Abstract
A preparation of isolated rat hindleg was perfused with a medium consisting of bicarbonate buffer containing Ficoll and fluorocarbon, containing glucose and/or lactate. The leg was electrically prestimulated to deplete partially muscle glycogen. The glucose was labelled uniformly with 14C and with 3H in positions 2, 5 or 6, and lactate uniformly with 14C and with 3H in positions 2 or 3. Glucose carbon was predominantly recovered in glycogen, and to a lesser extent in lactate. The 3H/14C ration in glycogen from [5-3H,U-14C]- and [6-3H,U-14C]-glucose was the same as in glucose. Nearly all the utilized 3H from [2-3H]glucose was recovered as water. Insulin increased glucose uptake and glycogen synthesis 3-fold. When the muscle was perfused with a medium containing 10 mM-glucose and 2 mM-lactate, there was little change in lactate concentration. 14C from lactate was incorporated into glycogen. There was a marked exponential decrease in lactate specific radioactivity, much greater with [3H]- than with [14C]-lactate. The 'apparent turnover' of [U-14C]lactate was 0.28 mumol/min per g of muscle, and those of [2-3H]- and [3-3H]-lactate were both about 0.7 mumol/min per g. With 10 mM-lactate as sole substrate, there was a net uptake of lactate, at a rate of about 0.15 mumol/min per g, and the apparent turnover of [U-14C]lactate was 0.3 mumol/min per g. The apparent turnover of [3H]lactate was 3-5 times greater. When glycogen synthesis was low (no prestimulation, no insulin), the incorporation of lactate carbon into glycogen exceeded that from glucose, but at high rates of glycogen deposition the incorporation of lactate carbon was much less than that of glucose. Lactate incorporation into glycogen was similar in fast-twitch white and fast-twitch red muscle, but was very low in slow-twitch red fibres. We find that (a) pyruvate in muscle is incorporated into glycogen without randomization of carbon, and synthesis is not inhibited by mercaptopicolinate or cycloserine; (b) there is extensive lactate turnover in the absence of net lactate uptake, and there is a large dilution of 14C-labelled lactate from endogenous supply; (c) there is extensive detritiation of [2-3H]- and [3-3H]-lactate in excess of 14C utilization.
Full text
PDF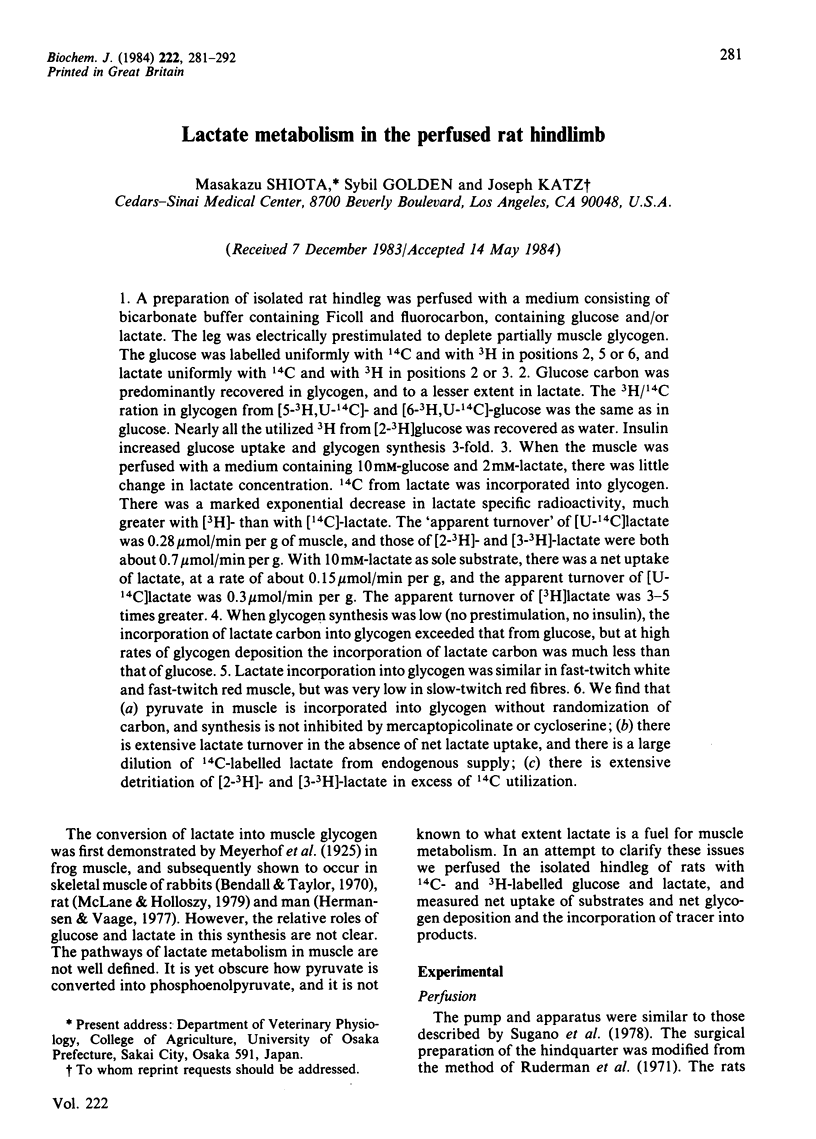
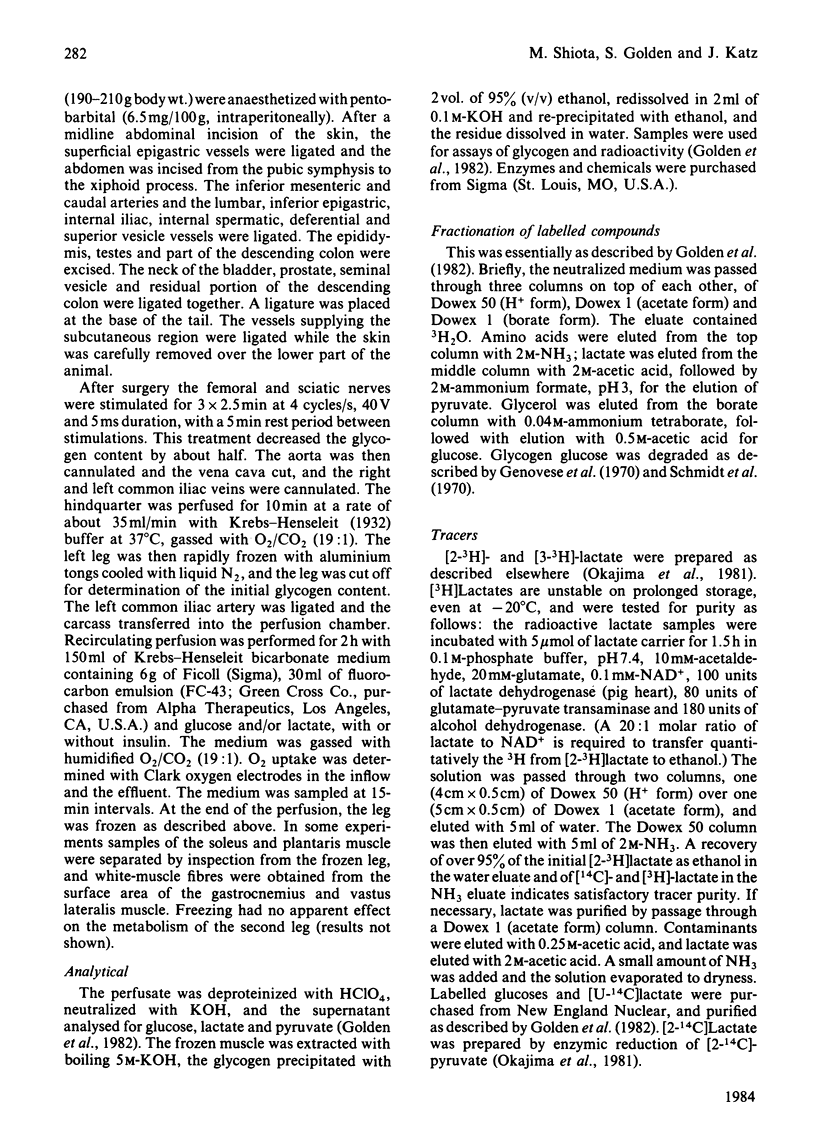
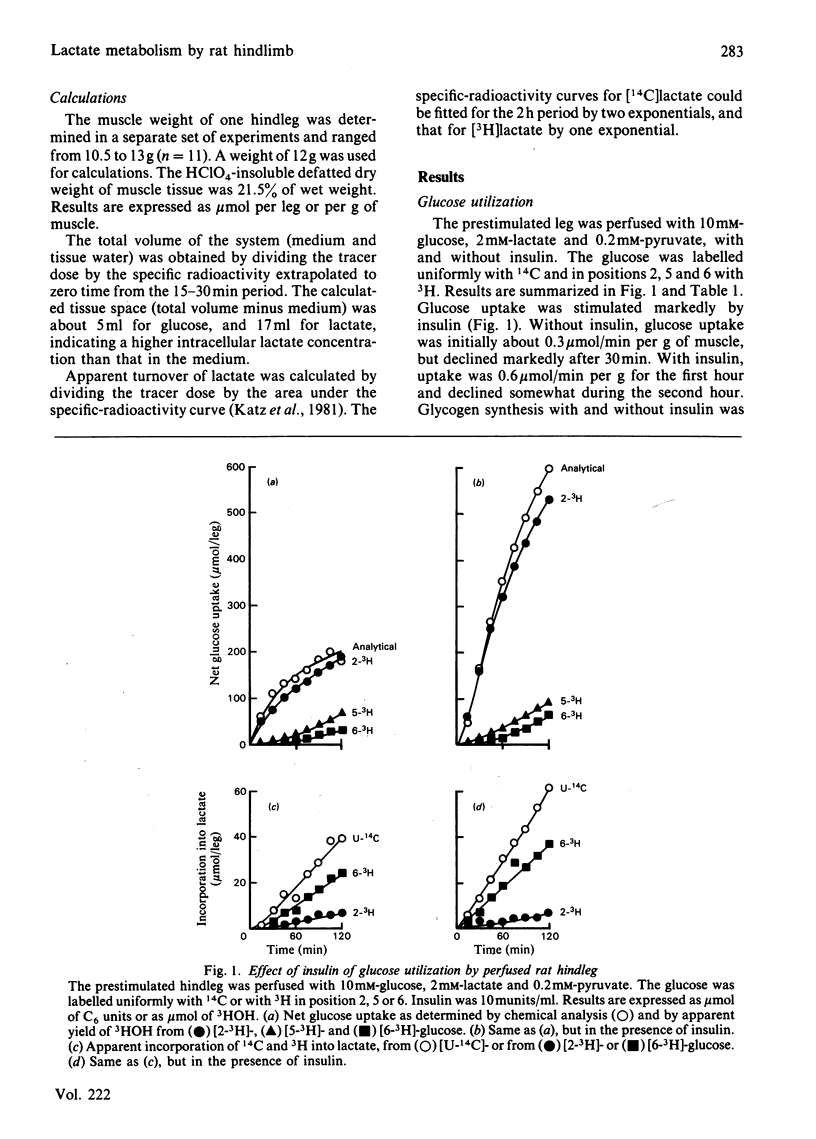
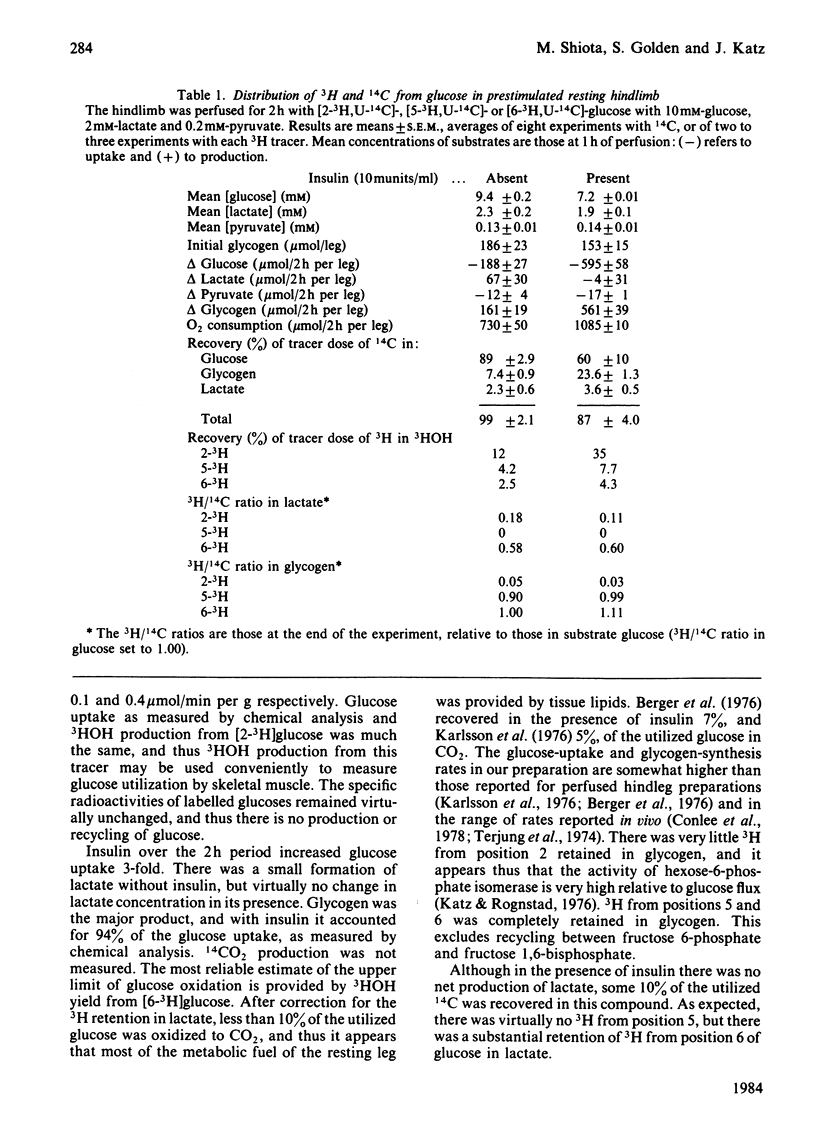
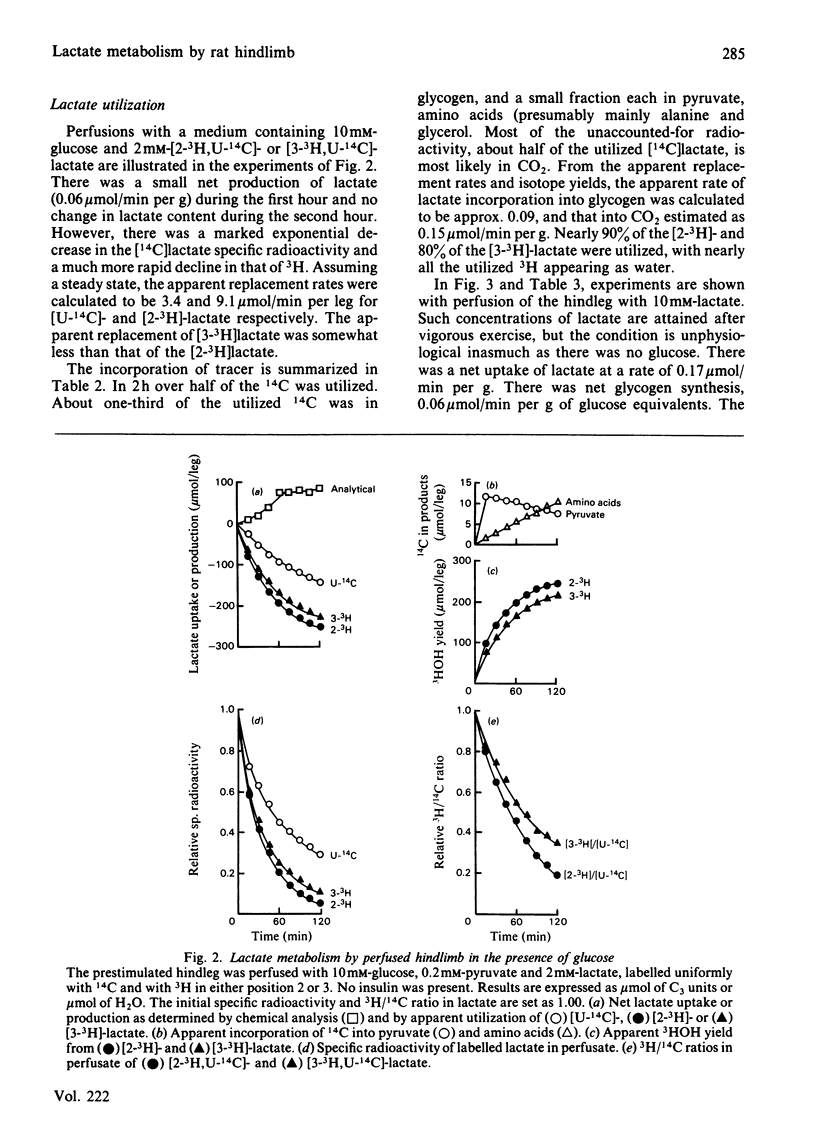
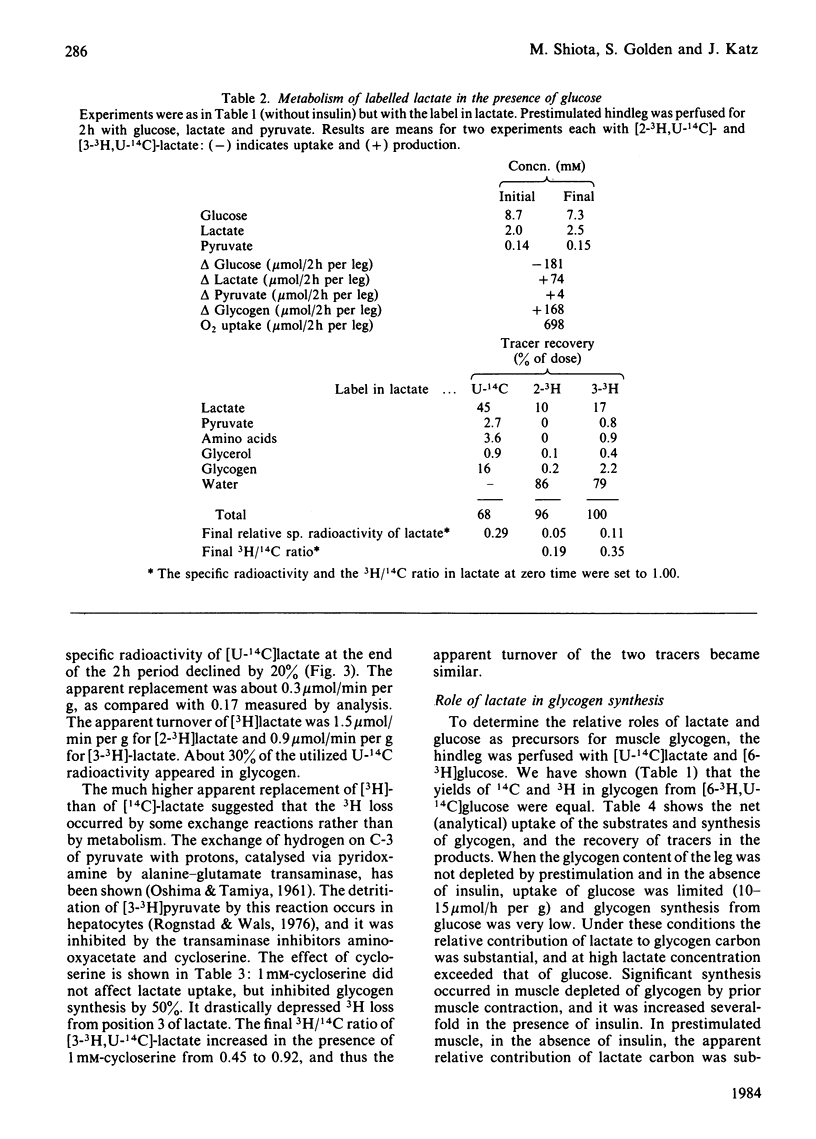
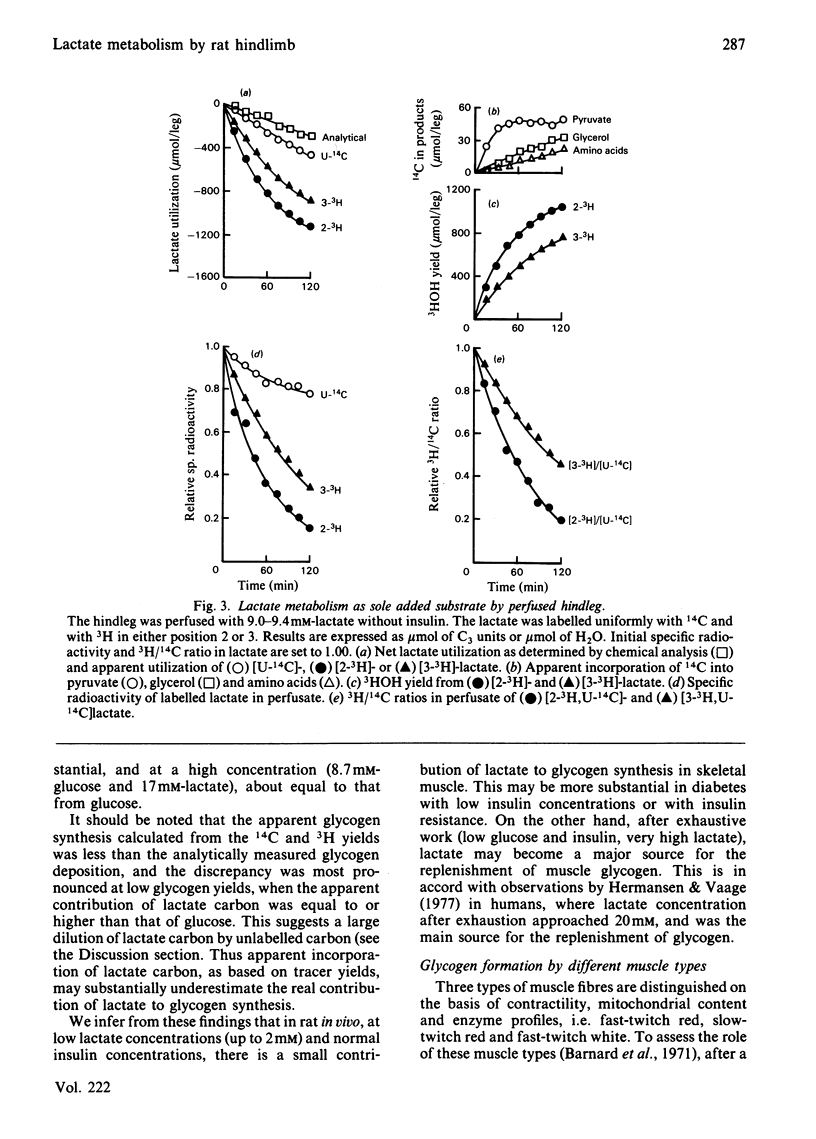
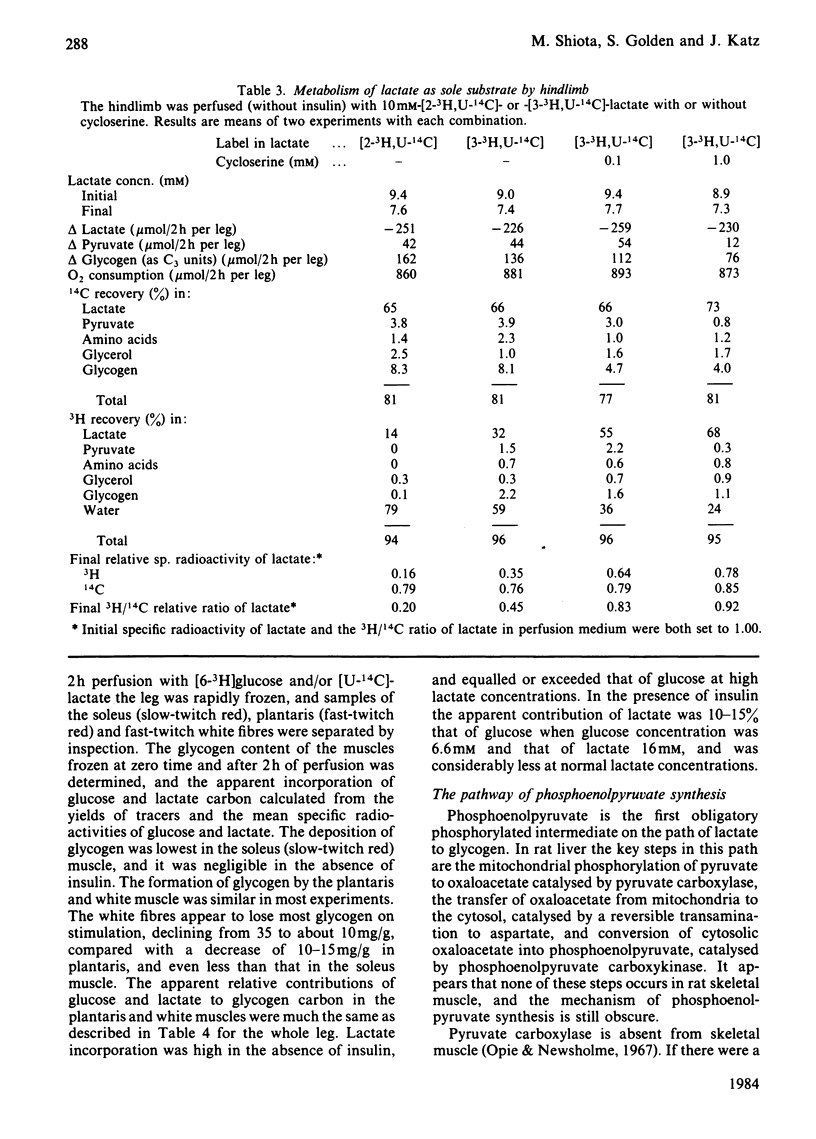
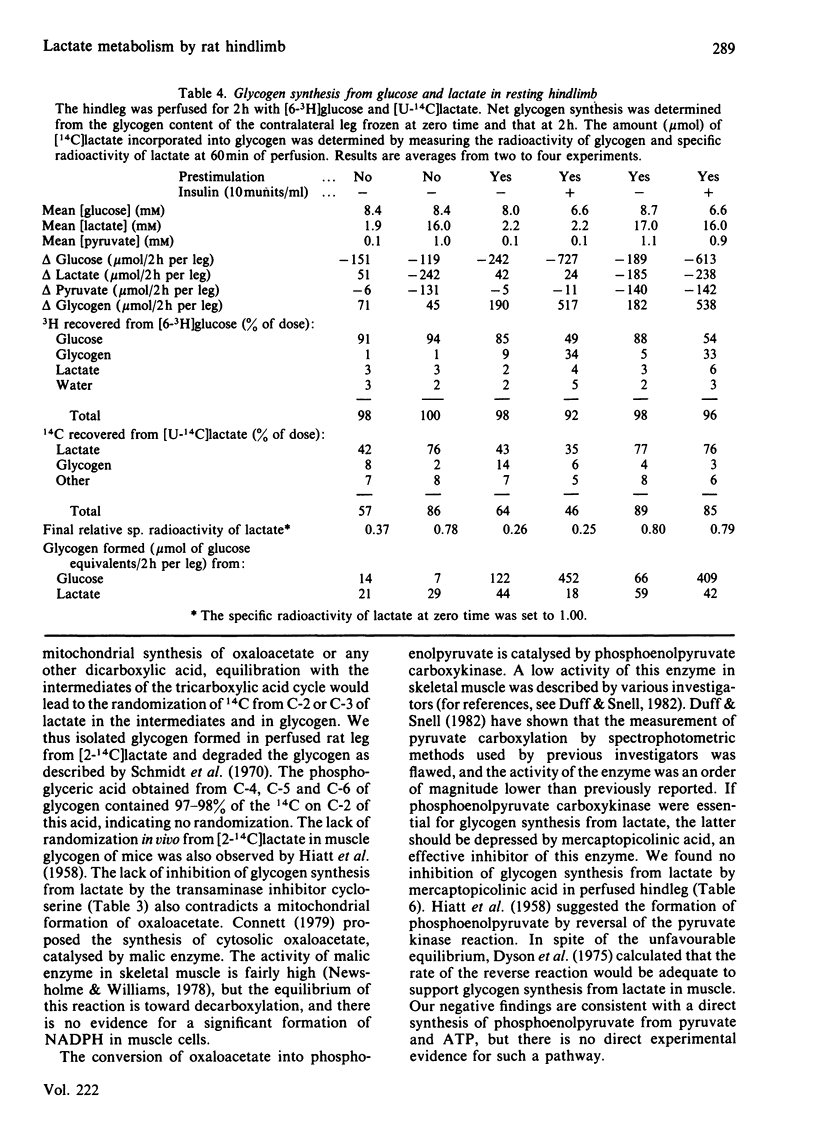
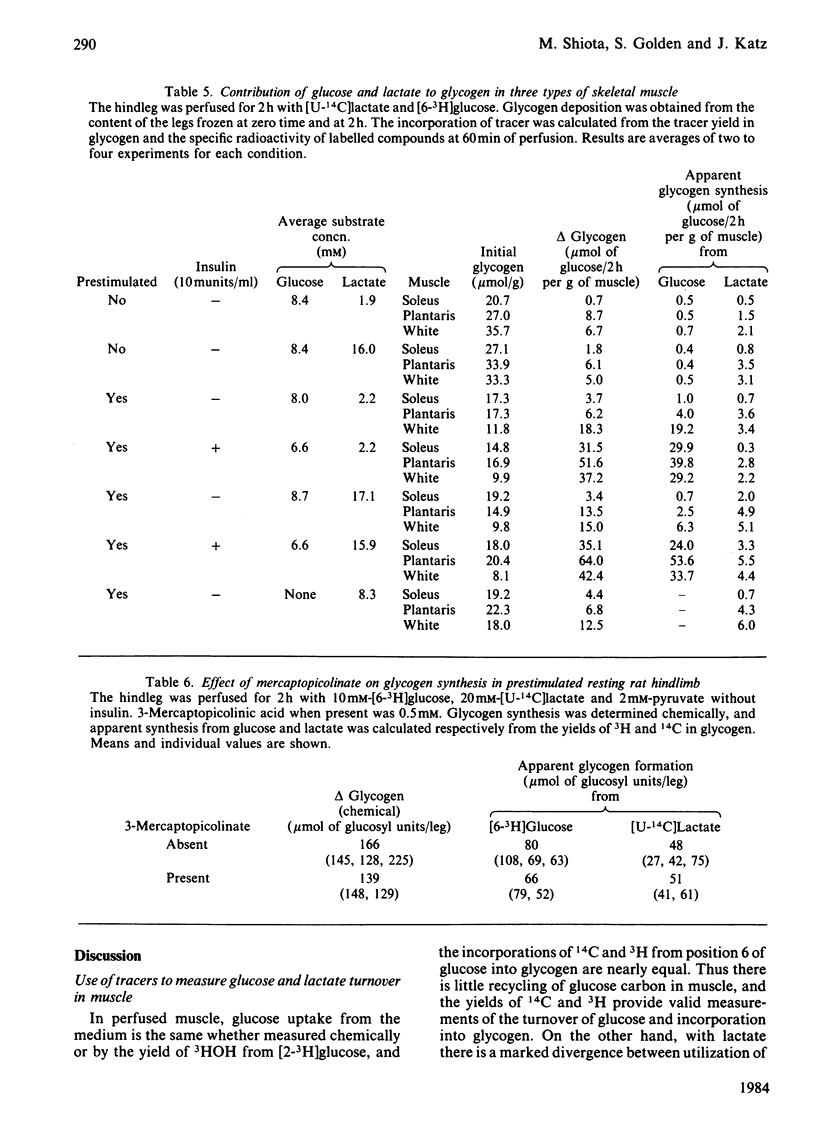
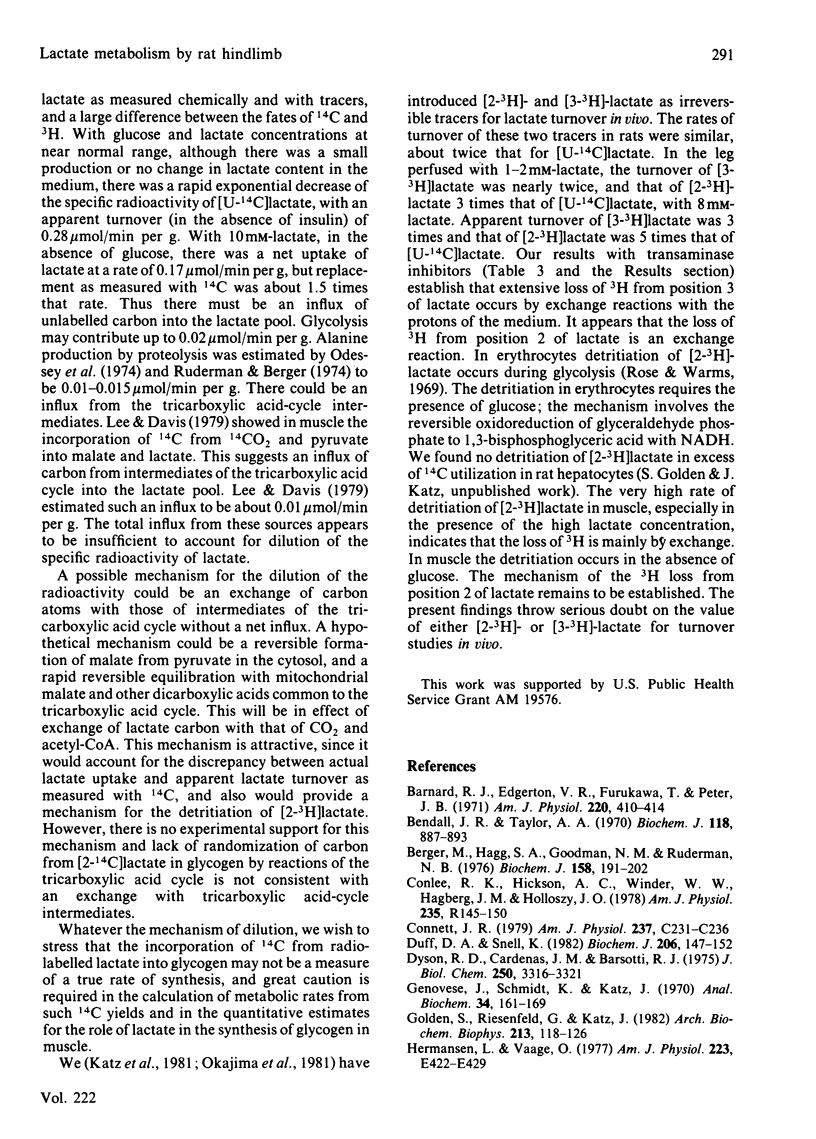
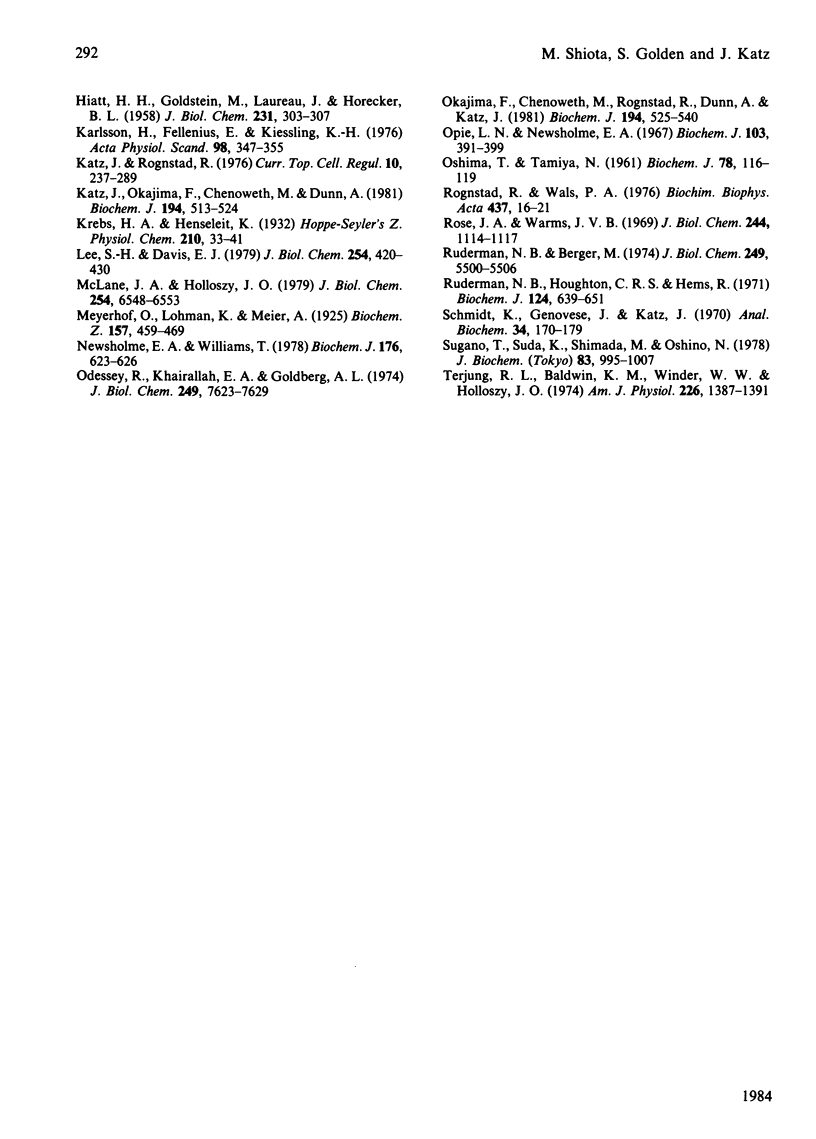
Selected References
These references are in PubMed. This may not be the complete list of references from this article.
- Barnard R. J., Edgerton V. R., Furukawa T., Peter J. B. Histochemical, biochemical, and contractile properties of red, white, and intermediate fibers. Am J Physiol. 1971 Feb;220(2):410–414. doi: 10.1152/ajplegacy.1971.220.2.410. [DOI] [PubMed] [Google Scholar]
- Bendall J. R., Taylor A. A. The Meyerhof quotient and the synthesis of glycogen from lactate in frog and rabbit muscle. Biochem J. 1970 Aug;118(5):887–893. doi: 10.1042/bj1180887. [DOI] [PMC free article] [PubMed] [Google Scholar]
- Berger M., Hagg S. A., Goodman M. N., Ruderman N. B. Glucose metabolism in perfused skeletal muscle. Effects of starvation, diabetes, fatty acids, acetoacetate, insulin and exercise on glucose uptake and disposition. Biochem J. 1976 Aug 15;158(2):191–202. doi: 10.1042/bj1580191. [DOI] [PMC free article] [PubMed] [Google Scholar]
- Conlee R. K., Hickson R. C., Winder W. W., Hagberg J. M., Holloszy J. O. Regulation of glycogen resynthesis in muscles of rats following exercise. Am J Physiol. 1978 Sep;235(3):R145–R150. doi: 10.1152/ajpregu.1978.235.3.R145. [DOI] [PubMed] [Google Scholar]
- Connett R. J. Glyconeogenesis from lactate in frog striated muscle. Am J Physiol. 1979 Nov;237(5):C231–C236. doi: 10.1152/ajpcell.1979.237.5.C231. [DOI] [PubMed] [Google Scholar]
- Duff D. A., Snell K. Limitations of commonly used spectrophotometric assay methods for phosphoenolypyruvate carboxykinase activity in crude extracts of muscle. Biochem J. 1982 Jul 15;206(1):147–152. doi: 10.1042/bj2060147. [DOI] [PMC free article] [PubMed] [Google Scholar]
- Dyson R. D., Cardenas J. M., Barsotti R. J. The reversibility of skeletal muscle pyruvate kinase and an assessment of its capacity to support glyconeogenesis. J Biol Chem. 1975 May 10;250(9):3316–3321. [PubMed] [Google Scholar]
- Genovese J., Schmidt K., Katz J. Enzymic degradation of isotopically labeled compounds. I. Degradation of 14C-labeled glycerol. Anal Biochem. 1970 Mar;34:161–169. doi: 10.1016/0003-2697(70)90097-7. [DOI] [PubMed] [Google Scholar]
- Golden S., Riesenfeld G., Katz J. Carbohydrate metabolism of hepatocytes from starved Japanese quail. Arch Biochem Biophys. 1982 Jan;213(1):118–126. doi: 10.1016/0003-9861(82)90446-5. [DOI] [PubMed] [Google Scholar]
- HIATT H. H., GOLDSTEIN M., LAREAU J., HORECKER B. L. The pathway of hexose synthesis from pyruvate in muscle. J Biol Chem. 1958 Mar;231(1):303–307. [PubMed] [Google Scholar]
- Hermansen L., Vaage O. Lactate disappearance and glycogen synthesis in human muscle after maximal exercise. Am J Physiol. 1977 Nov;233(5):E422–E429. doi: 10.1152/ajpendo.1977.233.5.E422. [DOI] [PubMed] [Google Scholar]
- Karlsson N., Fellenius E., Kiessling K. H. Influence of acetate on glucose metabolism in the perfused hind-quarter of the rat. Acta Physiol Scand. 1976 Nov;98(3):347–355. doi: 10.1111/j.1748-1716.1976.tb10319.x. [DOI] [PubMed] [Google Scholar]
- Katz J., Okajima F., Chenoweth M., Dunn A. The determination of lactate turnover in vivo with 3H- and 14C-labelled lactate. The significance of sites of tracer administration and sampling. Biochem J. 1981 Feb 15;194(2):513–524. doi: 10.1042/bj1940513. [DOI] [PMC free article] [PubMed] [Google Scholar]
- Katz J., Rognstad R. Futile cycles in the metabolism of glucose. Curr Top Cell Regul. 1976;10:237–289. doi: 10.1016/b978-0-12-152810-2.50013-9. [DOI] [PubMed] [Google Scholar]
- Lee S. H., Davis E. J. Carboxylation and decarboxylation reactions. Anaplerotic flux and removal of citrate cycle intermediates in skeletal muscle. J Biol Chem. 1979 Jan 25;254(2):420–430. [PubMed] [Google Scholar]
- McLane J. A., Holloszy J. O. Glycogen synthesis from lactate in the three types of skeletal muscle. J Biol Chem. 1979 Jul 25;254(14):6548–6553. [PubMed] [Google Scholar]
- Newsholme E. A., Williams T. The role of phosphoenolpyruvate carboxykinase in amino acid metabolism in muscle. Biochem J. 1978 Nov 15;176(2):623–626. doi: 10.1042/bj1760623. [DOI] [PMC free article] [PubMed] [Google Scholar]
- OSHIMA T., TAMIYA N. Mechanism of transaminase action. Biochem J. 1961 Jan;78:116–119. doi: 10.1042/bj0780116. [DOI] [PMC free article] [PubMed] [Google Scholar]
- Odessey R., Khairallah E. A., Goldberg A. L. Origin and possible significance of alanine production by skeletal muscle. J Biol Chem. 1974 Dec 10;249(23):7623–7629. [PubMed] [Google Scholar]
- Okajima F., Chenoweth M., Rognstad R., Dunn A., Katz J. Metabolism of 3H- and 14C-labelled lactate in starved rats. Biochem J. 1981 Feb 15;194(2):525–540. doi: 10.1042/bj1940525. [DOI] [PMC free article] [PubMed] [Google Scholar]
- Opie L. H., Newsholme E. A. The activities of fructose 1,6-diphosphatase, phosphofructokinase and phosphoenolpyruvate carboxykinase in white muscle and red muscle. Biochem J. 1967 May;103(2):391–399. doi: 10.1042/bj1030391. [DOI] [PMC free article] [PubMed] [Google Scholar]
- Rognstad R., Wals P. The metabolism of l-[3-3h]lactate by isolated hamster liver cells. Biochim Biophys Acta. 1976 Jun 23;437(1):16–21. doi: 10.1016/0304-4165(76)90343-3. [DOI] [PubMed] [Google Scholar]
- Rose I. A., Warms J. V. Glycolysis-dependent exchange of diphosphopyridine nucleotide-3H in red blood cells and ascites cells. J Biol Chem. 1969 Mar 10;244(5):1114–1117. [PubMed] [Google Scholar]
- Ruderman N. B., Berger M. The formation of glutamine and alanine in skeletal muscle. J Biol Chem. 1974 Sep 10;249(17):5500–5506. [PubMed] [Google Scholar]
- Ruderman N. B., Houghton C. R., Hems R. Evaluation of the isolated perfused rat hindquarter for the study of muscle metabolism. Biochem J. 1971 Sep;124(3):639–651. doi: 10.1042/bj1240639. [DOI] [PMC free article] [PubMed] [Google Scholar]
- Schmidt K., Genovese J., Katz J. Enzymic degradation of isotopically labeded compounds. II. Glucose labeled with 14C and tritium. Anal Biochem. 1970 Mar;34:170–179. doi: 10.1016/0003-2697(70)90098-9. [DOI] [PubMed] [Google Scholar]
- Sugano T., Suda K., Shimada M., Oshino N. Biochemical and ultrastructural evaluation of isolated rat liver systems perfused with a hemoglobin-free medium. J Biochem. 1978 Apr;83(4):995–1007. doi: 10.1093/oxfordjournals.jbchem.a132028. [DOI] [PubMed] [Google Scholar]
- Terjung R. L., Baldwin K. M., Winder W. W., Holloszy J. O. Glycogen repletion in different types of muscle and in liver after exhausting exercise. Am J Physiol. 1974 Jun;226(6):1387–1391. doi: 10.1152/ajplegacy.1974.226.6.1387. [DOI] [PubMed] [Google Scholar]