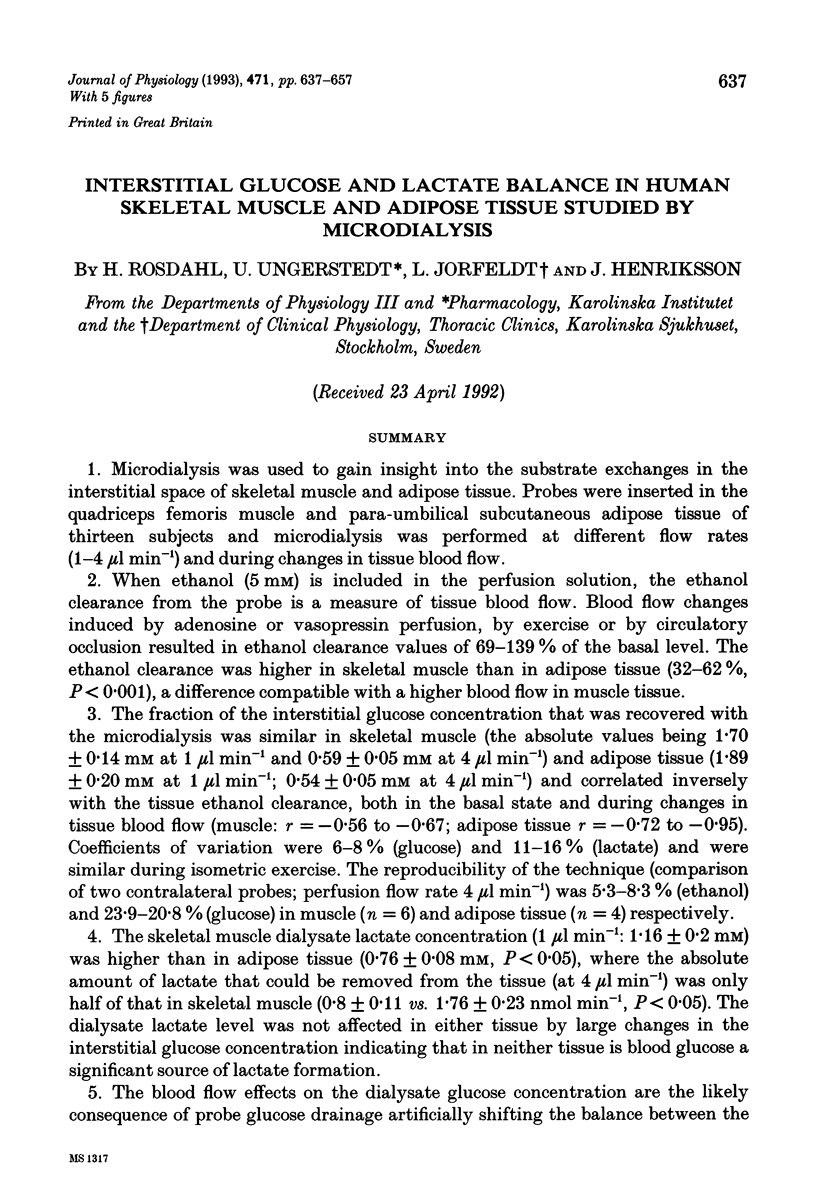
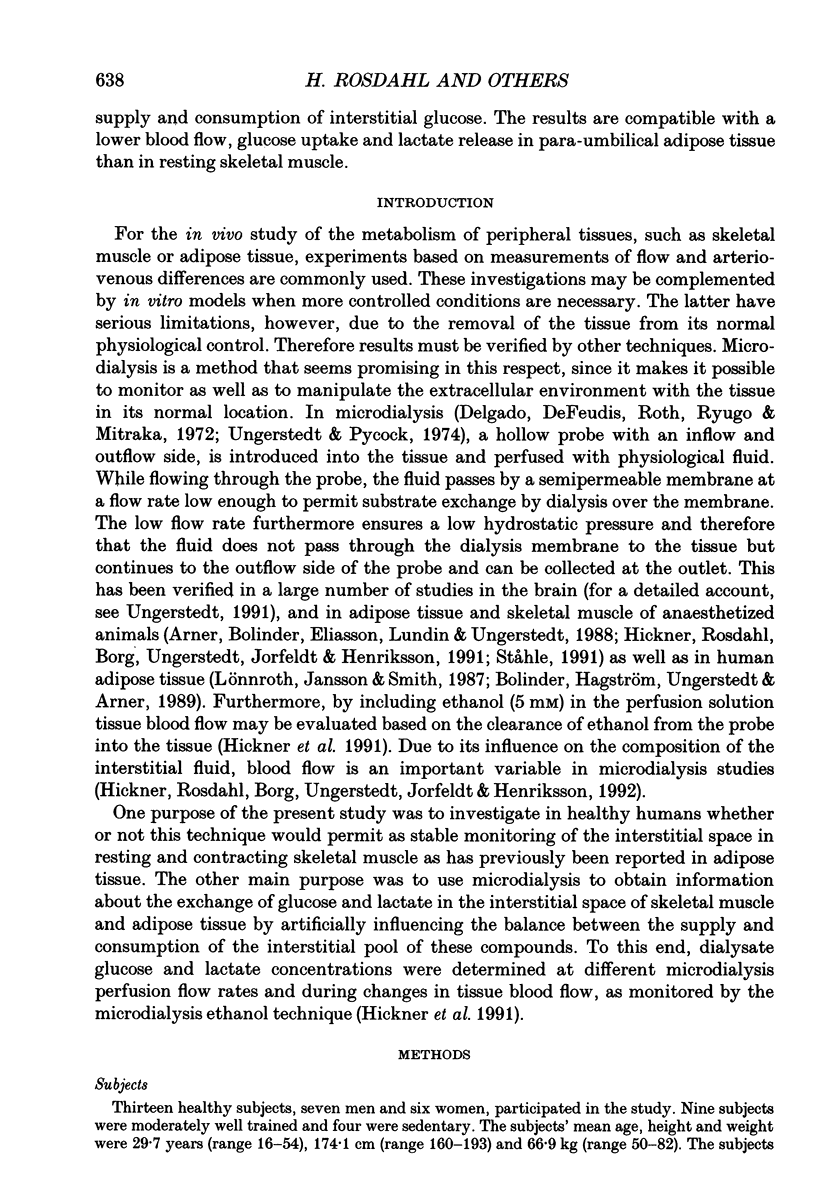
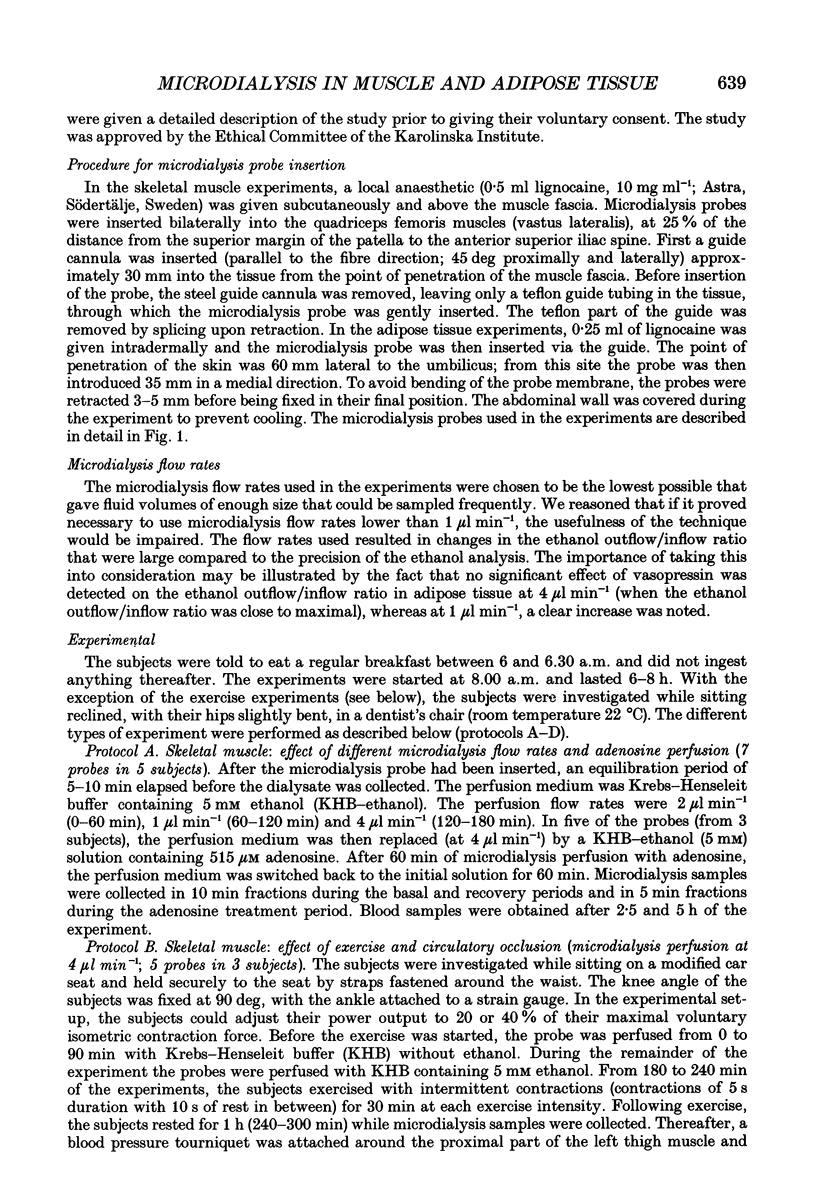
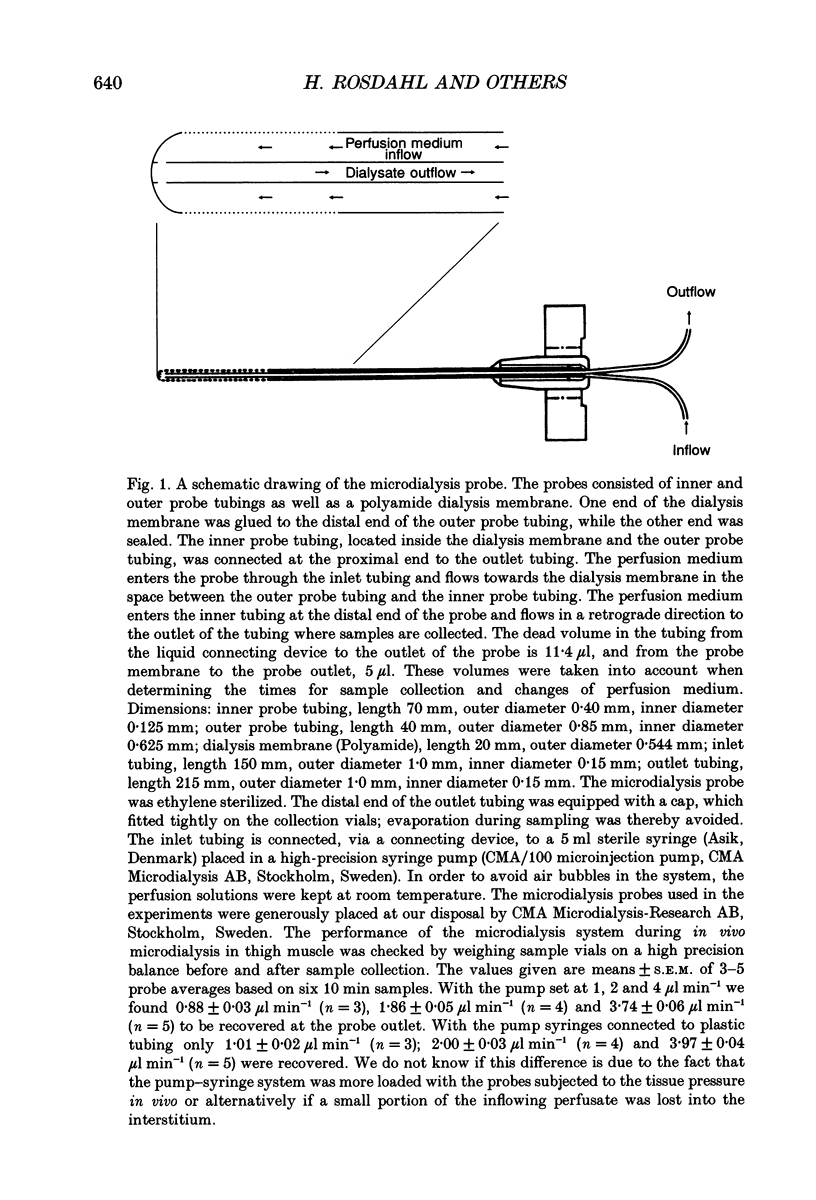
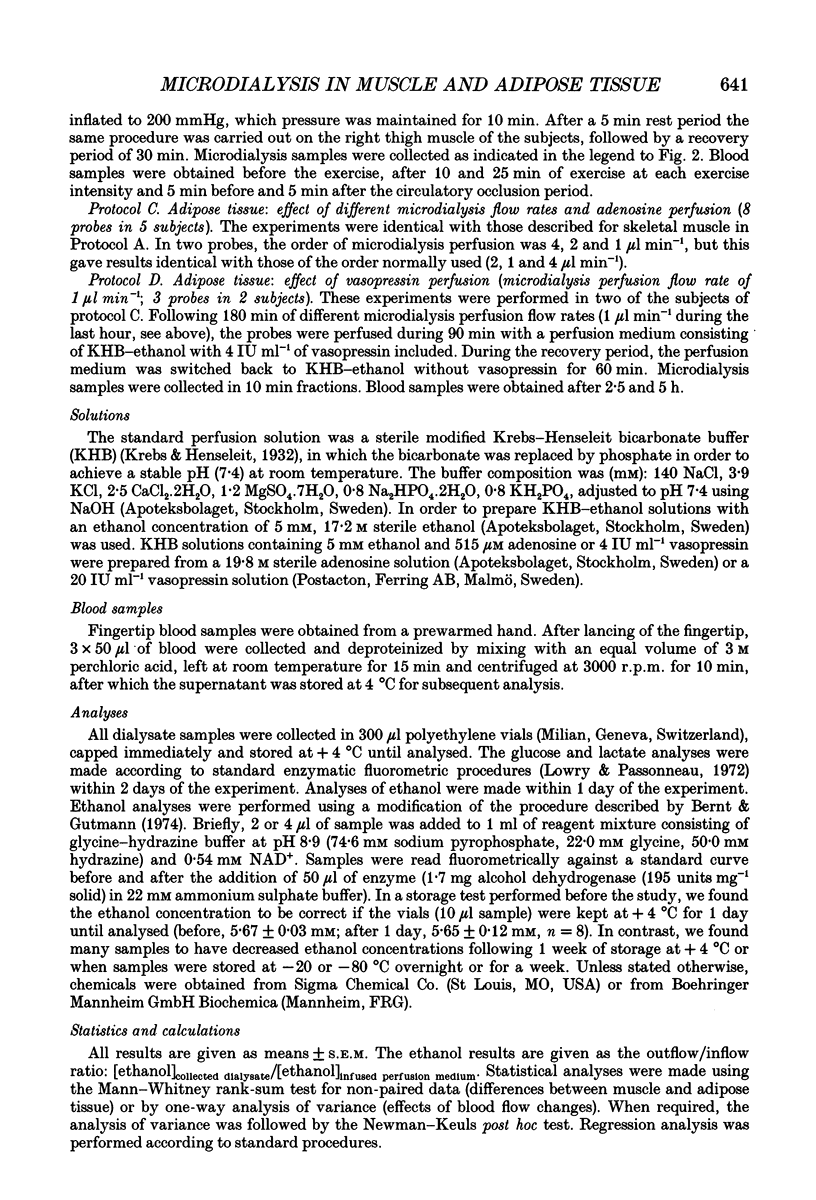
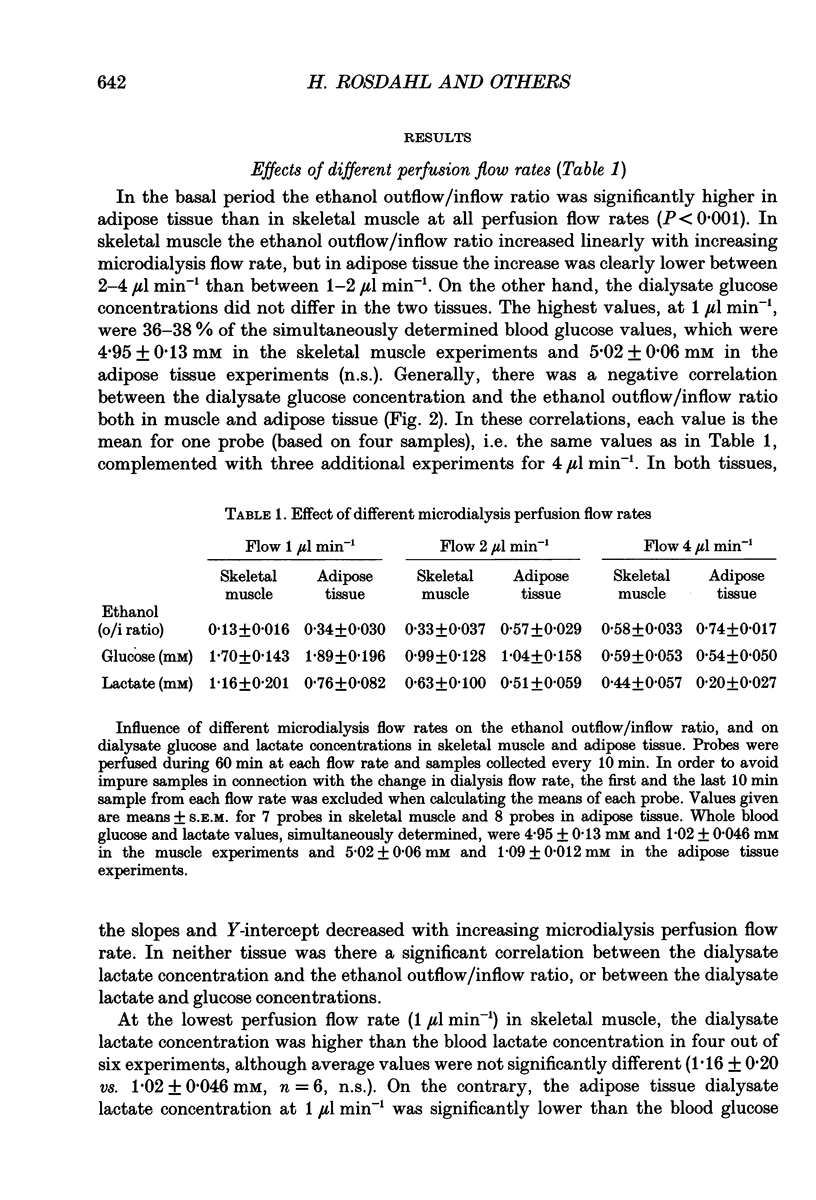
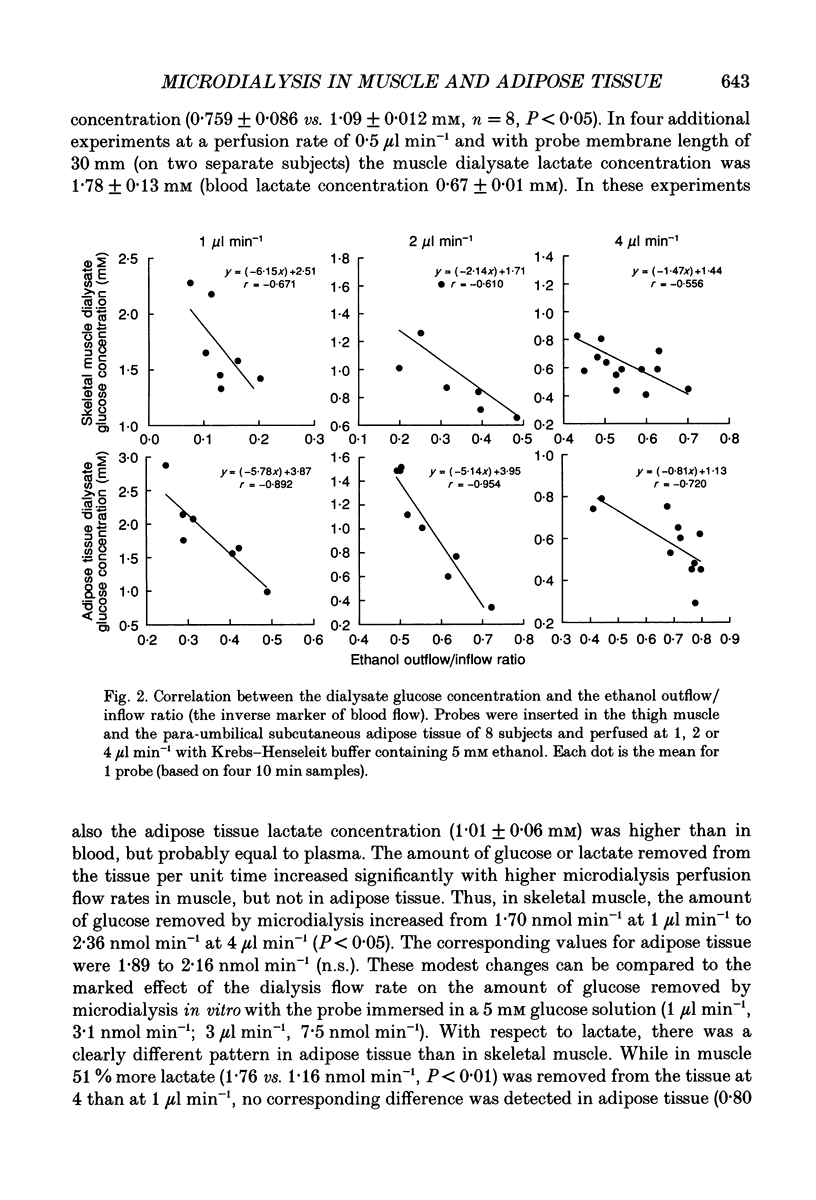
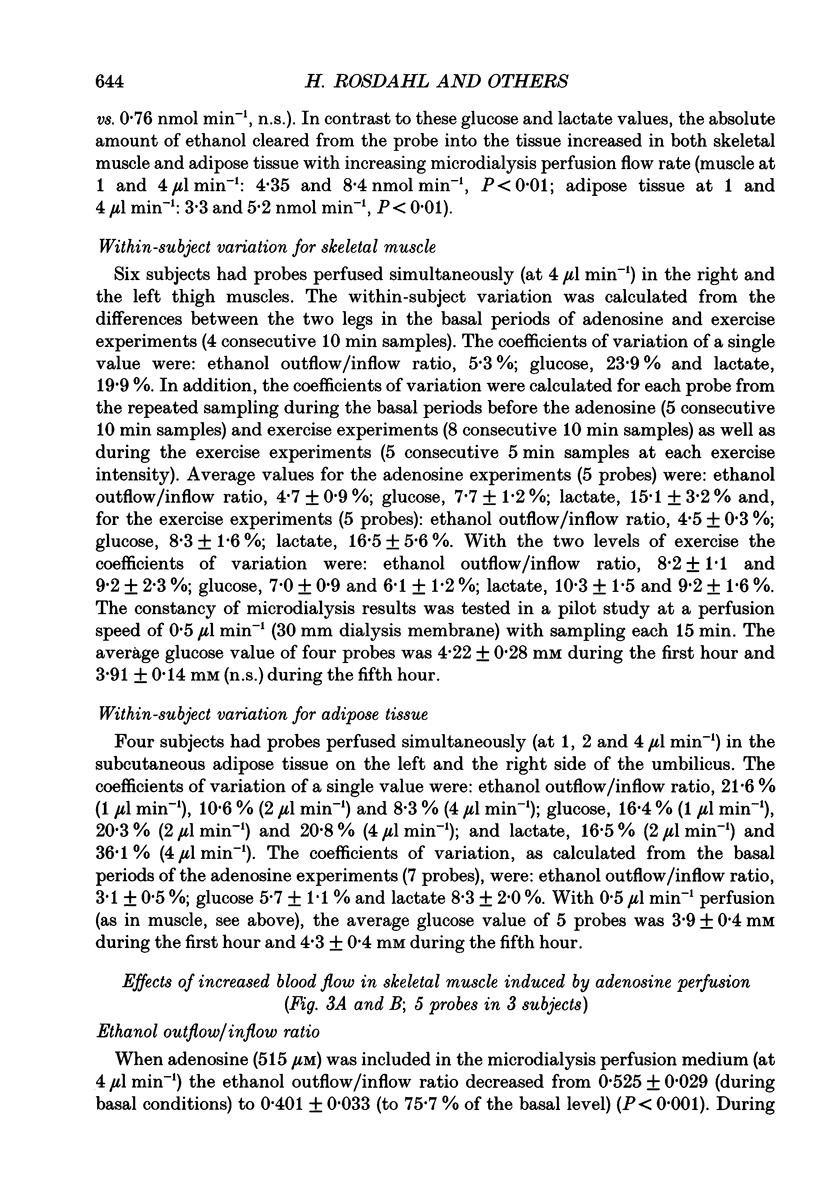
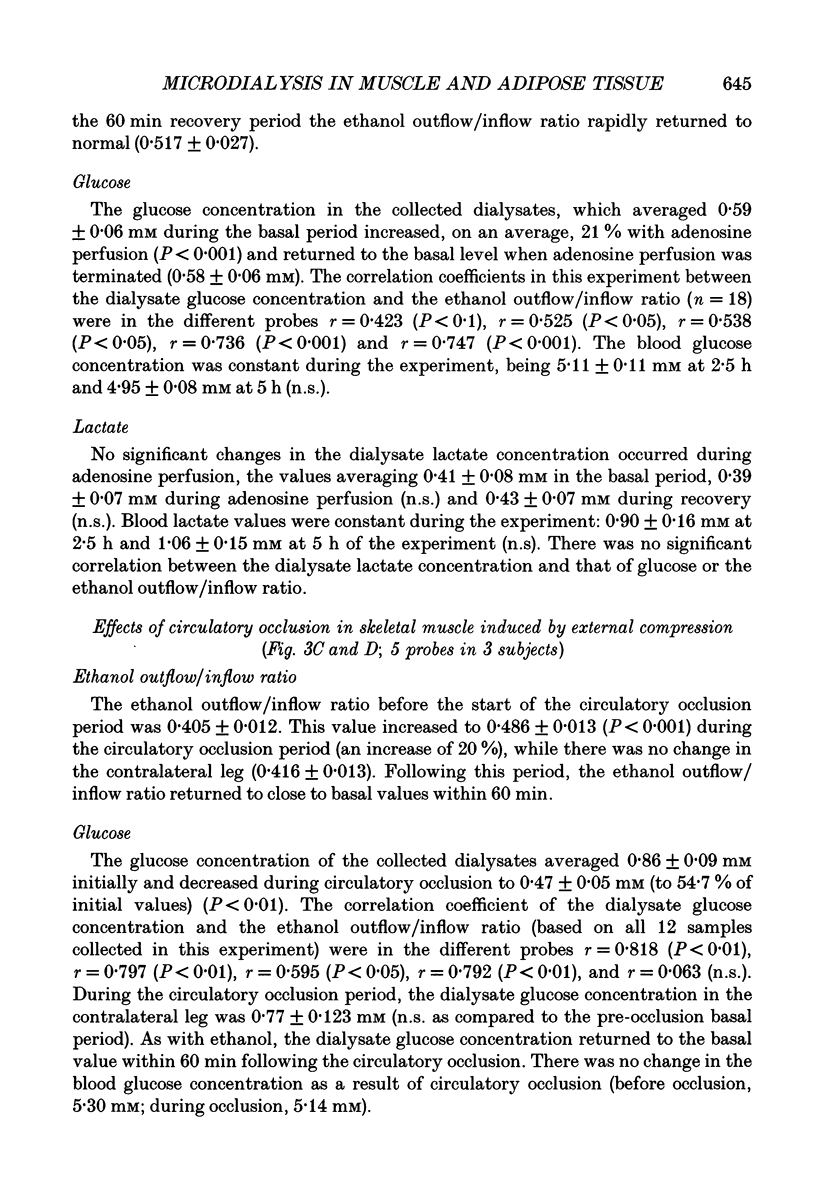
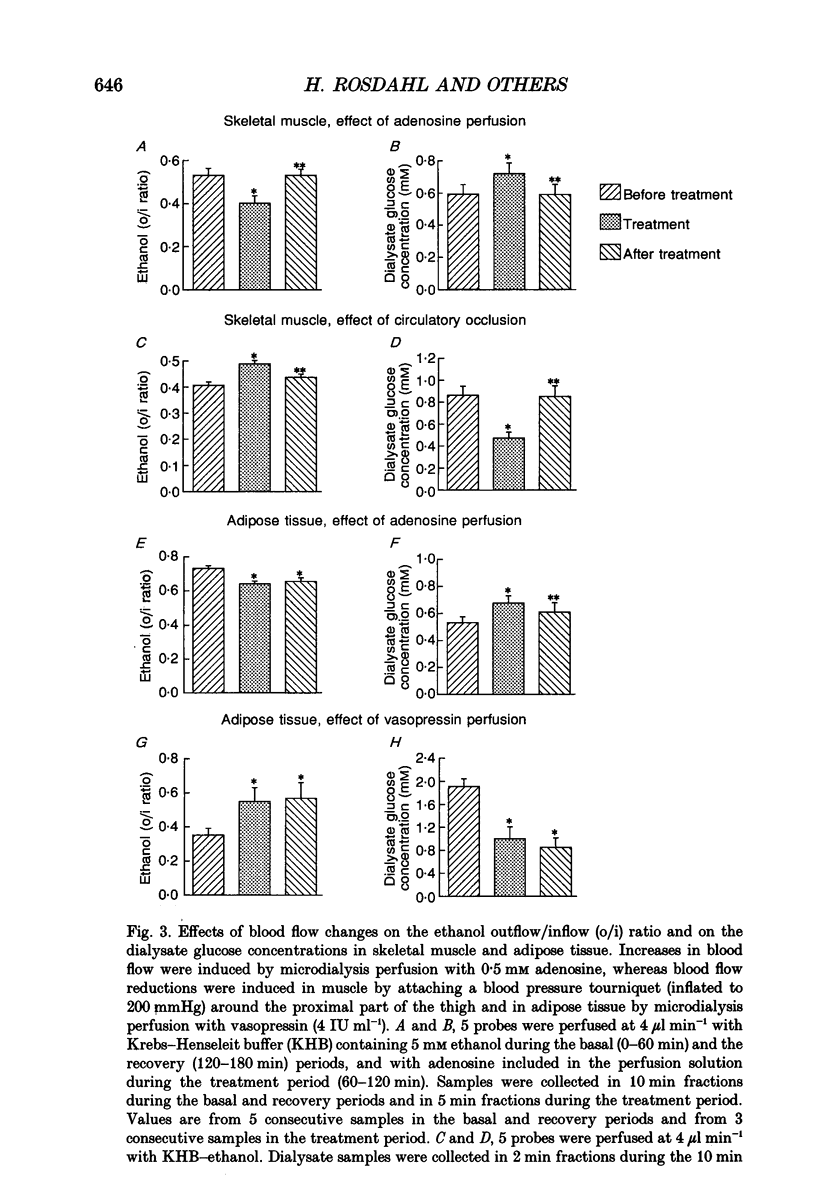
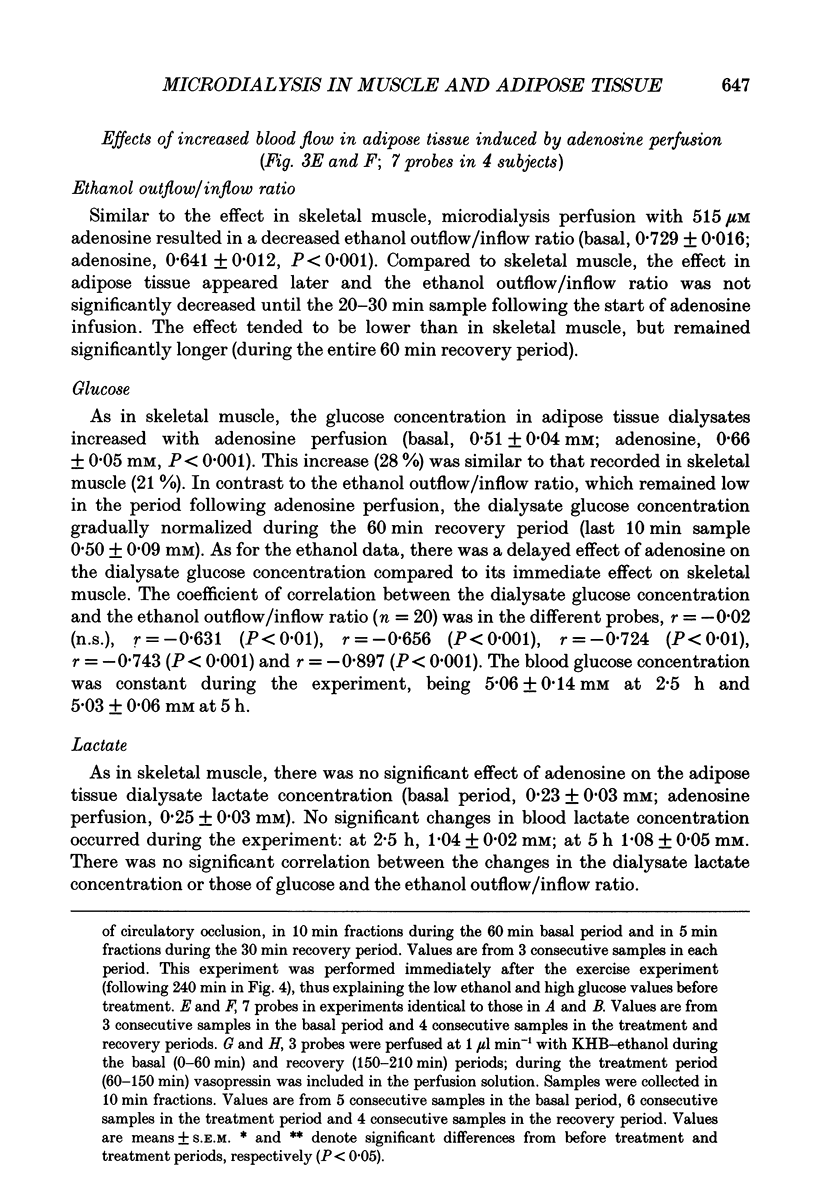
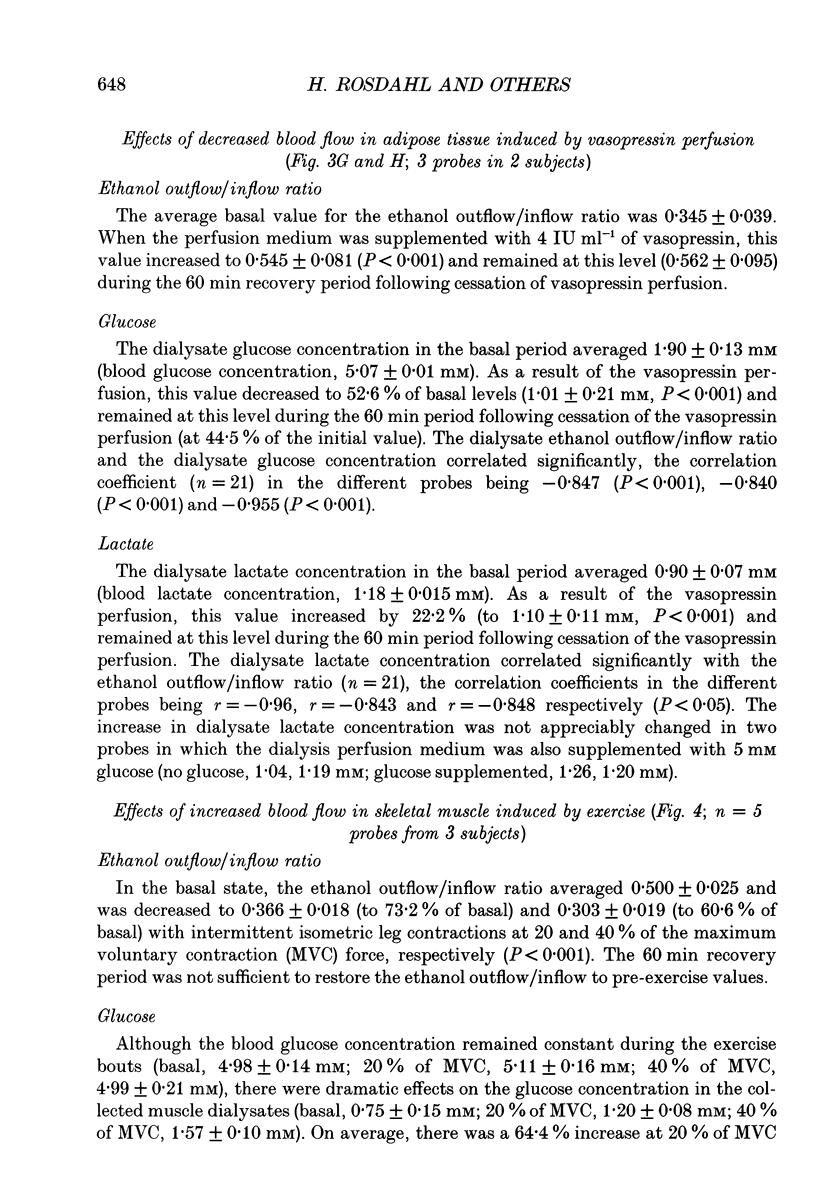
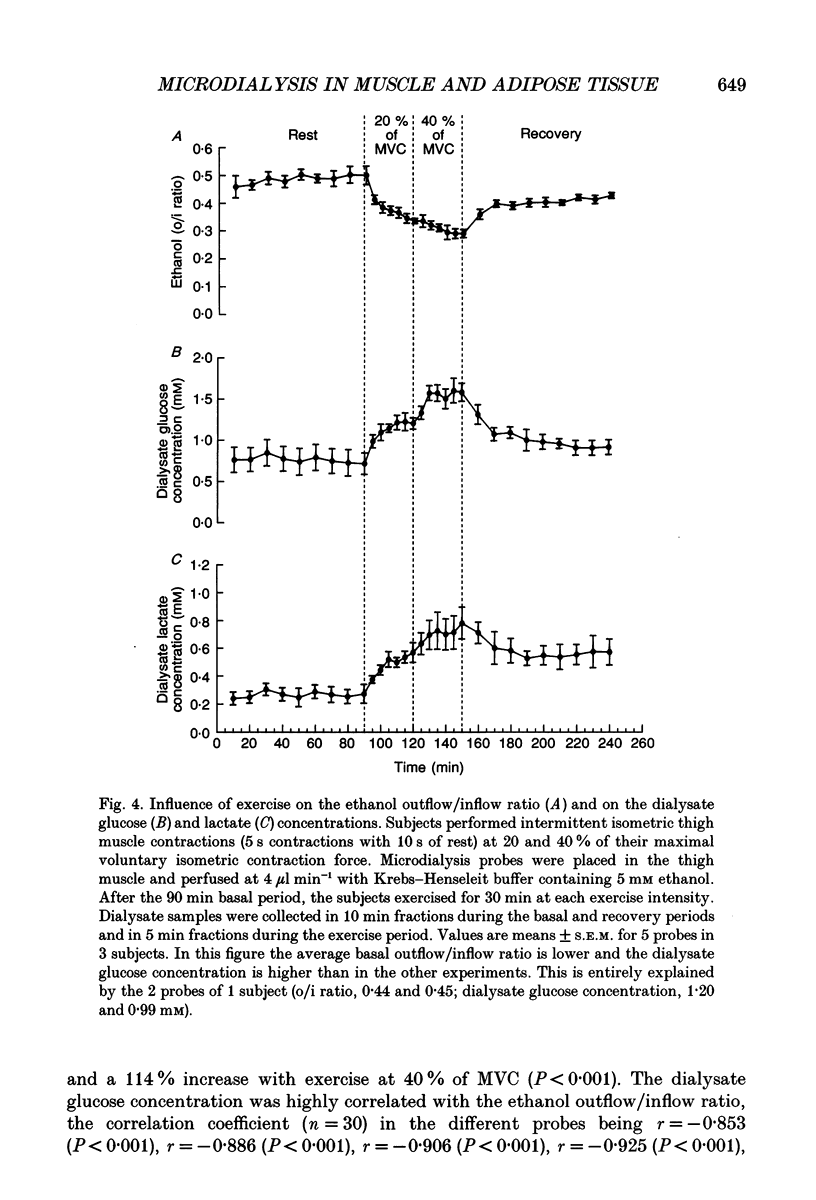
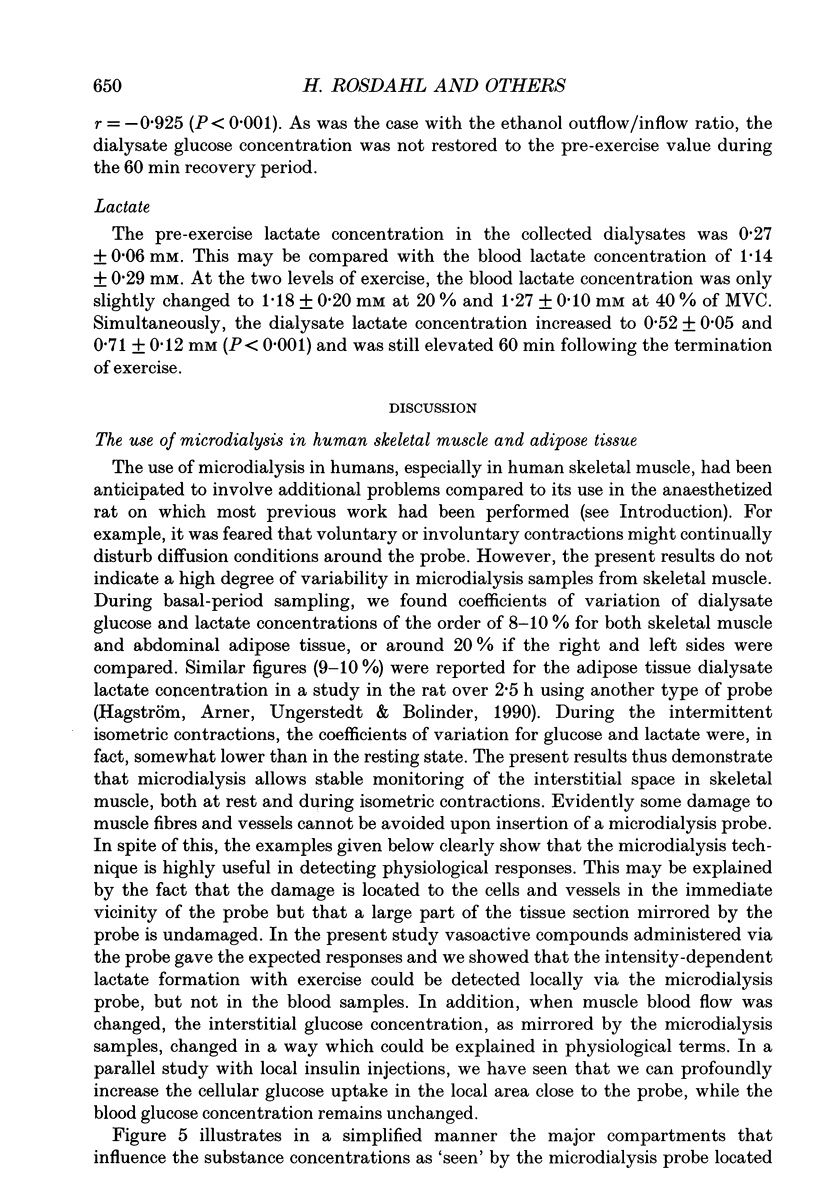
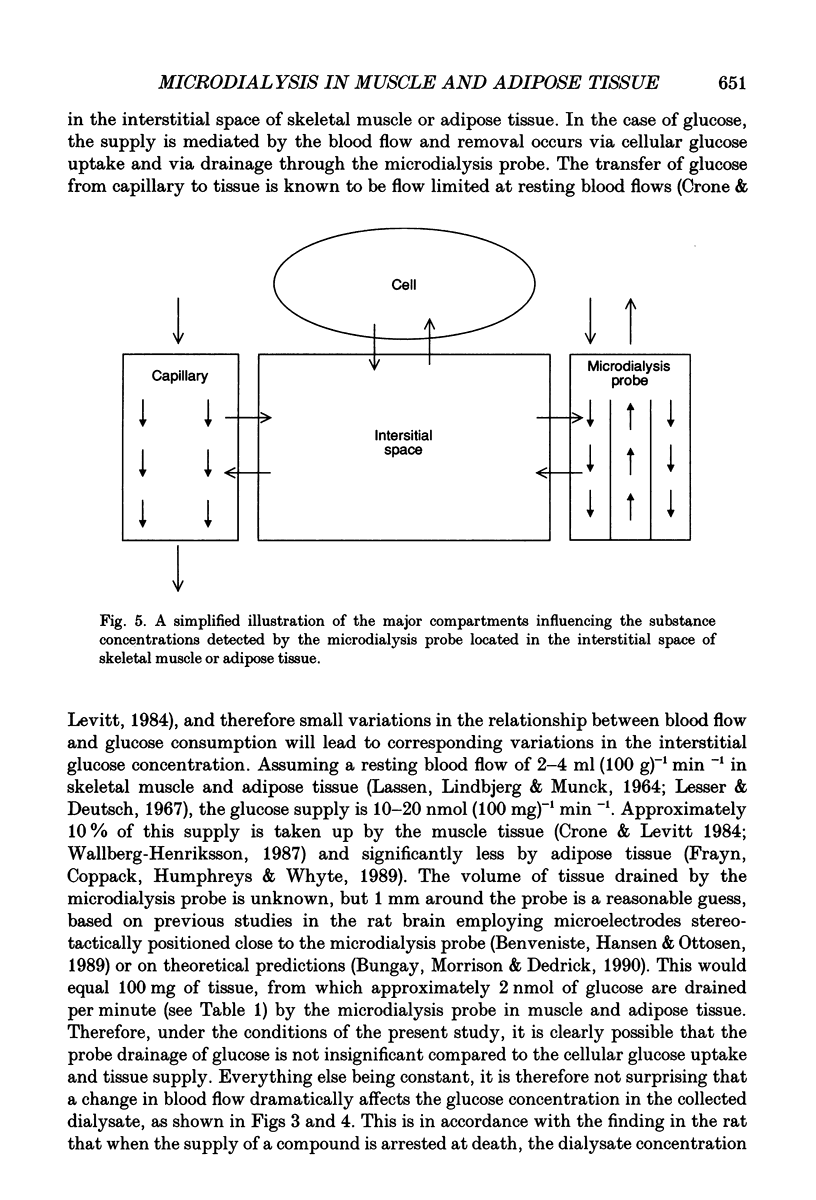
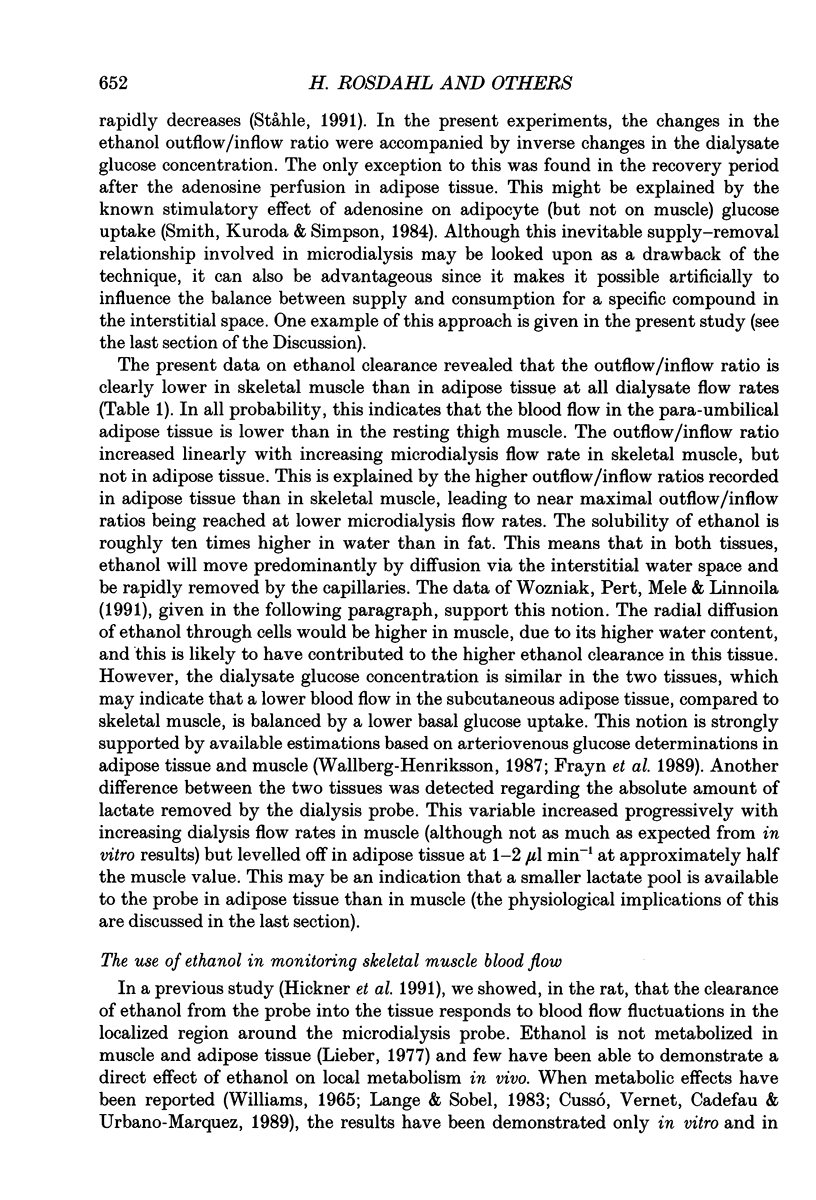
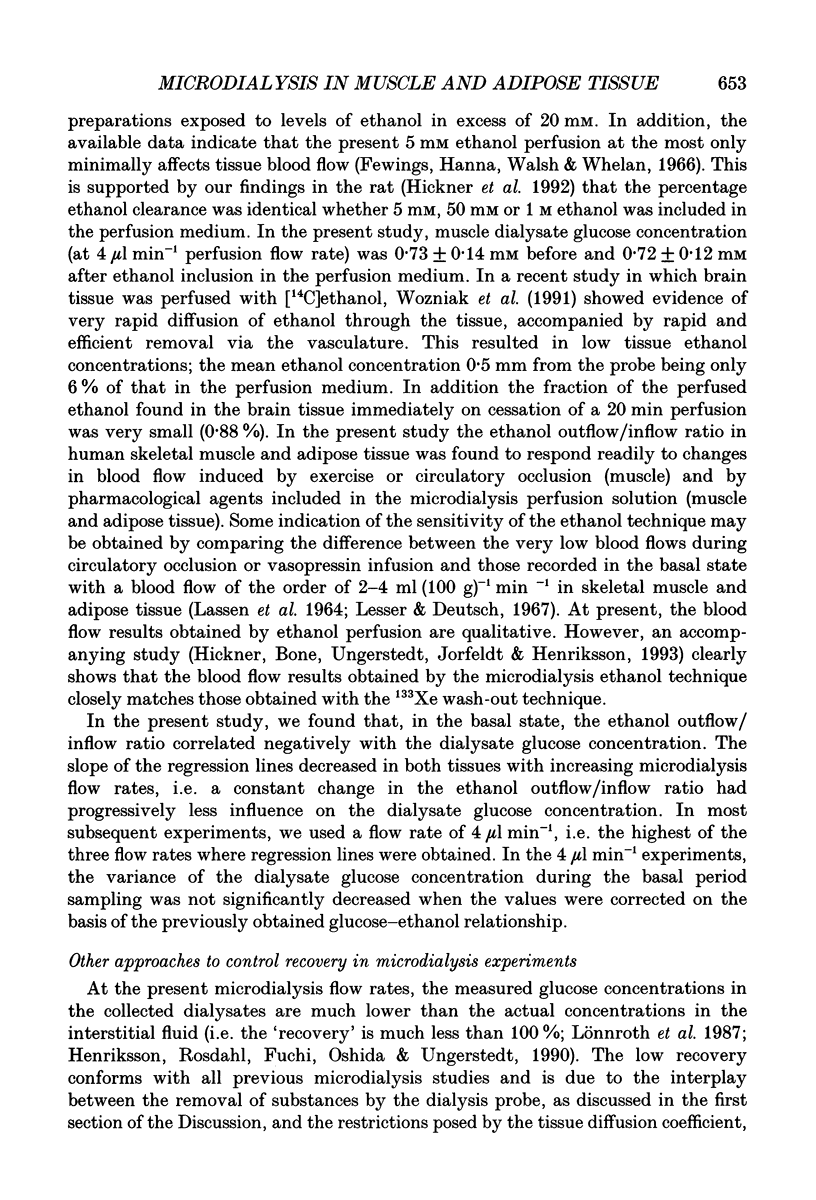
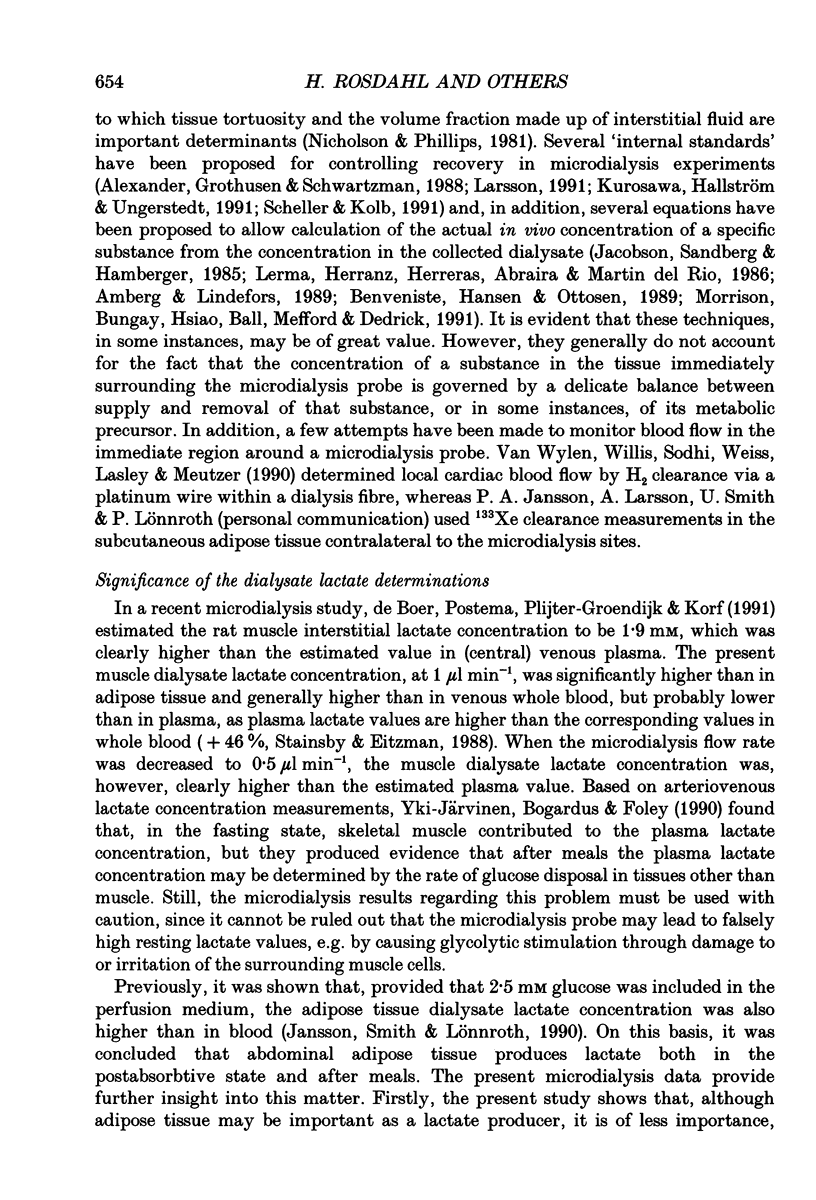
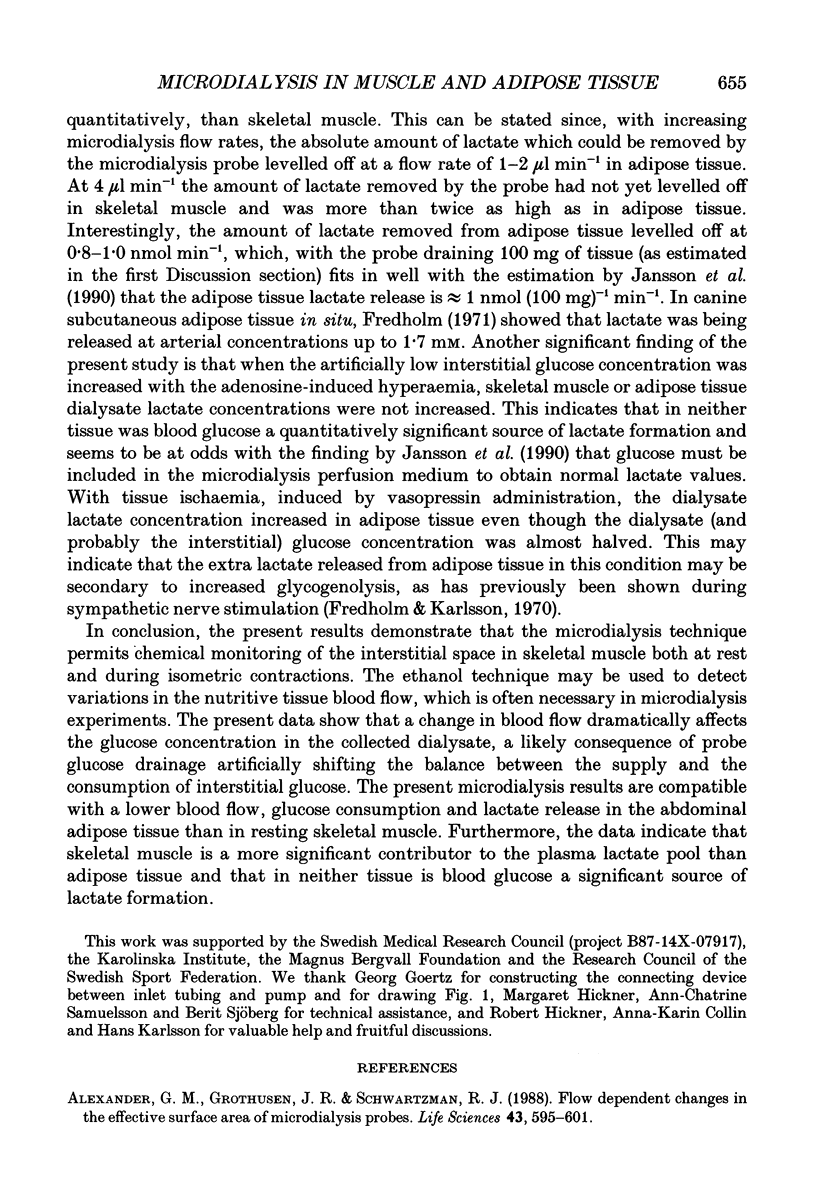
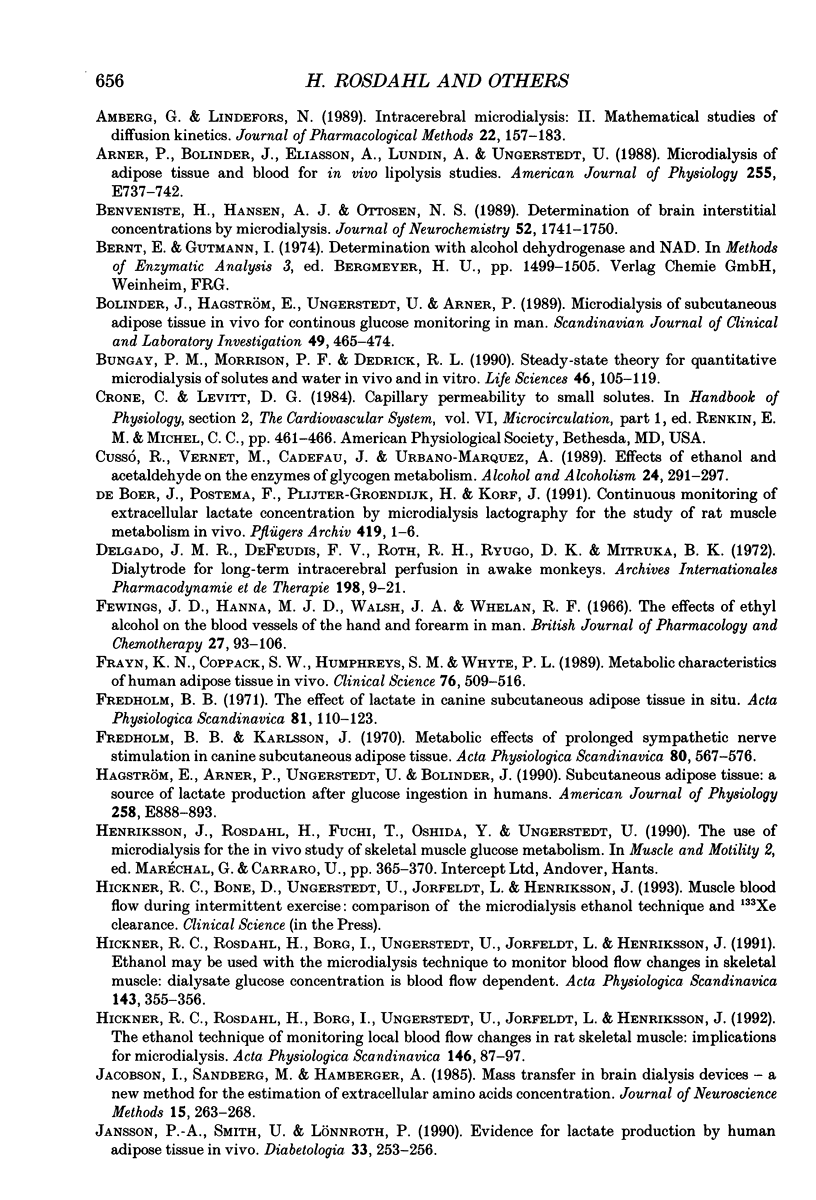
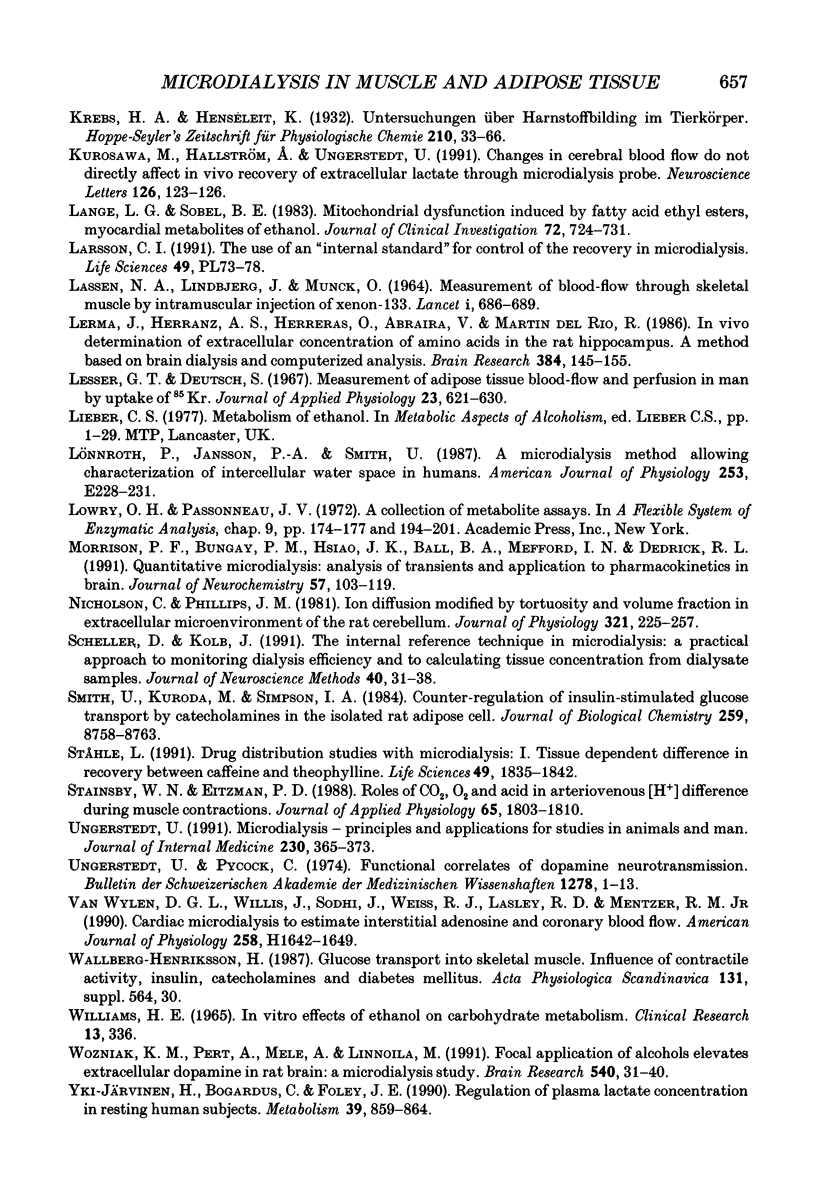
Selected References
These references are in PubMed. This may not be the complete list of references from this article.
- Alexander G. M., Grothusen J. R., Schwartzman R. J. Flow dependent changes in the effective surface area of microdialysis probes. Life Sci. 1988;43(7):595–601. doi: 10.1016/0024-3205(88)90063-x. [DOI] [PubMed] [Google Scholar]
- Amberg G., Lindefors N. Intracerebral microdialysis: II. Mathematical studies of diffusion kinetics. J Pharmacol Methods. 1989 Nov;22(3):157–183. doi: 10.1016/0160-5402(89)90012-0. [DOI] [PubMed] [Google Scholar]
- Arner P., Bolinder J., Eliasson A., Lundin A., Ungerstedt U. Microdialysis of adipose tissue and blood for in vivo lipolysis studies. Am J Physiol. 1988 Nov;255(5 Pt 1):E737–E742. doi: 10.1152/ajpendo.1988.255.5.E737. [DOI] [PubMed] [Google Scholar]
- Benveniste H., Hansen A. J., Ottosen N. S. Determination of brain interstitial concentrations by microdialysis. J Neurochem. 1989 Jun;52(6):1741–1750. doi: 10.1111/j.1471-4159.1989.tb07252.x. [DOI] [PubMed] [Google Scholar]
- Bolinder J., Hagström E., Ungerstedt U., Arner P. Microdialysis of subcutaneous adipose tissue in vivo for continuous glucose monitoring in man. Scand J Clin Lab Invest. 1989 Sep;49(5):465–474. doi: 10.1080/00365518909089123. [DOI] [PubMed] [Google Scholar]
- Bungay P. M., Morrison P. F., Dedrick R. L. Steady-state theory for quantitative microdialysis of solutes and water in vivo and in vitro. Life Sci. 1990;46(2):105–119. doi: 10.1016/0024-3205(90)90043-q. [DOI] [PubMed] [Google Scholar]
- Cussó R., Vernet M., Cadefau J., Urbano-Marquez A. Effects of ethanol and acetaldehyde on the enzymes of glycogen metabolism. Alcohol Alcohol. 1989;24(4):291–297. doi: 10.1093/oxfordjournals.alcalc.a044915. [DOI] [PubMed] [Google Scholar]
- Delgado J. M., DeFeudis F. V., Roth R. H., Ryugo D. K., Mitruka B. M. Dialytrode for long term intracerebral perfusion in awake monkeys. Arch Int Pharmacodyn Ther. 1972;198(1):9–21. [PubMed] [Google Scholar]
- Fewings J. D., Hanna M. J., Walsh J. A., Whelan R. F. The effects of ethyl alcohol on the blood vessels of the hand and forearm in man. Br J Pharmacol Chemother. 1966 May;27(1):93–106. doi: 10.1111/j.1476-5381.1966.tb01644.x. [DOI] [PMC free article] [PubMed] [Google Scholar]
- Frayn K. N., Coppack S. W., Humphreys S. M., Whyte P. L. Metabolic characteristics of human adipose tissue in vivo. Clin Sci (Lond) 1989 May;76(5):509–516. doi: 10.1042/cs0760509. [DOI] [PubMed] [Google Scholar]
- Fredholm B. B., Karlsson J. Metabolic effects of prolonged sympathetic nerve stimulation in canine subcutaneous adipose tissue. Acta Physiol Scand. 1970 Dec;80(4):567–576. doi: 10.1111/j.1748-1716.1970.tb04824.x. [DOI] [PubMed] [Google Scholar]
- Fredholm B. B. The effect of lactate in canine subcutaneous adipose tissue in situ. Acta Physiol Scand. 1971 Jan;81(1):110–123. doi: 10.1111/j.1748-1716.1971.tb04881.x. [DOI] [PubMed] [Google Scholar]
- Hagström E., Arner P., Ungerstedt U., Bolinder J. Subcutaneous adipose tissue: a source of lactate production after glucose ingestion in humans. Am J Physiol. 1990 May;258(5 Pt 1):E888–E893. doi: 10.1152/ajpendo.1990.258.5.E888. [DOI] [PubMed] [Google Scholar]
- Hickner R. C., Rosdahl H., Borg I., Ungerstedt U., Jorfeldt L., Henriksson J. Ethanol may be used with the microdialysis technique to monitor blood flow changes in skeletal muscle: dialysate glucose concentration is blood-flow-dependent. Acta Physiol Scand. 1991 Nov;143(3):355–356. doi: 10.1111/j.1748-1716.1991.tb09243.x. [DOI] [PubMed] [Google Scholar]
- Hickner R. C., Rosdahl H., Borg I., Ungerstedt U., Jorfeldt L., Henriksson J. The ethanol technique of monitoring local blood flow changes in rat skeletal muscle: implications for microdialysis. Acta Physiol Scand. 1992 Sep;146(1):87–97. doi: 10.1111/j.1748-1716.1992.tb09396.x. [DOI] [PubMed] [Google Scholar]
- Jacobson I., Sandberg M., Hamberger A. Mass transfer in brain dialysis devices--a new method for the estimation of extracellular amino acids concentration. J Neurosci Methods. 1985 Nov-Dec;15(3):263–268. doi: 10.1016/0165-0270(85)90107-4. [DOI] [PubMed] [Google Scholar]
- Jansson P. A., Smith U., Lönnroth P. Evidence for lactate production by human adipose tissue in vivo. Diabetologia. 1990 Apr;33(4):253–256. doi: 10.1007/BF00404805. [DOI] [PubMed] [Google Scholar]
- Kurosawa M., Hallström A., Ungerstedt U. Changes in cerebral blood flow do not directly affect in vivo recovery of extracellular lactate through microdialysis probe. Neurosci Lett. 1991 May 27;126(2):123–126. doi: 10.1016/0304-3940(91)90534-z. [DOI] [PubMed] [Google Scholar]
- LASSEN N. A., LINDBJERG J., MUNCK O. MEASUREMENT OF BLOOD-FLOW THROUGH SKELETAL MUSCLE BY INTRAMUSCULAR INJECTION OF XENON-133. Lancet. 1964 Mar 28;1(7335):686–689. doi: 10.1016/s0140-6736(64)91518-1. [DOI] [PubMed] [Google Scholar]
- Lange L. G., Sobel B. E. Mitochondrial dysfunction induced by fatty acid ethyl esters, myocardial metabolites of ethanol. J Clin Invest. 1983 Aug;72(2):724–731. doi: 10.1172/JCI111022. [DOI] [PMC free article] [PubMed] [Google Scholar]
- Lerma J., Herranz A. S., Herreras O., Abraira V., Martín del Río R. In vivo determination of extracellular concentration of amino acids in the rat hippocampus. A method based on brain dialysis and computerized analysis. Brain Res. 1986 Oct 1;384(1):145–155. doi: 10.1016/0006-8993(86)91230-8. [DOI] [PubMed] [Google Scholar]
- Lesser G. T., Deutsch S. Measurement of adipose tissue blood flow and perfusion in man by uptake of 85Kr. J Appl Physiol. 1967 Nov;23(5):621–630. doi: 10.1152/jappl.1967.23.5.621. [DOI] [PubMed] [Google Scholar]
- Lönnroth P., Jansson P. A., Smith U. A microdialysis method allowing characterization of intercellular water space in humans. Am J Physiol. 1987 Aug;253(2 Pt 1):E228–E231. doi: 10.1152/ajpendo.1987.253.2.E228. [DOI] [PubMed] [Google Scholar]
- Morrison P. F., Bungay P. M., Hsiao J. K., Ball B. A., Mefford I. N., Dedrick R. L. Quantitative microdialysis: analysis of transients and application to pharmacokinetics in brain. J Neurochem. 1991 Jul;57(1):103–119. doi: 10.1111/j.1471-4159.1991.tb02105.x. [DOI] [PubMed] [Google Scholar]
- Nicholson C., Phillips J. M. Ion diffusion modified by tortuosity and volume fraction in the extracellular microenvironment of the rat cerebellum. J Physiol. 1981 Dec;321:225–257. doi: 10.1113/jphysiol.1981.sp013981. [DOI] [PMC free article] [PubMed] [Google Scholar]
- Scheller D., Kolb J. The internal reference technique in microdialysis: a practical approach to monitoring dialysis efficiency and to calculating tissue concentration from dialysate samples. J Neurosci Methods. 1991 Nov;40(1):31–38. doi: 10.1016/0165-0270(91)90114-f. [DOI] [PubMed] [Google Scholar]
- Smith U., Kuroda M., Simpson I. A. Counter-regulation of insulin-stimulated glucose transport by catecholamines in the isolated rat adipose cell. J Biol Chem. 1984 Jul 25;259(14):8758–8763. [PubMed] [Google Scholar]
- Stainsby W. N., Eitzman P. D. Roles of CO2, O2, and acid in arteriovenous [H+] difference during muscle contractions. J Appl Physiol (1985) 1988 Oct;65(4):1803–1810. doi: 10.1152/jappl.1988.65.4.1803. [DOI] [PubMed] [Google Scholar]
- Ståhle L. Drug distribution studies with microdialysis: I. Tissue dependent difference in recovery between caffeine and theophylline. Life Sci. 1991;49(24):1835–1842. doi: 10.1016/0024-3205(91)90486-u. [DOI] [PubMed] [Google Scholar]
- Ungerstedt U. Microdialysis--principles and applications for studies in animals and man. J Intern Med. 1991 Oct;230(4):365–373. doi: 10.1111/j.1365-2796.1991.tb00459.x. [DOI] [PubMed] [Google Scholar]
- Van Wylen D. G., Willis J., Sodhi J., Weiss R. J., Lasley R. D., Mentzer R. M., Jr Cardiac microdialysis to estimate interstitial adenosine and coronary blood flow. Am J Physiol. 1990 Jun;258(6 Pt 2):H1642–H1649. doi: 10.1152/ajpheart.1990.258.6.H1642. [DOI] [PubMed] [Google Scholar]
- Wozniak K. M., Pert A., Mele A., Linnoila M. Focal application of alcohols elevates extracellular dopamine in rat brain: a microdialysis study. Brain Res. 1991 Feb 1;540(1-2):31–40. doi: 10.1016/0006-8993(91)90489-i. [DOI] [PubMed] [Google Scholar]
- Yki-Järvinen H., Bogardus C., Foley J. E. Regulation of plasma lactate concentration in resting human subjects. Metabolism. 1990 Aug;39(8):859–864. doi: 10.1016/0026-0495(90)90133-w. [DOI] [PubMed] [Google Scholar]
- de Boer J., Postema F., Plijter-Groendijk H., Korf J. Continuous monitoring of extracellular lactate concentration by microdialysis lactography for the study of rat muscle metabolism in vivo. Pflugers Arch. 1991 Aug;419(1):1–6. doi: 10.1007/BF00373739. [DOI] [PubMed] [Google Scholar]