Abstract
The kinetics of assembly were studied for bovine and pig microtubule protein in vitro over a range of conditions of pH, temperature, nucleotide and protein concentration. The kinetics are in general biphasic with two major processes of similar amplitude but separated in rate by one order of magnitude. Rates and amplitudes are complex functions of solution conditions. The rates of the fast phase and the slow phase attain limiting values as a function of increasing protein concentration, and are more stringently limited at pH 6.5 than pH 6.95. Such behaviour indicates that mechanisms other than the condensation polymerization of tubulin dimer become rate-limiting at higher protein concentration. The constancy of the wavelength-dependence of light-scattering and ultrastructural criteria indicate that microtubules of normal morphology are formed in both phases of the assembly process. Electrophoretic analysis of assembling microtubule protein shows that MAP- (microtubule-associated-protein-)rich microtubules are formed during the fast phase. The rate of dissociation of oligomeric species on dilution of microtubule protein closely parallels the fast-phase rate in magnitude and temperature-dependence. We propose that the rate of this process constitutes an upper limit to the rate of the fast phase of assembly. The kinetics of redistribution of MAPs from MAP-rich microtubules may be a factor limiting the slow-phase rate. A working model is derived for the self-assembly of microtubule protein incorporating the dissociation and redistribution mechanisms that impose upper limits to the rates of assembly attainable by bimolecular addition reactions. Key roles are assigned to MAP-containing fragments in both phases of microtubule elongation. Variations in kinetic behaviour with solution conditions are inferred to derive from the nature and properties of fragments formed from oligomeric species after the rapid temperature jump. The model accounts for the limiting rate behaviour and indicates experimental criteria to be applied in evaluating the relative contributions of alternative pathways.
Full text
PDF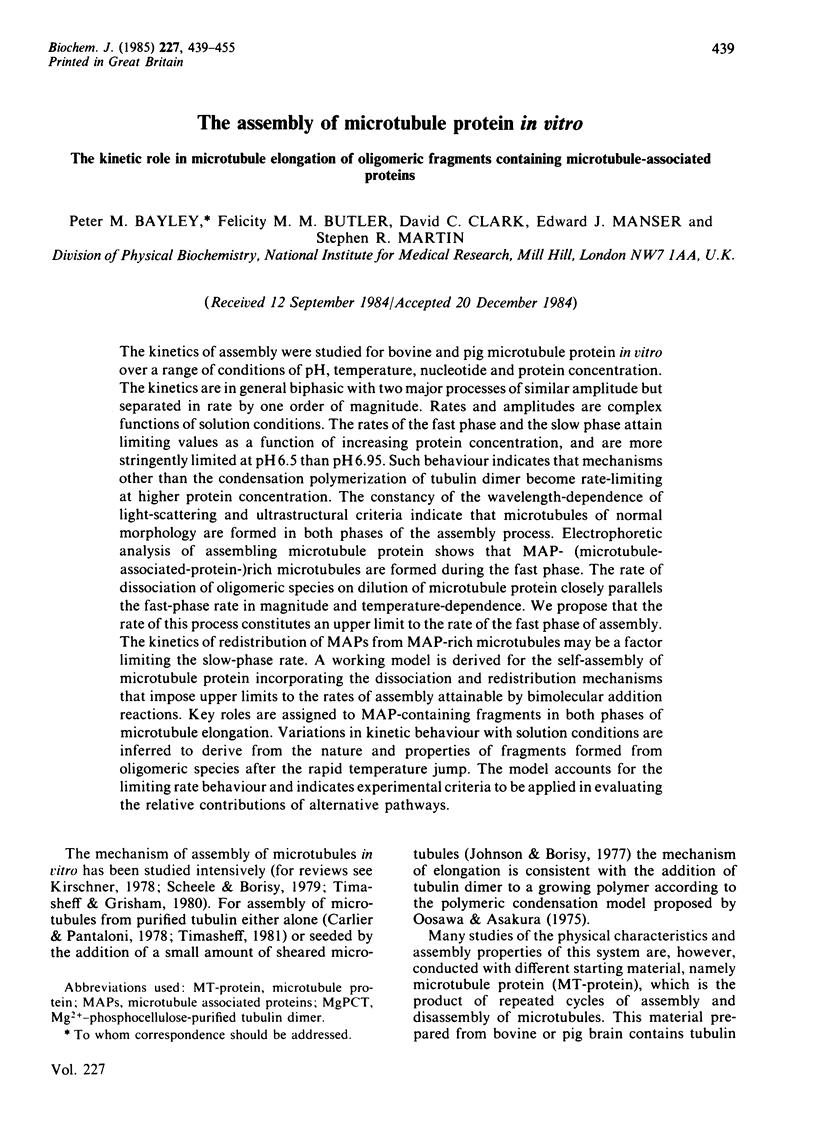
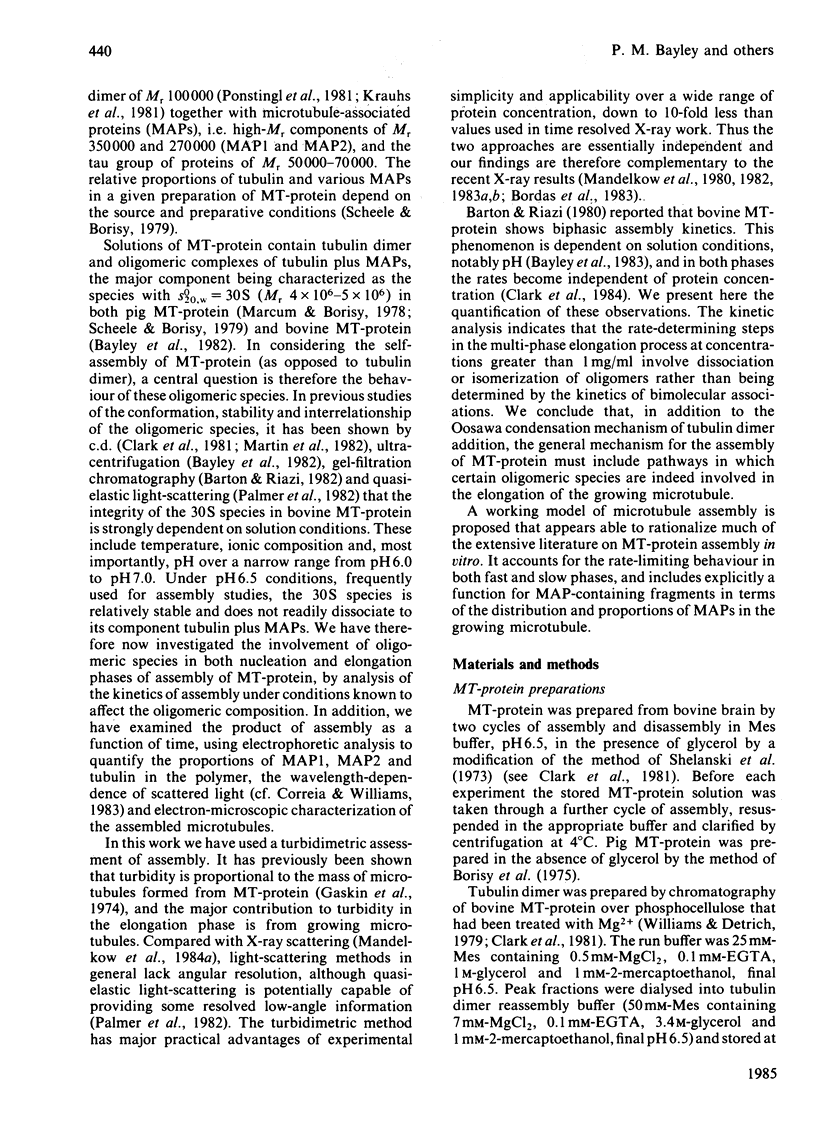
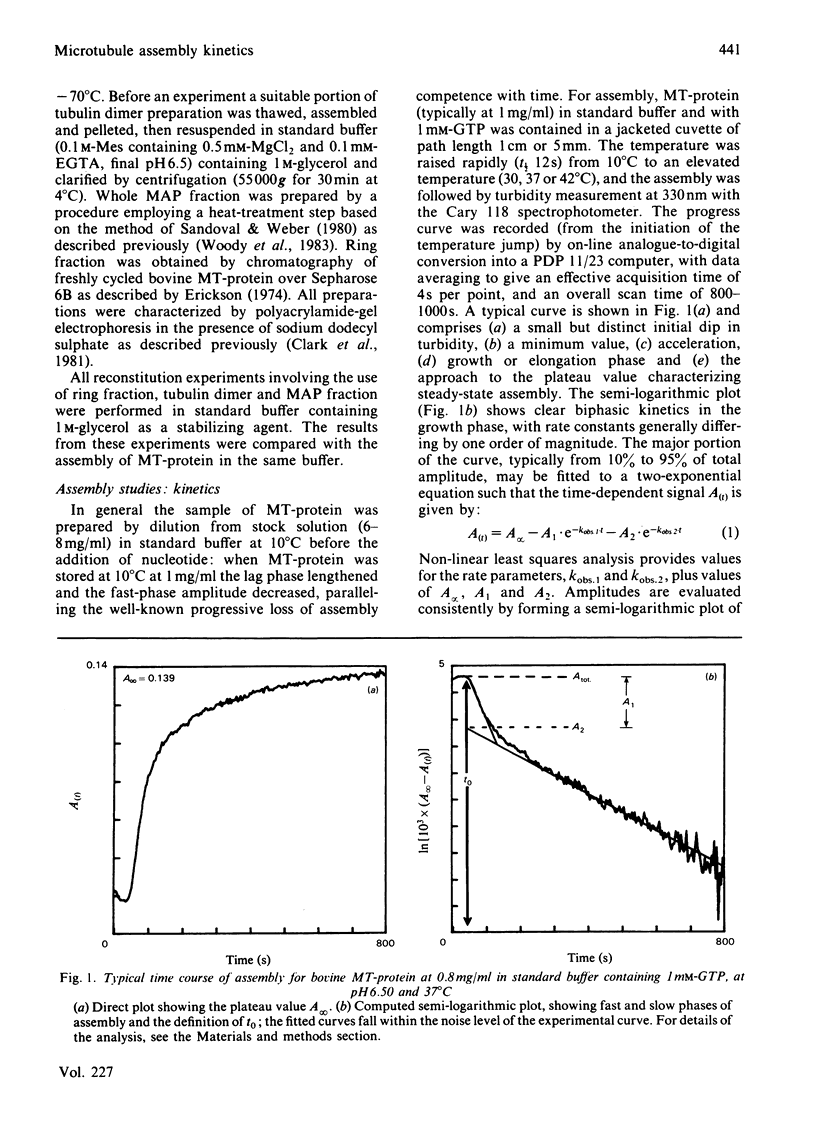
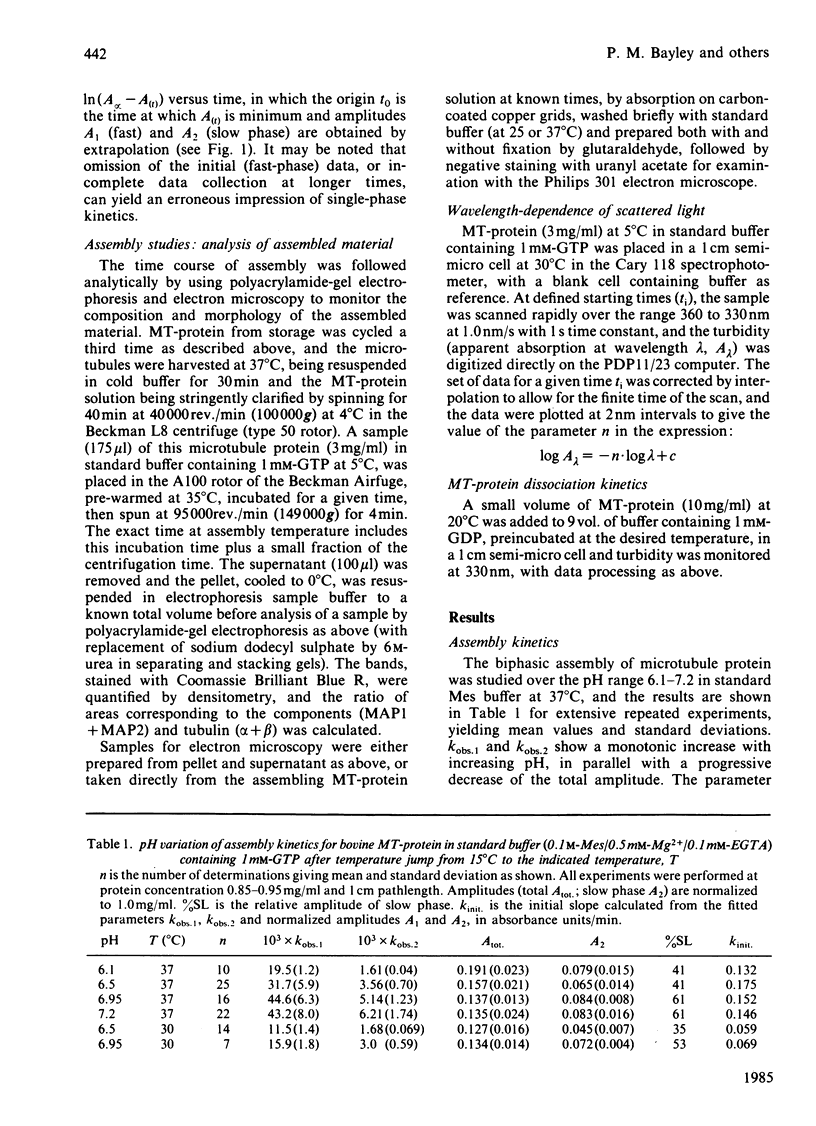
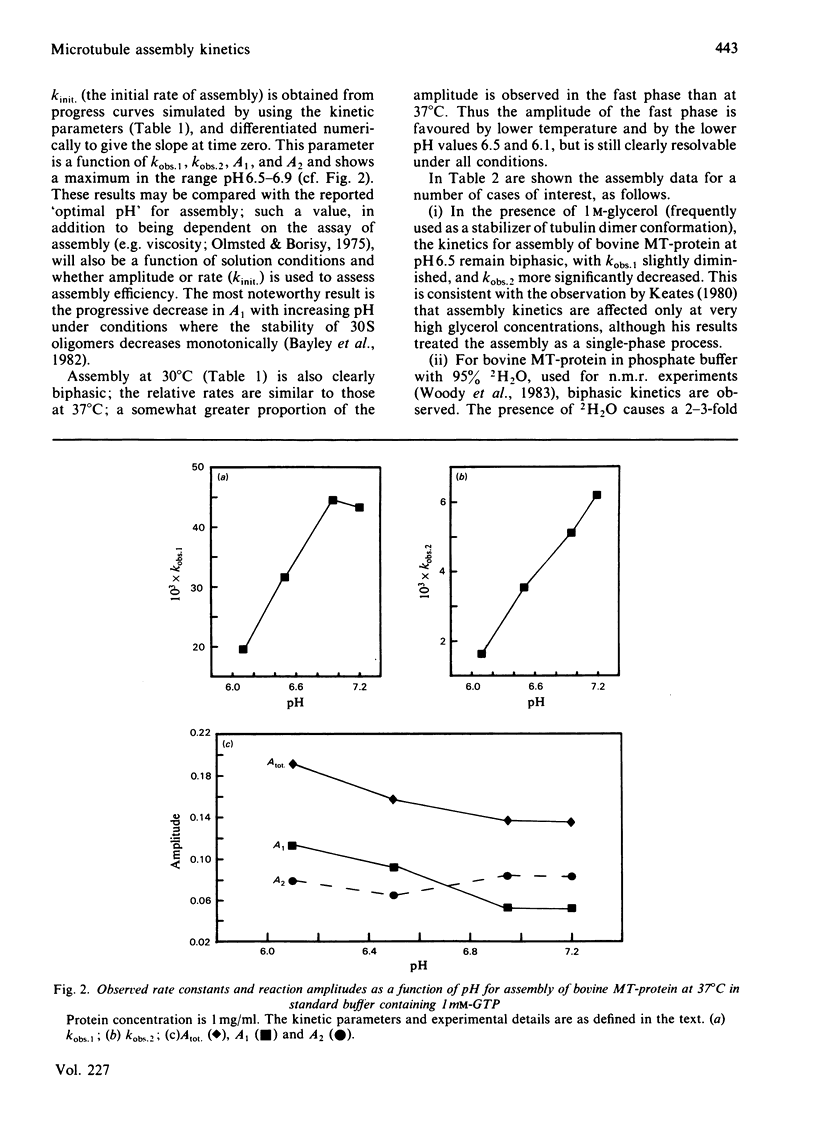
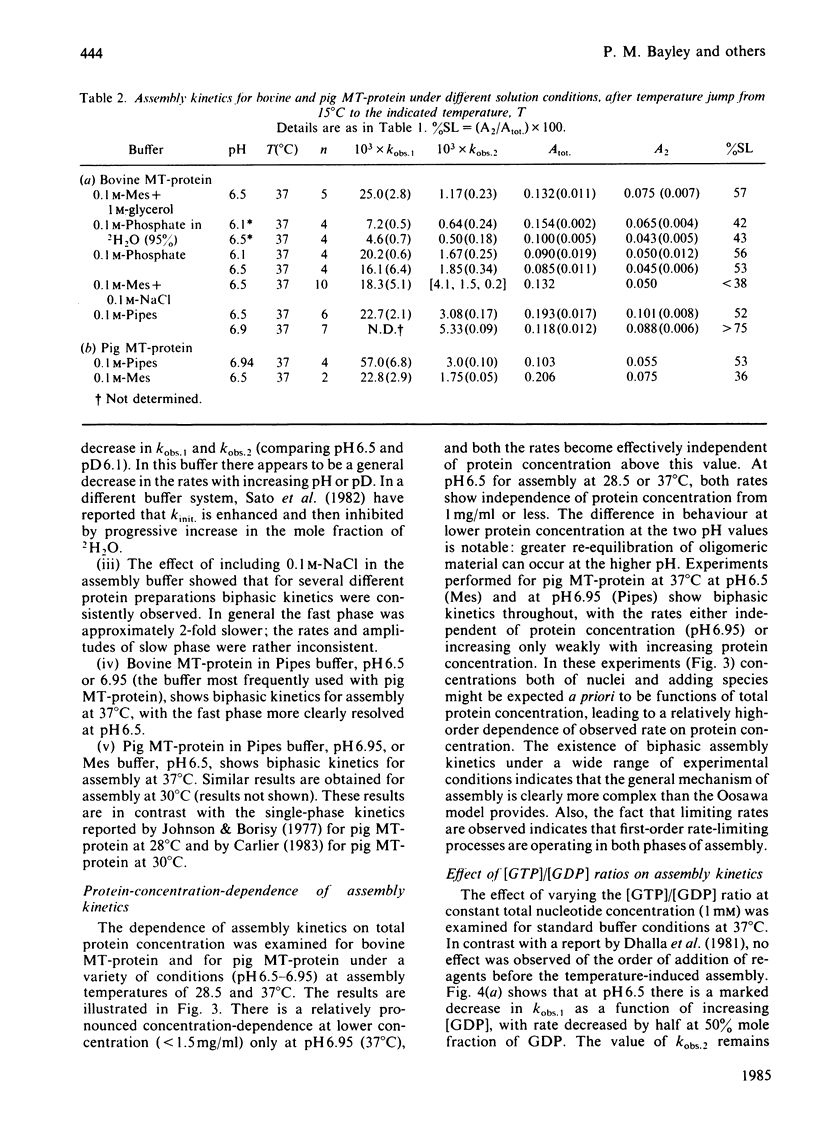
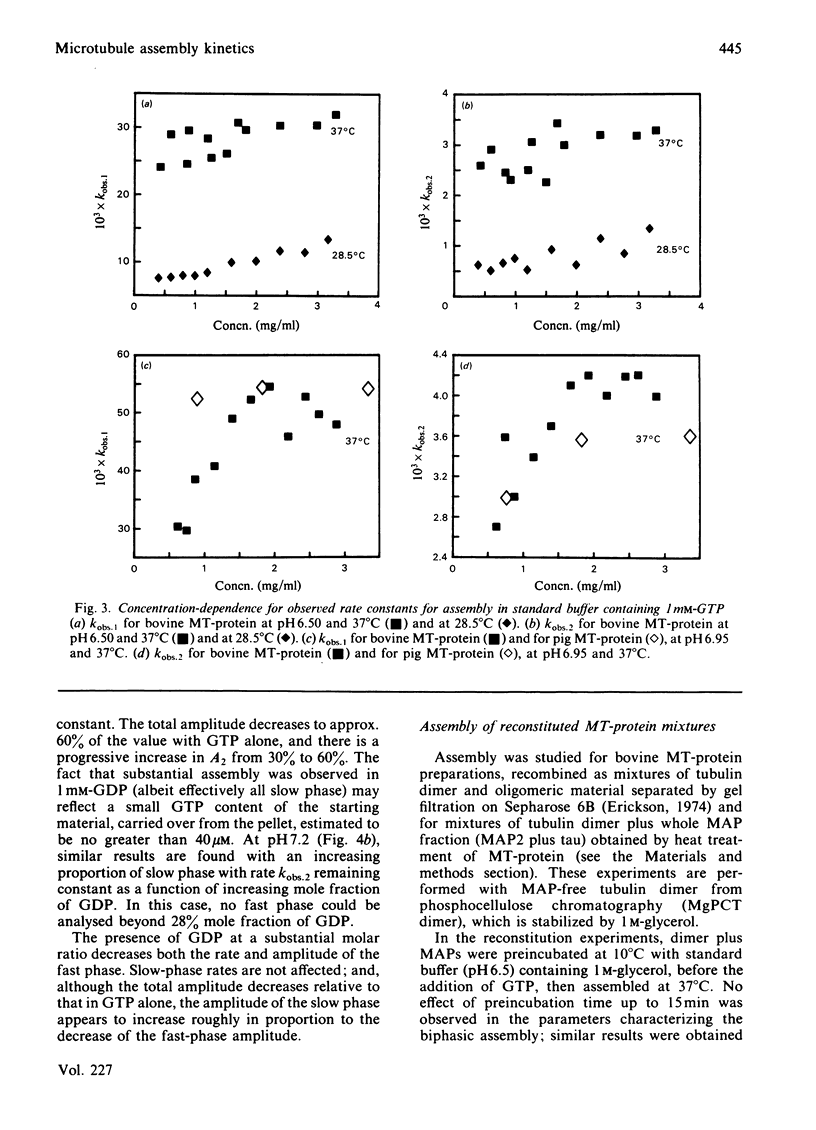
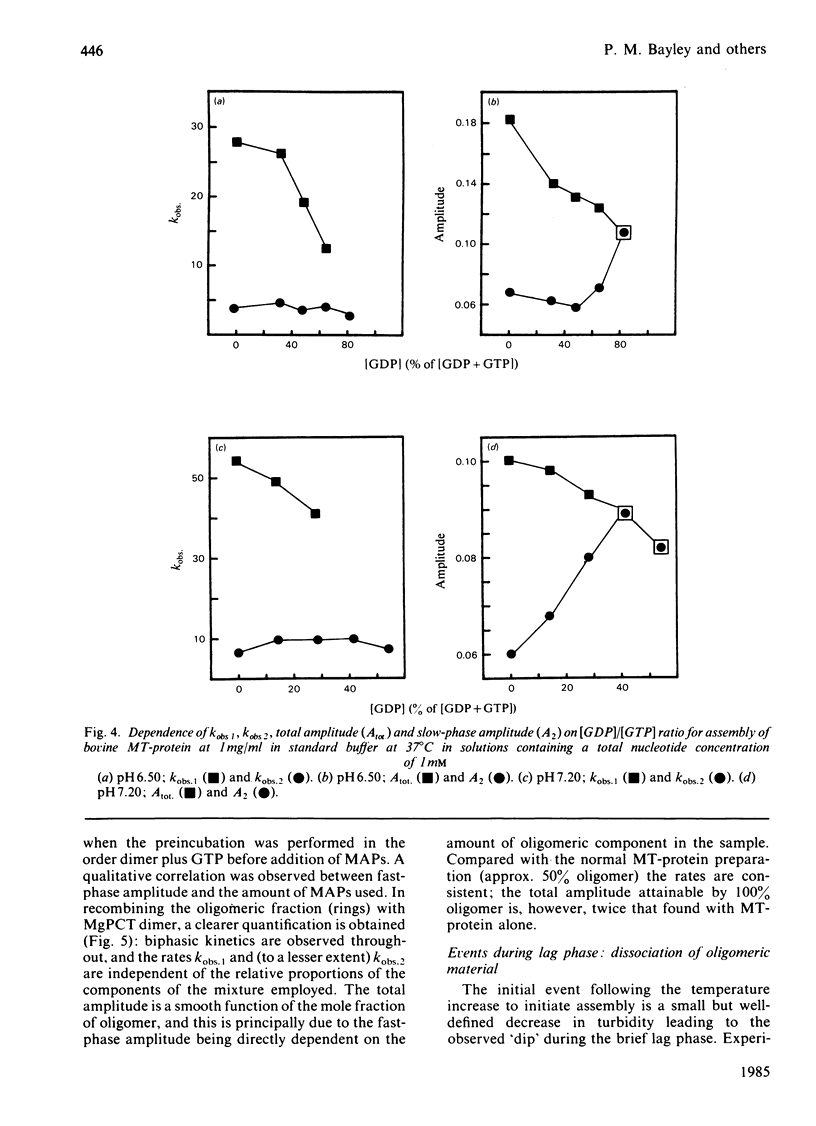
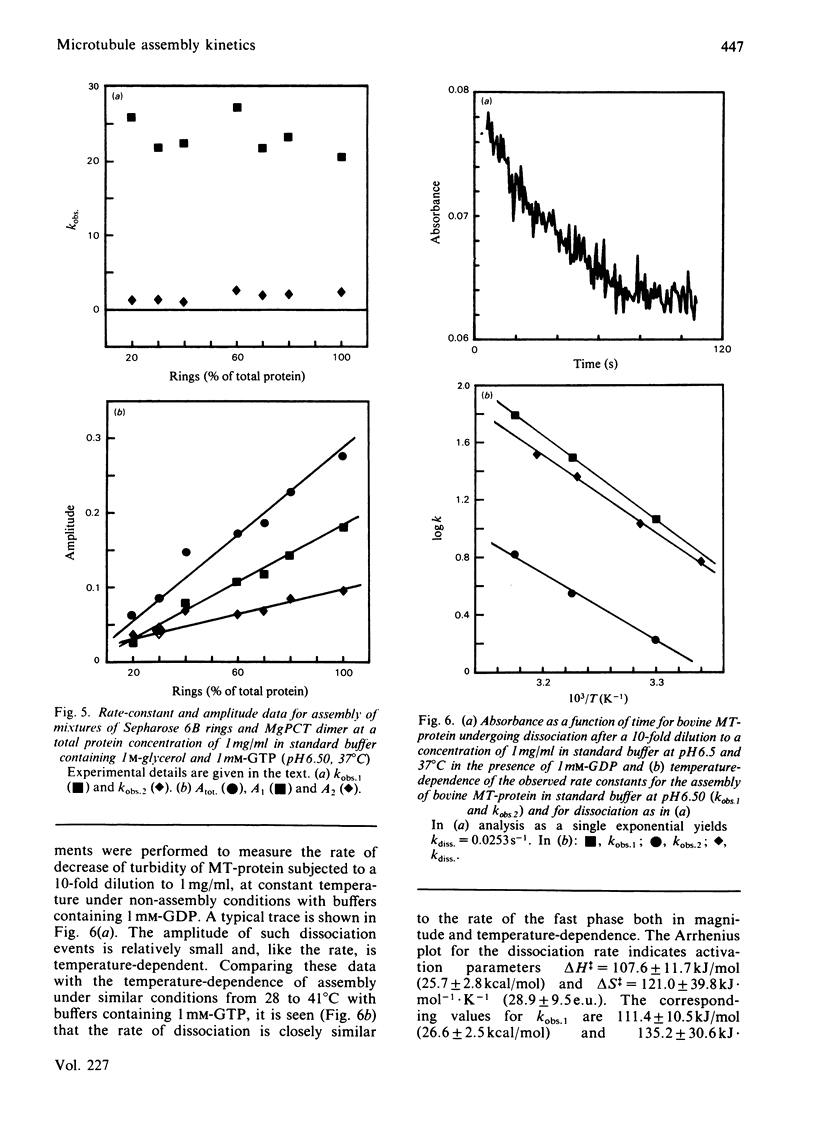
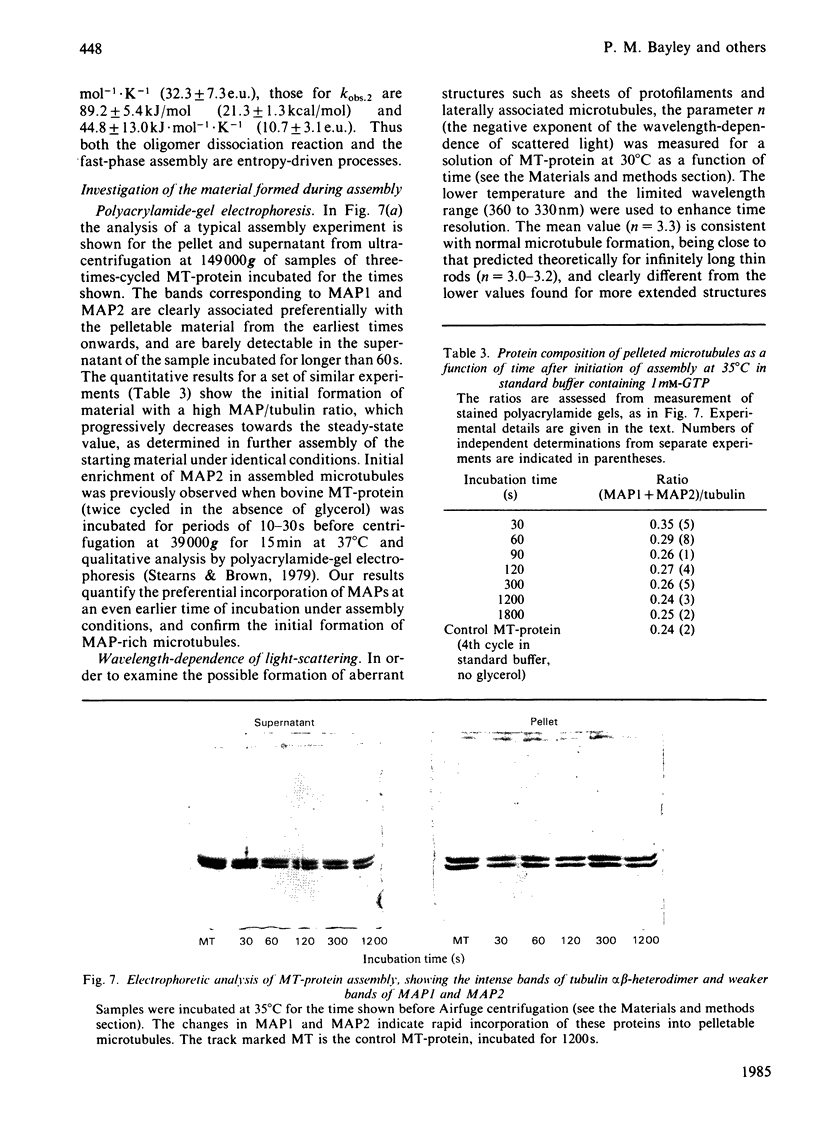
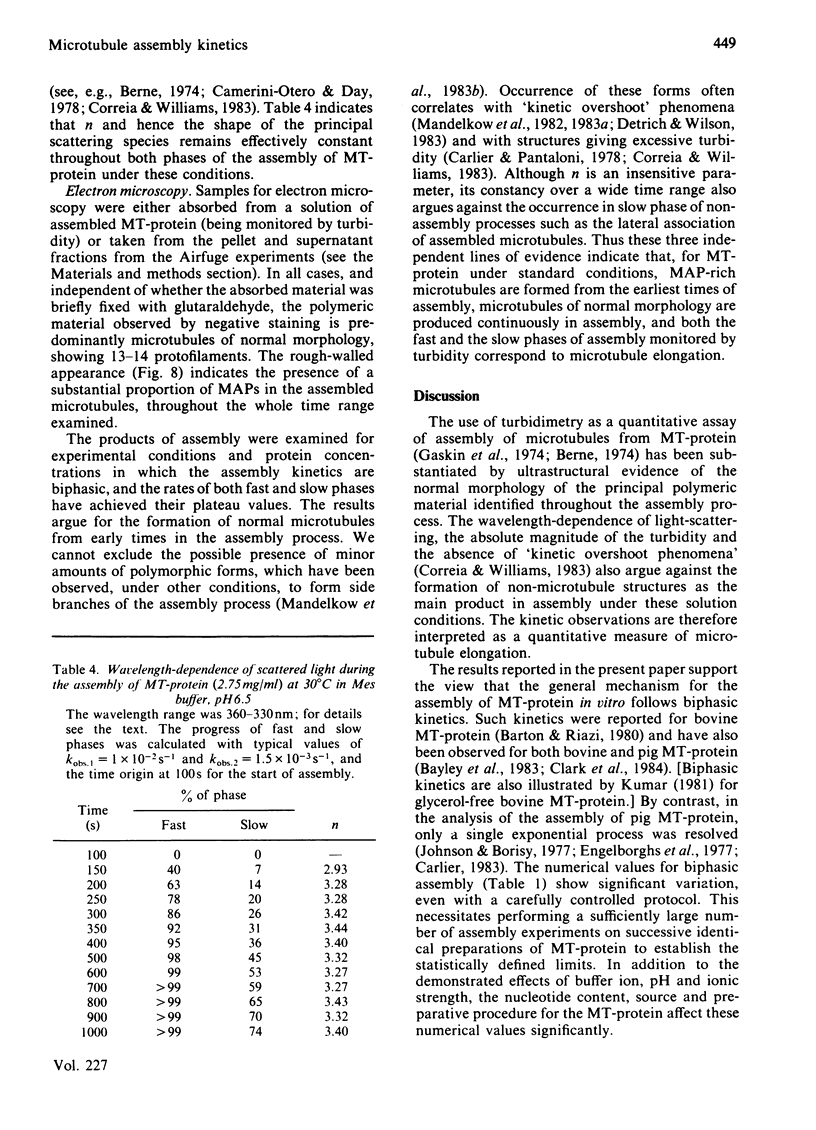
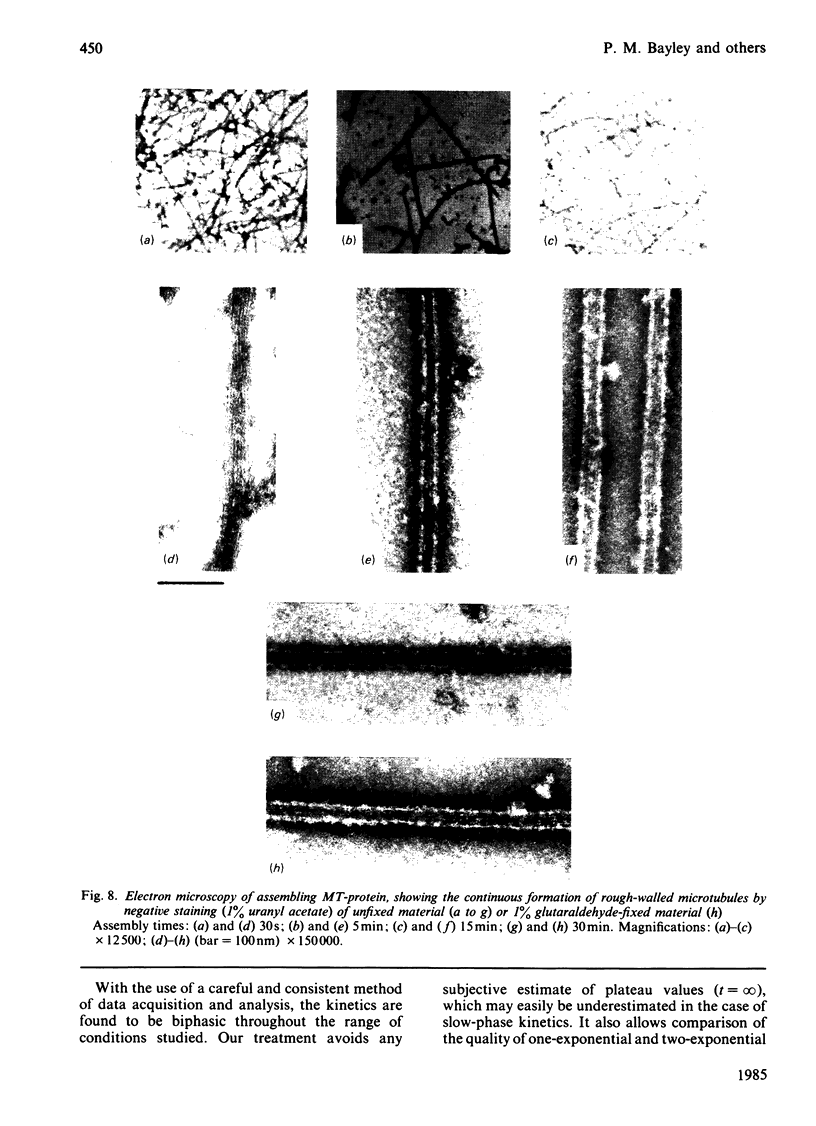
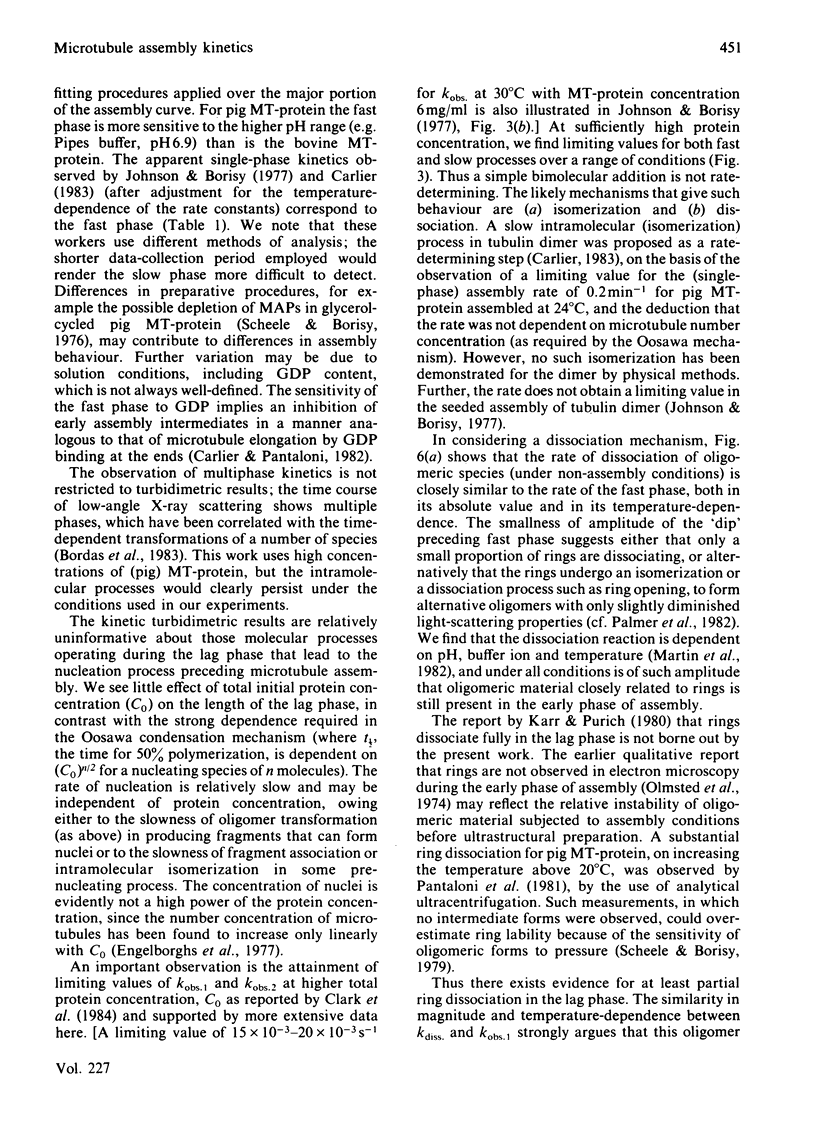
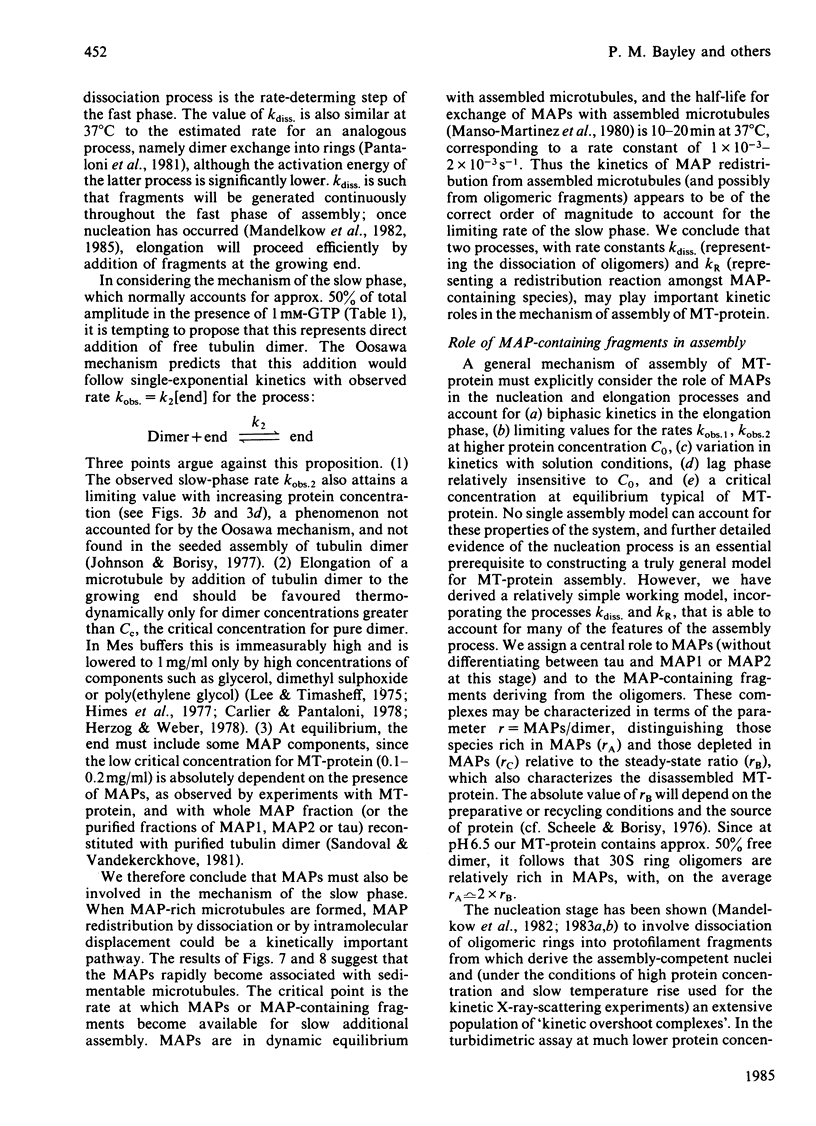
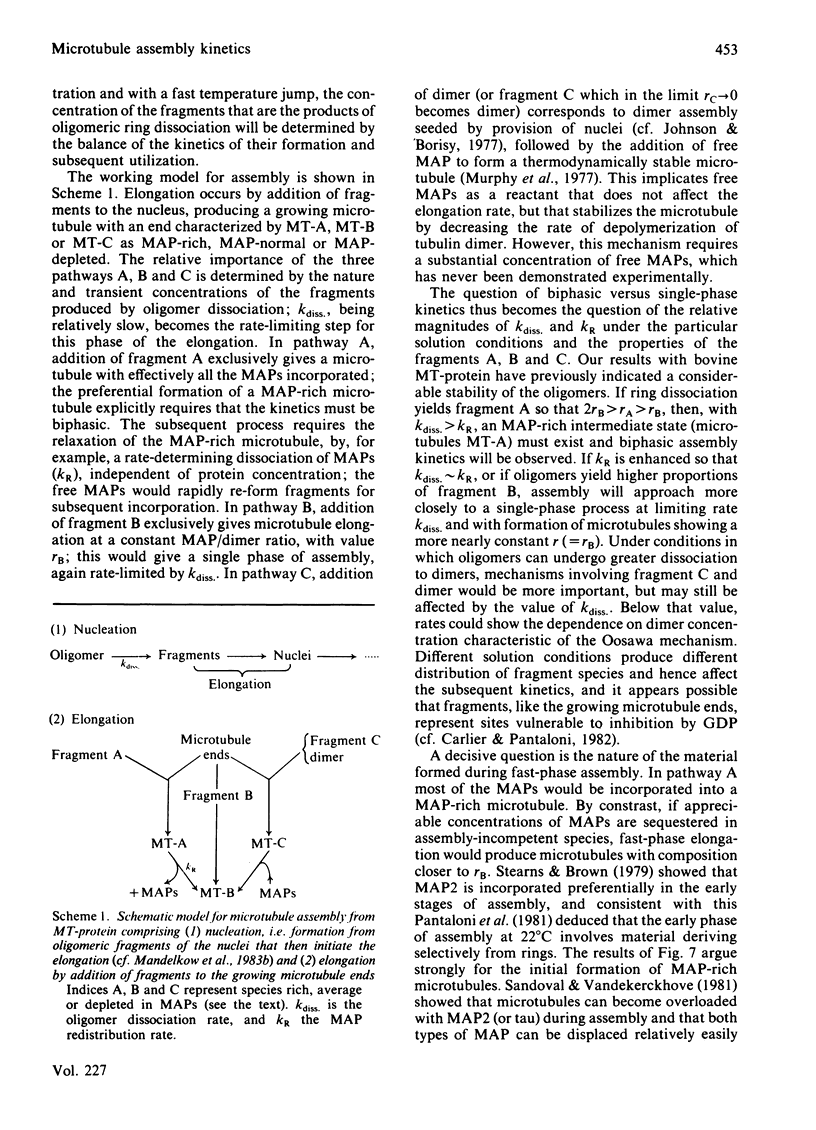
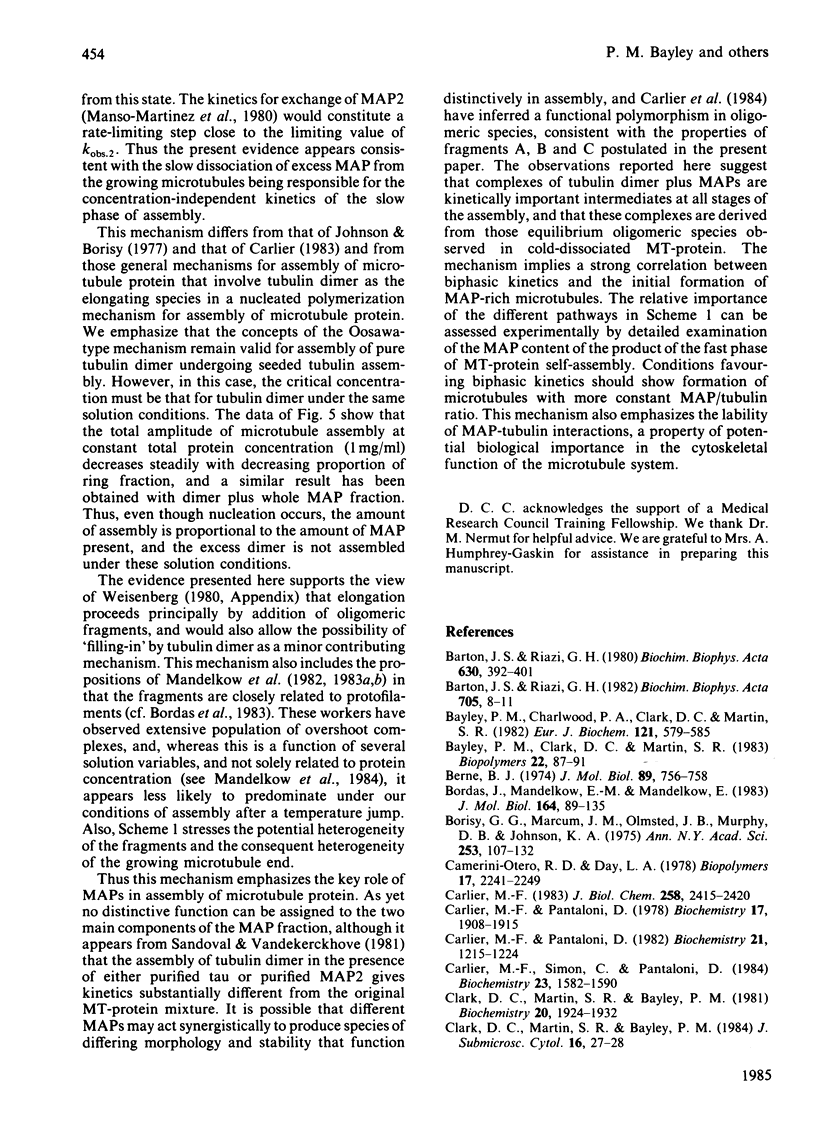
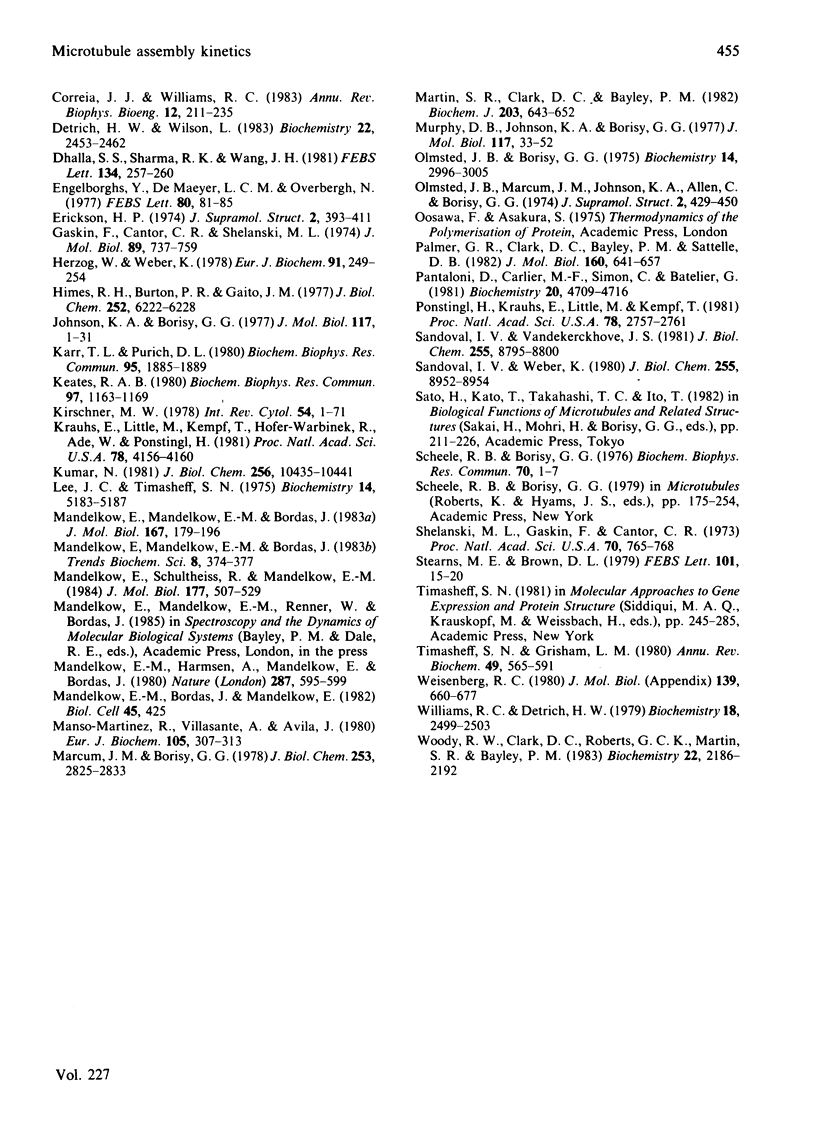
Images in this article
Selected References
These references are in PubMed. This may not be the complete list of references from this article.
- Barton J. S., Riazi G. H. Evidence against tubulin oligomer dissociation to tubulin dimer at assembly temperatures. Biochim Biophys Acta. 1982 Jul 12;705(1):8–11. doi: 10.1016/0167-4838(82)90328-4. [DOI] [PubMed] [Google Scholar]
- Barton J. S., Riazi G. H. Evidence for two growth steps in microtubule polymerization. Biochim Biophys Acta. 1980 Jul 3;630(3):392–401. doi: 10.1016/0304-4165(80)90288-3. [DOI] [PubMed] [Google Scholar]
- Bayley P. M., Charlwood P. A., Clark D. C., Martin S. R. Oligomeric species in glycerol-cycled bovine-brain microtubule protein. Analytical ultracentrifugal characterisation. Eur J Biochem. 1982 Jan;121(3):579–585. doi: 10.1111/j.1432-1033.1982.tb05826.x. [DOI] [PubMed] [Google Scholar]
- Bayley P. M., Clark D. C., Martin S. R. Conformational properties of microtubule protein: their relation to the self-assembly process in vitro. Biopolymers. 1983 Jan;22(1):87–91. doi: 10.1002/bip.360220114. [DOI] [PubMed] [Google Scholar]
- Berne B. J. Interpretation of the light scattering from long rods. J Mol Biol. 1974 Nov 15;89(4):755–758. doi: 10.1016/0022-2836(74)90049-7. [DOI] [PubMed] [Google Scholar]
- Bordas J., Mandelkow E. M., Mandelkow E. Stages of tubulin assembly and disassembly studied by time-resolved synchrotron X-ray scattering. J Mol Biol. 1983 Feb 15;164(1):89–135. doi: 10.1016/0022-2836(83)90089-x. [DOI] [PubMed] [Google Scholar]
- Borisy G. G., Marcum J. M., Olmsted J. B., Murphy D. B., Johnson K. A. Purification of tubulin and associated high molecular weight proteins from porcine brain and characterization of microtubule assembly in vitro. Ann N Y Acad Sci. 1975 Jun 30;253:107–132. doi: 10.1111/j.1749-6632.1975.tb19196.x. [DOI] [PubMed] [Google Scholar]
- Carlier M. F. Kinetic evidence for a conformation change of tubulin preceding microtubule assembly. J Biol Chem. 1983 Feb 25;258(4):2415–2420. [PubMed] [Google Scholar]
- Carlier M. F., Pantaloni D. Assembly of microtubule protein: role of guanosine di- and triphosphate nucleotides. Biochemistry. 1982 Mar 16;21(6):1215–1224. doi: 10.1021/bi00535a017. [DOI] [PubMed] [Google Scholar]
- Carlier M. F., Pantaloni D. Kinetic analysis of cooperativity in tubulin polymerization in the presence of guanosine di- or triphosphate nucleotides. Biochemistry. 1978 May 16;17(10):1908–1915. doi: 10.1021/bi00603a017. [DOI] [PubMed] [Google Scholar]
- Carlier M. F., Simon C., Pantaloni D. Polymorphism of tubulin oligomers in the presence of microtubule-associated proteins. Implications in microtubule assembly. Biochemistry. 1984 Mar 27;23(7):1582–1590. doi: 10.1021/bi00302a037. [DOI] [PubMed] [Google Scholar]
- Clark D. C., Martin S. R., Bayley P. M. Conformation and assembly characteristics of tubulin and microtubule protein from bovine brain. Biochemistry. 1981 Mar 31;20(7):1924–1932. doi: 10.1021/bi00510a031. [DOI] [PubMed] [Google Scholar]
- Correia J. J., Williams R. C., Jr Mechanisms of assembly and disassembly of microtubules. Annu Rev Biophys Bioeng. 1983;12:211–235. doi: 10.1146/annurev.bb.12.060183.001235. [DOI] [PubMed] [Google Scholar]
- Detrich H. W., 3rd, Wilson L. Purification, characterization, and assembly properties of tubulin from unfertilized eggs of the sea urchin Strongylocentrotus purpuratus. Biochemistry. 1983 May 10;22(10):2453–2462. doi: 10.1021/bi00279a023. [DOI] [PubMed] [Google Scholar]
- Dhalla S. S., Sharma R. K., Wang J. H. Dependence of the order of addition of MAPs and GTP for microtubule assembly. FEBS Lett. 1981 Nov 16;134(2):257–260. doi: 10.1016/0014-5793(81)80614-x. [DOI] [PubMed] [Google Scholar]
- Engelborghs Y., De Maeyer L. C., Overbergh N. A kinetic analysis of the assembly of microtubules in vitro. FEBS Lett. 1977 Aug 1;80(1):81–85. doi: 10.1016/0014-5793(77)80411-0. [DOI] [PubMed] [Google Scholar]
- Erickson H. P. Assembly of microtubules from preformed, ring-shaped protofilaments and 6-S tubulin. J Supramol Struct. 1974;2(2-4):393–411. doi: 10.1002/jss.400020228. [DOI] [PubMed] [Google Scholar]
- Gaskin F., Cantor C. R., Shelanski M. L. Turbidimetric studies of the in vitro assembly and disassembly of porcine neurotubules. J Mol Biol. 1974 Nov 15;89(4):737–755. doi: 10.1016/0022-2836(74)90048-5. [DOI] [PubMed] [Google Scholar]
- Herzog W., Weber K. Microtubule formation by pure brain tubulin in vitro. The influence of dextran and poly(ethylene glycol). Eur J Biochem. 1978 Nov 2;91(1):249–254. doi: 10.1111/j.1432-1033.1978.tb20959.x. [DOI] [PubMed] [Google Scholar]
- Himes R. H., Burton P. R., Gaito J. M. Dimethyl sulfoxide-induced self-assembly of tubulin lacking associated proteins. J Biol Chem. 1977 Sep 10;252(17):6222–6228. [PubMed] [Google Scholar]
- Johnson K. A., Borisy G. G. Kinetic analysis of microtubule self-assembly in vitro. J Mol Biol. 1977 Nov 25;117(1):1–31. doi: 10.1016/0022-2836(77)90020-1. [DOI] [PubMed] [Google Scholar]
- Karr T. L., Purich D. L. Rings are not microtubule assembly intermediates: an analysis of the lag phase in GTP-dependent self-assembly of bovine brain tubulin. Biochem Biophys Res Commun. 1980 Aug 29;95(4):1885–1889. doi: 10.1016/s0006-291x(80)80119-7. [DOI] [PubMed] [Google Scholar]
- Keates R. A. Effects of glycerol on microtubule polymerization kinetics. Biochem Biophys Res Commun. 1980 Dec 16;97(3):1163–1169. doi: 10.1016/0006-291x(80)91497-7. [DOI] [PubMed] [Google Scholar]
- Kirschner M. W. Microtubule assembly and nucleation. Int Rev Cytol. 1978;54:1–71. doi: 10.1016/s0074-7696(08)60164-3. [DOI] [PubMed] [Google Scholar]
- Krauhs E., Little M., Kempf T., Hofer-Warbinek R., Ade W., Ponstingl H. Complete amino acid sequence of beta-tubulin from porcine brain. Proc Natl Acad Sci U S A. 1981 Jul;78(7):4156–4160. doi: 10.1073/pnas.78.7.4156. [DOI] [PMC free article] [PubMed] [Google Scholar]
- Kumar N. Taxol-induced polymerization of purified tubulin. Mechanism of action. J Biol Chem. 1981 Oct 25;256(20):10435–10441. [PubMed] [Google Scholar]
- Lee J. C., Timasheff S. N. The reconstitution of microtubules from purified calf brain tubulin. Biochemistry. 1975 Nov 18;14(23):5183–5187. doi: 10.1021/bi00694a025. [DOI] [PubMed] [Google Scholar]
- Mandelkow E. M., Harmsen A., Mandelkow E., Bordas J. X-ray kinetic studies of microtubule assembly using synchrotron radiation. Nature. 1980 Oct 16;287(5783):595–599. doi: 10.1038/287595a0. [DOI] [PubMed] [Google Scholar]
- Mandelkow E., Mandelkow E. M., Bordas J. Structure of tubulin rings studied by X-ray scattering using synchrotron radiation. J Mol Biol. 1983 Jun 15;167(1):179–196. doi: 10.1016/s0022-2836(83)80040-0. [DOI] [PubMed] [Google Scholar]
- Mandelkow E., Schultheiss R., Mandelkow E. M. Assembly and three-dimensional image reconstruction of tubulin hoops. J Mol Biol. 1984 Aug 15;177(3):507–529. doi: 10.1016/0022-2836(84)90297-3. [DOI] [PubMed] [Google Scholar]
- Manso-Martínez R., Villasante A., Avila J. Incorporation of the high-molecular-weight microtubule-associated protein 2 (MAP2) into microtubules at steady state in vitro. Eur J Biochem. 1980 Apr;105(2):307–313. doi: 10.1111/j.1432-1033.1980.tb04502.x. [DOI] [PubMed] [Google Scholar]
- Marcum J. M., Borisy G. G. Characterization of microtubule protein oligomers by analytical ultracentrifugation. J Biol Chem. 1978 Apr 25;253(8):2825–2833. [PubMed] [Google Scholar]
- Martin S. R., Clark D. C., Mayley P. M. Interactions of tubulin and microtubule-associated proteins. Conformation and stability of the oligomeric species from glycerol-cycled microtubule protein of bovine brain. Biochem J. 1982 Jun 1;203(3):643–652. doi: 10.1042/bj2030643. [DOI] [PMC free article] [PubMed] [Google Scholar]
- Murphy D. B., Johnson K. A., Borisy G. G. Role of tubulin-associated proteins in microtubule nucleation and elongation. J Mol Biol. 1977 Nov 25;117(1):33–52. doi: 10.1016/0022-2836(77)90021-3. [DOI] [PubMed] [Google Scholar]
- Olmsted J. B., Borisy G. G. Ionic and nucleotide requirements for microtubule polymerization in vitro. Biochemistry. 1975 Jul;14(13):2996–3005. doi: 10.1021/bi00684a032. [DOI] [PubMed] [Google Scholar]
- Olmsted J. B., Marcum J. M., Johnson K. A., Allen C., Borisy G. G. Microtuble assembly: some possible regulatory mechanisms. J Supramol Struct. 1974;2(2-4):429–450. doi: 10.1002/jss.400020230. [DOI] [PubMed] [Google Scholar]
- Palmer G. R., Clark D. C., Bayley P. M., Sattelle D. B. A quasi-elastic laser light scattering study of tubulin and microtubule protein from bovine brain. J Mol Biol. 1982 Oct 5;160(4):641–658. doi: 10.1016/0022-2836(82)90320-5. [DOI] [PubMed] [Google Scholar]
- Pantaloni D., Carlier M. F., Simon C., Batelier G. Mechanism of tubulin assembly: role of rings in the nucleation process and of associated proteins in the stabilization of microtubules. Biochemistry. 1981 Aug 4;20(16):4709–4716. doi: 10.1021/bi00519a029. [DOI] [PubMed] [Google Scholar]
- Ponstingl H., Krauhs E., Little M., Kempf T. Complete amino acid sequence of alpha-tubulin from porcine brain. Proc Natl Acad Sci U S A. 1981 May;78(5):2757–2761. doi: 10.1073/pnas.78.5.2757. [DOI] [PMC free article] [PubMed] [Google Scholar]
- Sandoval I. V., Vandekerckhove J. S. A comparative study of the in vitro polymerization of tubulin in the presence of the microtubule-associated proteins MAP2 and tau. J Biol Chem. 1981 Aug 25;256(16):8795–8800. [PubMed] [Google Scholar]
- Sandoval I. V., Weber K. Different tubulin polymers are produced by microtubule-associated proteins MAP2 and tau in the presence of guanosine 5'-(alpha, beta-methylene)triphosphate. J Biol Chem. 1980 Oct 10;255(19):8952–8954. [PubMed] [Google Scholar]
- Scheele R. B., Borisy G. G. Comparison of the sedimentation properties of microtubule protein oligomers prepared by two different procedures. Biochem Biophys Res Commun. 1976 May 3;70(1):1–7. doi: 10.1016/0006-291x(76)91100-1. [DOI] [PubMed] [Google Scholar]
- Shelanski M. L., Gaskin F., Cantor C. R. Microtubule assembly in the absence of added nucleotides. Proc Natl Acad Sci U S A. 1973 Mar;70(3):765–768. doi: 10.1073/pnas.70.3.765. [DOI] [PMC free article] [PubMed] [Google Scholar]
- Stearns M. E., Brown D. L. Purification of a microtubule-associated protein based on its preferential association with tubulin during microtubule initiation. FEBS Lett. 1979 May 1;101(1):15–20. [PubMed] [Google Scholar]
- Timasheff S. N., Grisham L. M. In vitro assembly of cytoplasmic microtubules. Annu Rev Biochem. 1980;49:565–591. doi: 10.1146/annurev.bi.49.070180.003025. [DOI] [PubMed] [Google Scholar]
- Weisenberg R. C. Role of co-operative interactions, microtubule-associated proteins and guanosine triphosphate in microtubule assembly: a model. J Mol Biol. 1980 Jun 5;139(4):660–677. [PubMed] [Google Scholar]
- Williams R. C., Jr, Detrich H. W., 3rd Separation of tubulin from microtubule-associated proteins on phosphocellulose. Accompanying alterations in concentrations of buffer components. Biochemistry. 1979 Jun 12;18(12):2499–2503. doi: 10.1021/bi00579a010. [DOI] [PubMed] [Google Scholar]
- Woody R. W., Clark D. C., Roberts G. C., Martin S. R., Bayley P. M. Molecular flexibility in microtubule proteins: proton nuclear magnetic resonance characterization. Biochemistry. 1983 Apr 26;22(9):2186–2192. doi: 10.1021/bi00278a020. [DOI] [PubMed] [Google Scholar]