Abstract
Energy metabolism in proliferating cultured rat thymocytes was compared with that of freshly prepared non-proliferating resting cells. Cultured rat thymocytes enter a proliferative cycle after stimulation by concanavalin A and Lymphocult T (interleukin-2), with maximal rates of DNA synthesis at 60 h. Compared with incubated resting thymocytes, glucose metabolism by incubated proliferating thymocytes was 53-fold increased; 90% of the amount of glucose utilized was converted into lactate, whereas resting cells metabolized only 56% to lactate. However, the latter oxidized 27% of glucose to CO2, as opposed to 1.1% by the proliferating cells. Activities of hexokinase, 6-phosphofructokinase, pyruvate kinase and aldolase in proliferating thymocytes were increased 12-, 17-, 30- and 24-fold respectively, whereas the rate of pyruvate oxidation was enhanced only 3-fold. The relatively low capacity of pyruvate degradation in proliferating thymocytes might be the reason for almost complete conversion of glucose into lactate by these cells. Glutamine utilization by rat thymocytes was 8-fold increased during proliferation. The major end products of glutamine metabolism are glutamate, aspartate, CO2 and ammonia. A complete recovery of glutamine carbon and nitrogen in the products was obtained. The amount of glutamate formed by phosphate-dependent glutaminase which entered the citric acid cycle was enhanced 5-fold in the proliferating cells: 76% was converted into 2-oxoglutarate by aspartate aminotransferase, present in high activity, and the remaining 24% by glutamate dehydrogenase. With resting cells the same percentages were obtained (75 and 25). Maximal activities of glutaminase, glutamate dehydrogenase and aspartate aminotransferase were increased 3-, 12- and 6-fold respectively in proliferating cells; 32% of the glutamate metabolized in the citric acid cycle was recovered in CO2 and 61% in aspartate. In resting cells this proportion was 41% and 59% and in mitogen-stimulated cells 39% and 65% respectively. Addition of glucose (4 mM) or malate (2 mM) strongly decreased the rates of glutamine utilization and glutamate conversion into 2-oxoglutarate by proliferating thymocytes and also affected the pathways of further glutamate metabolism. Addition of 2 mM-pyruvate did not alter the rate of glutamine utilization by proliferating thymocytes, but decreased the rate of metabolism beyond the stage of glutamate significantly. Formation of acetyl-CoA in the presence of pyruvate might explain the relatively enhanced oxidation of glutamate to CO2 (56%) by proliferating thymocytes.
Full text
PDF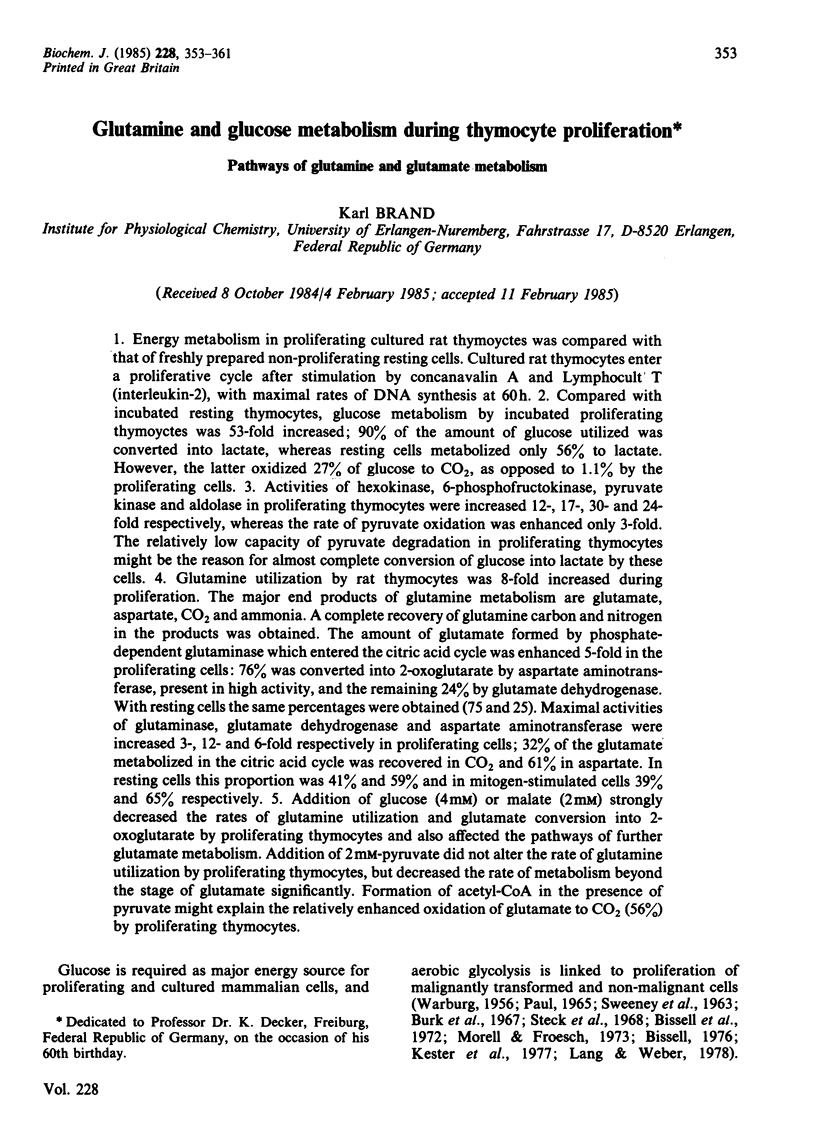
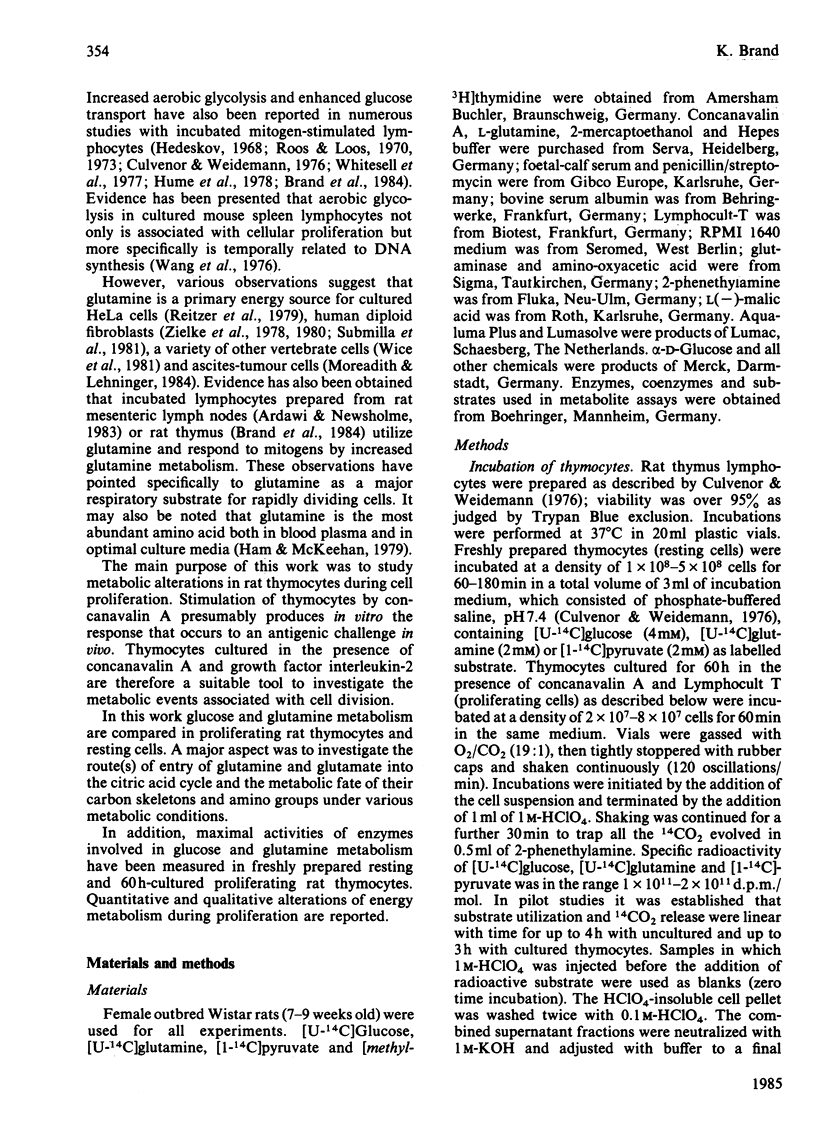
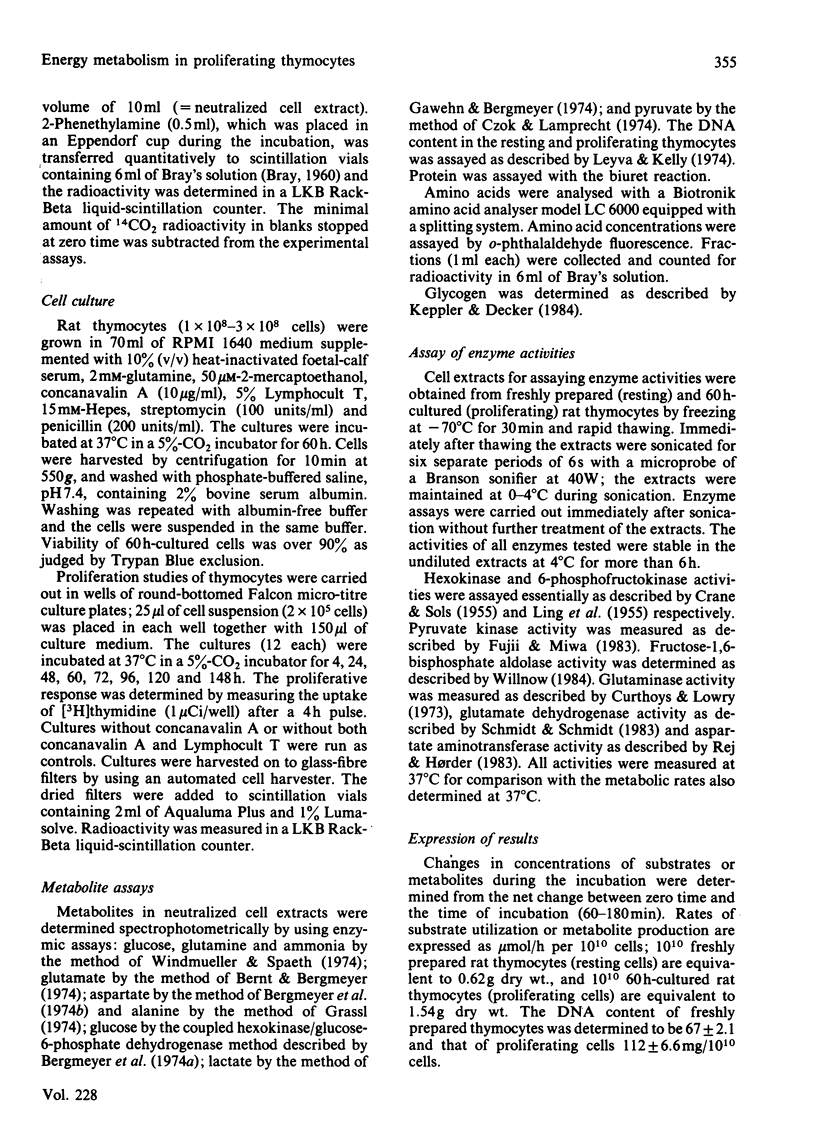
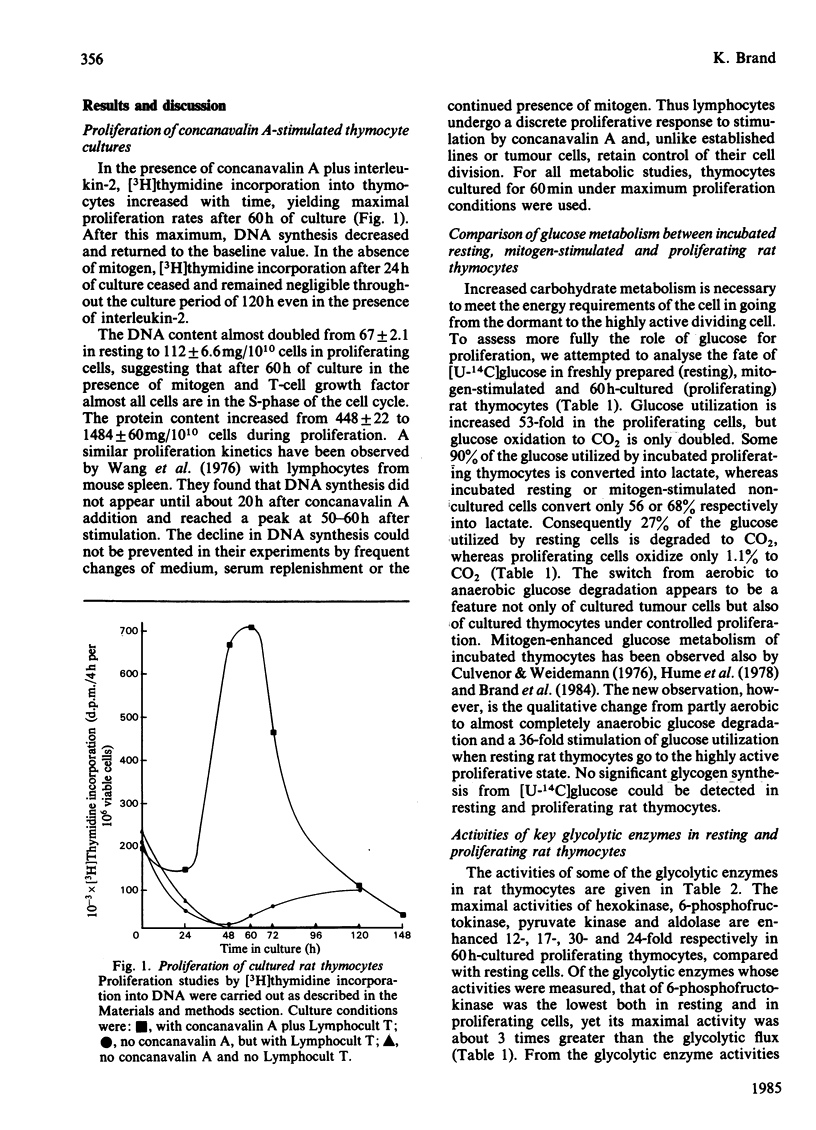
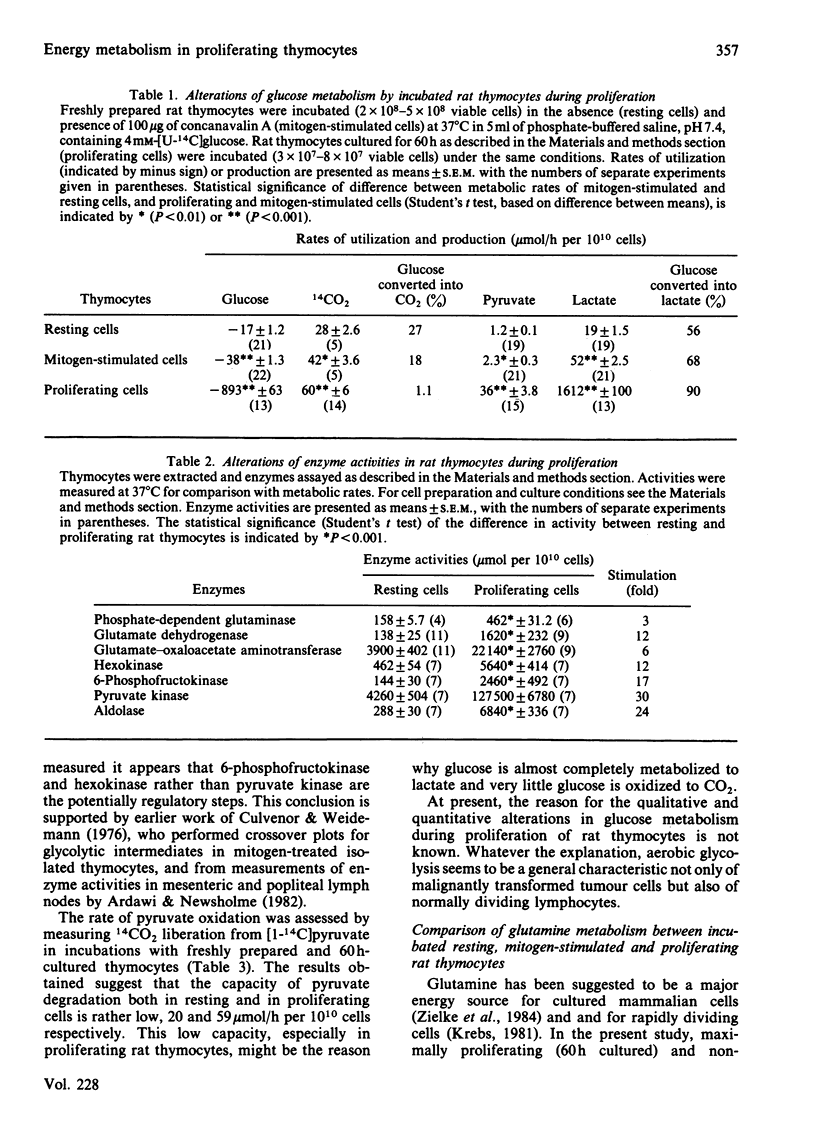
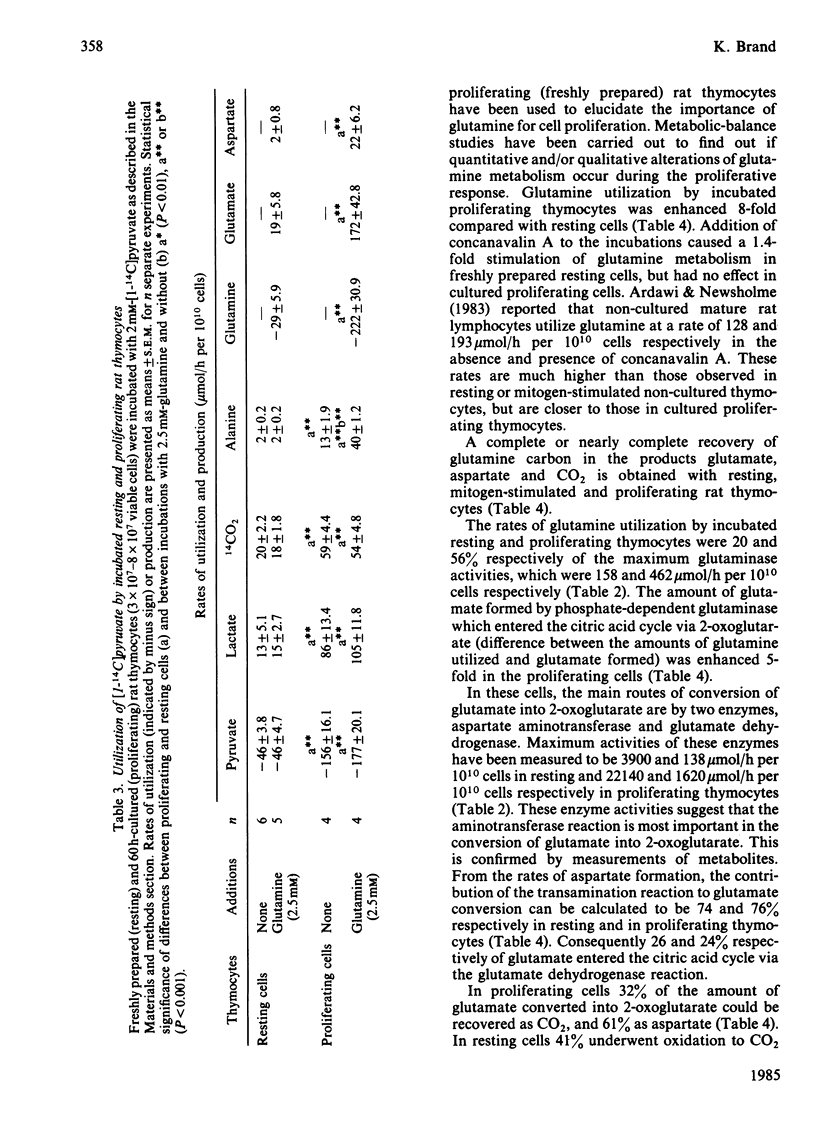
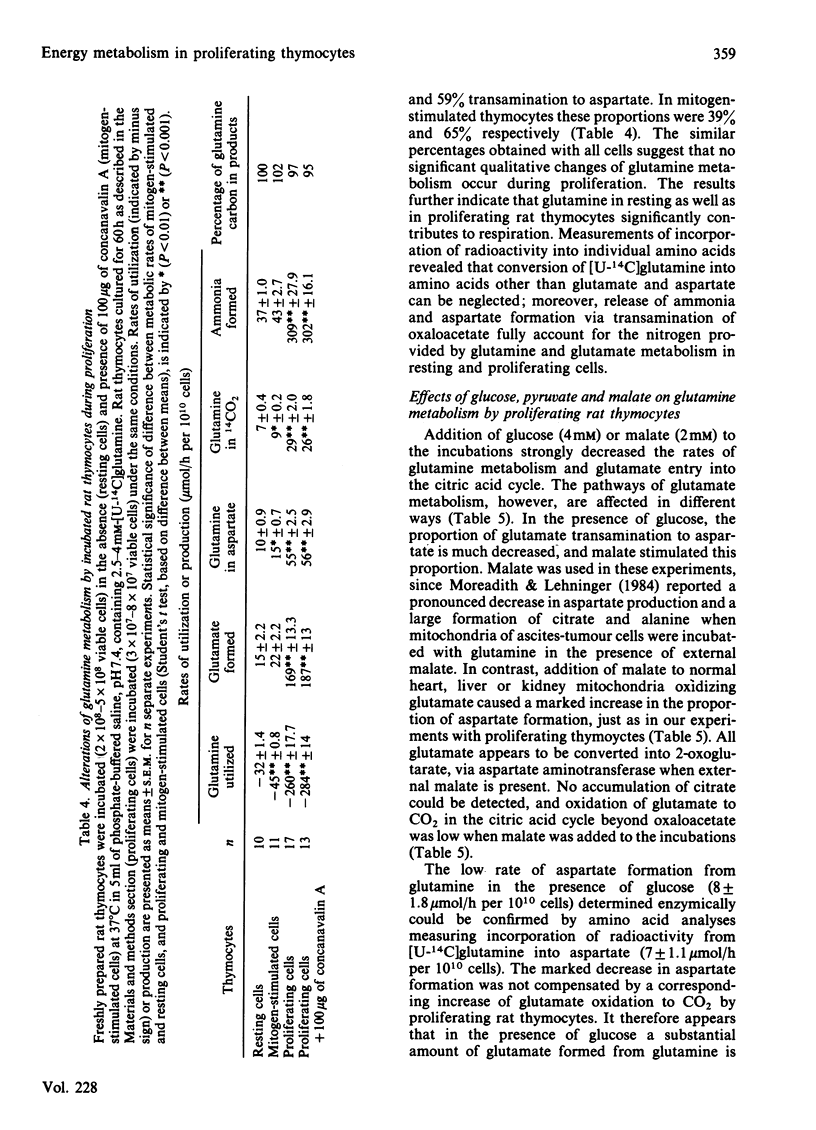
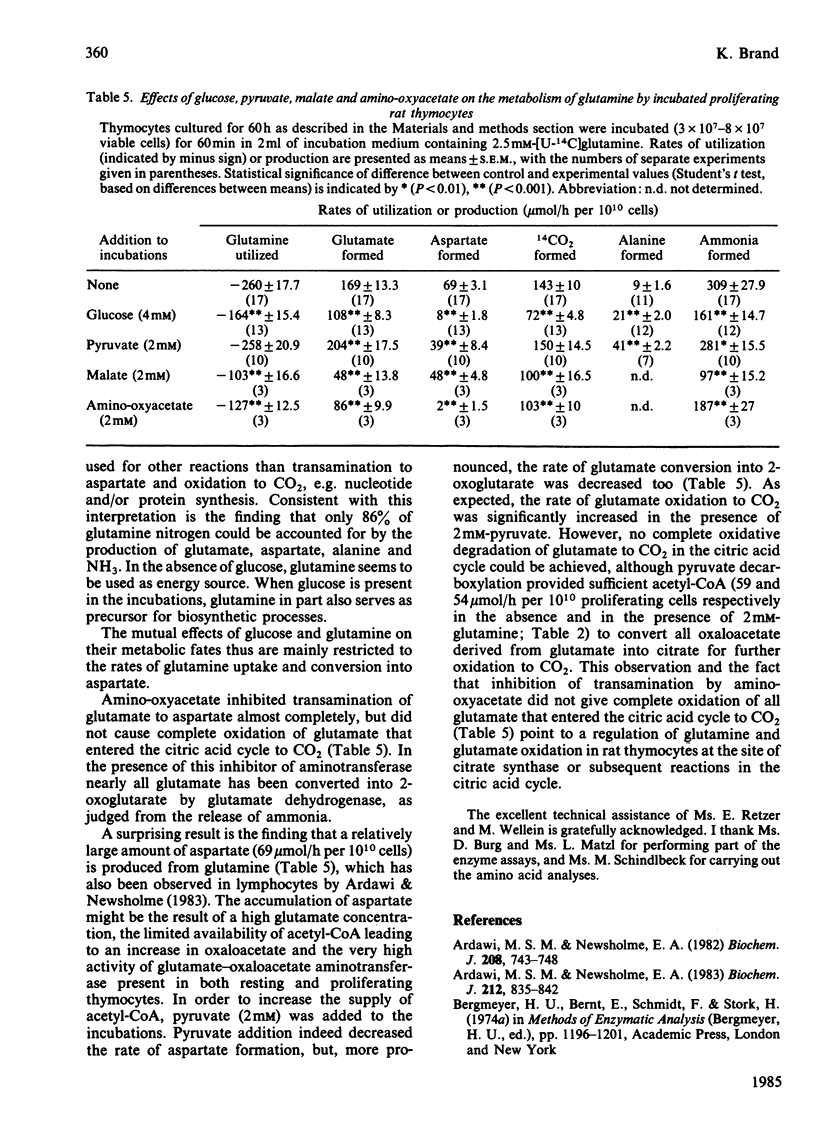
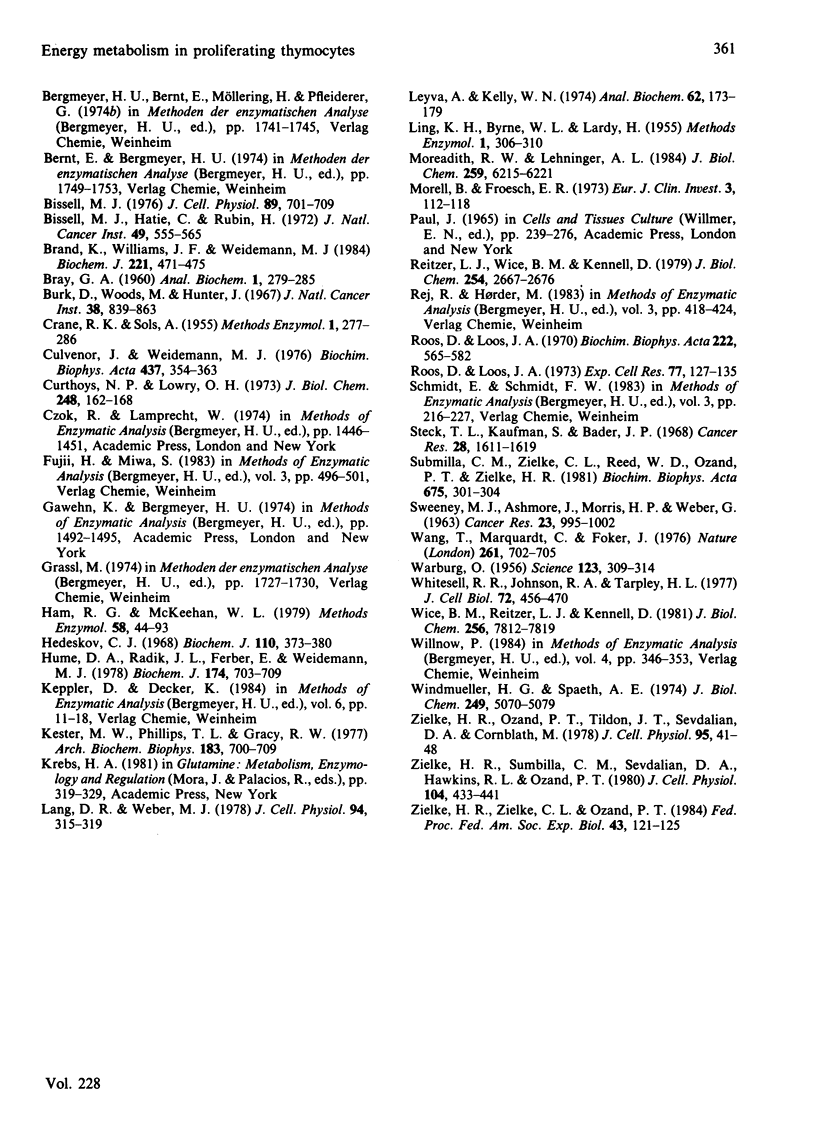
Selected References
These references are in PubMed. This may not be the complete list of references from this article.
- Ardawi M. S., Newsholme E. A. Glutamine metabolism in lymphocytes of the rat. Biochem J. 1983 Jun 15;212(3):835–842. doi: 10.1042/bj2120835. [DOI] [PMC free article] [PubMed] [Google Scholar]
- Ardawi M. S., Newsholme E. A. Maximum activities of some enzymes of glycolysis, the tricarboxylic acid cycle and ketone-body and glutamine utilization pathways in lymphocytes of the rat. Biochem J. 1982 Dec 15;208(3):743–748. doi: 10.1042/bj2080743. [DOI] [PMC free article] [PubMed] [Google Scholar]
- Bissell M. J., Hatié C., Rubin H. Patterns of glucose metabolism in normal and virus-transformed chick cells in tissue culture. J Natl Cancer Inst. 1972 Aug;49(2):555–565. [PubMed] [Google Scholar]
- Bissell M. J. Transport as a rate limiting step in glucose metabolism in virus-transformed cells: studies with cytochalasin B. J Cell Physiol. 1976 Dec;89(4):701–709. doi: 10.1002/jcp.1040890430. [DOI] [PubMed] [Google Scholar]
- Bradbury E. M., Moss T., Hayashi H., Hjelm R. P., Suau P., Stephens R. M., Baldwin J. P., Crane-Robinson C. Nucleosomes, histone interactions, and the role of histones H3 and H4. Cold Spring Harb Symp Quant Biol. 1978;42(Pt 1):277–286. doi: 10.1101/sqb.1978.042.01.030. [DOI] [PubMed] [Google Scholar]
- Brand K., Williams J. F., Weidemann M. J. Glucose and glutamine metabolism in rat thymocytes. Biochem J. 1984 Jul 15;221(2):471–475. doi: 10.1042/bj2210471. [DOI] [PMC free article] [PubMed] [Google Scholar]
- Burk D., Woods M., Hunter J. On the significance of glucolysis for cancer growth, with special reference to Morris rat hepatomas. J Natl Cancer Inst. 1967 Jun;38(6):839–863. [PubMed] [Google Scholar]
- Culvenor J. G., Weidemann M. J. Phytohaemagglutinin stimulation of rat thymus lymphocytes glycolysis. Biochim Biophys Acta. 1976 Jul 21;437(2):354–363. doi: 10.1016/0304-4165(76)90005-2. [DOI] [PubMed] [Google Scholar]
- Curthoys N. P., Lowry O. H. The distribution of glutaminase isoenzymes in the various structures of the nephron in normal, acidotic, and alkalotic rat kidney. J Biol Chem. 1973 Jan 10;248(1):162–168. [PubMed] [Google Scholar]
- Ham R. G., McKeehan W. L. Media and growth requirements. Methods Enzymol. 1979;58:44–93. doi: 10.1016/s0076-6879(79)58126-9. [DOI] [PubMed] [Google Scholar]
- Hedeskov C. J. Early effects of phytohaemagglutinin on glucose metabolism of normal human lymphocytes. Biochem J. 1968 Nov;110(2):373–380. doi: 10.1042/bj1100373. [DOI] [PMC free article] [PubMed] [Google Scholar]
- Hume D. A., Radik J. L., Ferber E., Weidemann M. J. Aerobic glycolysis and lymphocyte transformation. Biochem J. 1978 Sep 15;174(3):703–709. doi: 10.1042/bj1740703. [DOI] [PMC free article] [PubMed] [Google Scholar]
- Kester M. V., Phillips T. L., Gracy R. W. Changes in glycolytic enzyme levels and isozyme expression in human lymphocytes during blast transformation. Arch Biochem Biophys. 1977 Oct;183(2):700–709. doi: 10.1016/0003-9861(77)90403-9. [DOI] [PubMed] [Google Scholar]
- Lang D. R., Weber M. J. Increased membrane transport of 2-deoxyglucose and 3-O-methylglucose is an early event in the transformation of chick embryo fibroblasts by Rous sarcoma virus. J Cell Physiol. 1978 Mar;94(3):315–319. doi: 10.1002/jcp.1040940309. [DOI] [PubMed] [Google Scholar]
- Leyva A., Jr, Kelley W. N. Measurement of DNA in cultured human cells. Anal Biochem. 1974 Nov;62(1):173–179. doi: 10.1016/0003-2697(74)90378-9. [DOI] [PubMed] [Google Scholar]
- Moreadith R. W., Lehninger A. L. The pathways of glutamate and glutamine oxidation by tumor cell mitochondria. Role of mitochondrial NAD(P)+-dependent malic enzyme. J Biol Chem. 1984 May 25;259(10):6215–6221. [PubMed] [Google Scholar]
- Morell B., Froesch E. R. Fibroblasts as an experimental tool in metabolic and hormone studies. I. Growth and glucose metabolism of fibroblasts in culture. Eur J Clin Invest. 1973 Mar;3(2):112–118. doi: 10.1111/j.1365-2362.1973.tb00337.x. [DOI] [PubMed] [Google Scholar]
- Reitzer L. J., Wice B. M., Kennell D. Evidence that glutamine, not sugar, is the major energy source for cultured HeLa cells. J Biol Chem. 1979 Apr 25;254(8):2669–2676. [PubMed] [Google Scholar]
- Roos D., Loos J. A. Changes in the carbohydrate metabolism of mitogenically stimulated human peripheral lymphocytes. I. Stimulation by phytohaemagglutinin. Biochim Biophys Acta. 1970 Dec 29;222(3):565–582. doi: 10.1016/0304-4165(70)90182-0. [DOI] [PubMed] [Google Scholar]
- Roos D., Loos J. A. Changes in the carbohydrate metabolism of mitogenically stimulated human peripheral lymphocytes. II. Relative importance of glycolysis and oxidative phosphorylation on phytohaemagglutinin stimulation. Exp Cell Res. 1973 Mar 15;77(1):127–135. doi: 10.1016/0014-4827(73)90561-2. [DOI] [PubMed] [Google Scholar]
- SWEENEY M. J., ASHMORE J., MORRIS H. P., WEBER G. COMPARATIVE BIOCHEMISTRY HEPATOMAS. IV. ISOTOPE STUDIES OF GLUCOSE AND FRUCTOSE METABOLISM IN LIVER TUMORS OF DIFFERENT GROWTH RATES. Cancer Res. 1963 Aug;23:995–1002. [PubMed] [Google Scholar]
- Steck T. L., Kaufman S., Bader J. P. Glycolysis in chick embryo cell cultures transformed by Rous sarcoma virus. Cancer Res. 1968 Aug;28(8):1611–1619. [PubMed] [Google Scholar]
- Sumbilla C. M., Zielke C. L., Reed W. D., Ozand P. T., Zielke H. R. Comparison of the oxidation of glutamine, glucose, ketone bodies and fatty acids by human diploid fibroblasts. Biochim Biophys Acta. 1981 Jul;675(2):301–304. doi: 10.1016/0304-4165(81)90242-7. [DOI] [PubMed] [Google Scholar]
- WARBURG O. On the origin of cancer cells. Science. 1956 Feb 24;123(3191):309–314. doi: 10.1126/science.123.3191.309. [DOI] [PubMed] [Google Scholar]
- Wang T., Marquardt C., Foker J. Aerobic glycolysis during lymphocyte proliferation. Nature. 1976 Jun 24;261(5562):702–705. doi: 10.1038/261702a0. [DOI] [PubMed] [Google Scholar]
- Whitesell R. R., Johnson R. A., Tarpley H. L., Regen D. M. Mitogen-stimulated glucose transport in thymocytes. Possible role of Ca++ and antagonism by adenosine 3':5'-monophosphate. J Cell Biol. 1977 Feb;72(2):456–469. doi: 10.1083/jcb.72.2.456. [DOI] [PMC free article] [PubMed] [Google Scholar]
- Wice B. M., Reitzer L. J., Kennell D. The continuous growth of vertebrate cells in the absence of sugar. J Biol Chem. 1981 Aug 10;256(15):7812–7819. [PubMed] [Google Scholar]
- Windmueller H. G., Spaeth A. E. Uptake and metabolism of plasma glutamine by the small intestine. J Biol Chem. 1974 Aug 25;249(16):5070–5079. [PubMed] [Google Scholar]
- Zielke H. R., Ozand P. T., Tildon J. T., Sevdalian D. A., Cornblath M. Reciprocal regulation of glucose and glutamine utilization by cultured human diploid fibroblasts. J Cell Physiol. 1978 Apr;95(1):41–48. doi: 10.1002/jcp.1040950106. [DOI] [PubMed] [Google Scholar]
- Zielke H. R., Sumbilla C. M., Sevdalian D. A., Hawkins R. L., Ozand P. T. Lactate: a major product of glutamine metabolism by human diploid fibroblasts. J Cell Physiol. 1980 Sep;104(3):433–441. doi: 10.1002/jcp.1041040316. [DOI] [PubMed] [Google Scholar]
- Zielke H. R., Zielke C. L., Ozand P. T. Glutamine: a major energy source for cultured mammalian cells. Fed Proc. 1984 Jan;43(1):121–125. [PubMed] [Google Scholar]