Abstract
Meprin is an intrinsic protein of the brush border, a specialized plasma membrane, of the mouse kidney. It is a metalloendopeptidase that contains 1 mol of zinc and 3 mol of calcium per mol of the 85,000-Mr subunit. The enzyme is isolated, and active, as a tetramer. The behaviour of the enzyme on SDS/polyacrylamide gels in the presence and absence of beta-mercaptoethanol indicates that the subunits are of the same Mr (approx. 85,000) and held together by intersubunit S--S bridges. Eight S-carboxymethyl-L-cysteine residues were detected after reduction of the enzyme with beta-mercaptoethanol and carboxymethylation with iodoacetate. The enzyme is a glycoprotein and contains approx. 18% carbohydrate. Most of the carbohydrate is removed by endoglycosidase F, indicating that the sugar residues are N-linked. The isoelectric point of the enzyme is between pH 4 and 5, and the purified protein yields a pattern of evenly spaced bands in this range on isoelectric focusing. The peptide-bond specificity of the enzyme has been determined by using the oxidized B-chain of insulin as substrate. In all, 15 peptide degradation products were separated by h.p.l.c. and analysed for their amino acid content and N-terminal amino acid residue. The prevalent peptide-bond cleavages were between Gly20 and Glu21, Phe24 and Phe25 and between Phe25 and Tyr26. Other sites of cleavage were Leu6-Cysteic acid7, Ala14-Leu15, His10-Leu11, Leu17-Val18, Gly8-Ser9, Leu15-Tyr16, His5-Leu6. These results indicate that meprin has a preference for peptide bonds that are flanked by hydrophobic or neutral amino acid residues, but hydrolysis is not limited to these bonds. The ability of meprin to hydrolyse peptide bonds between small neutral and negatively charged amino acid residues distinguishes it from several other metalloendopeptidases.
Full text
PDF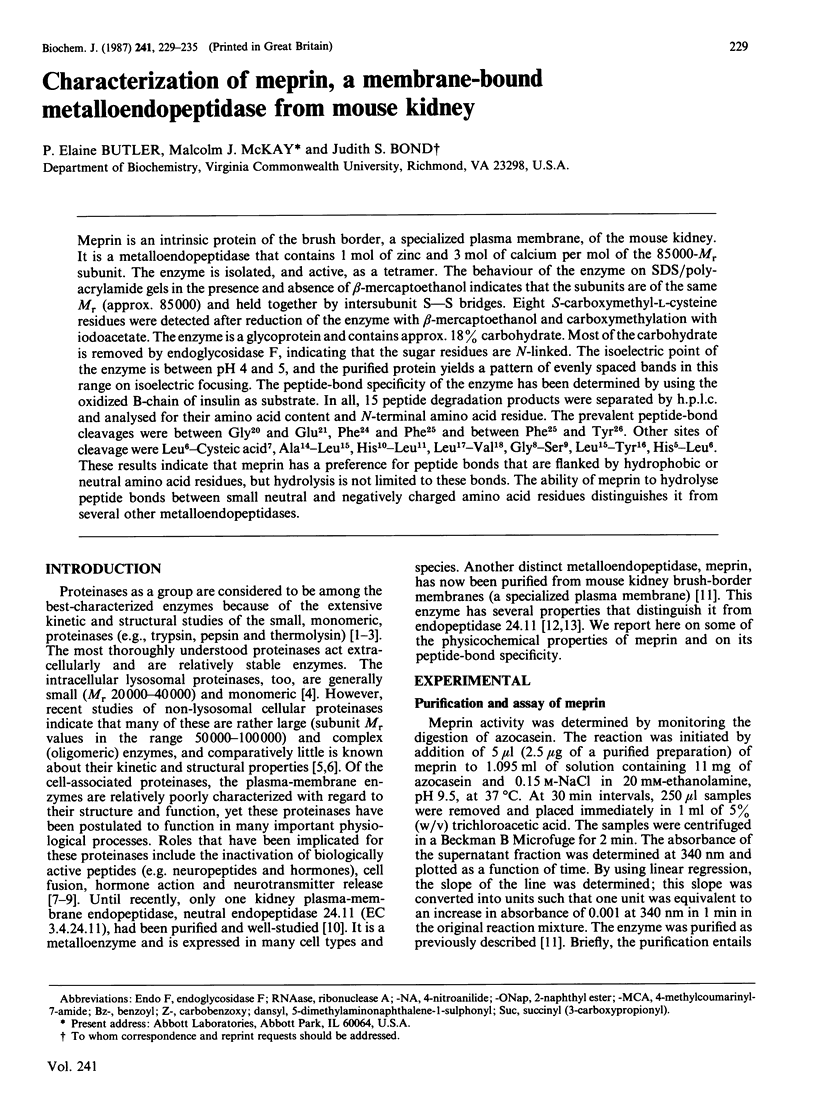
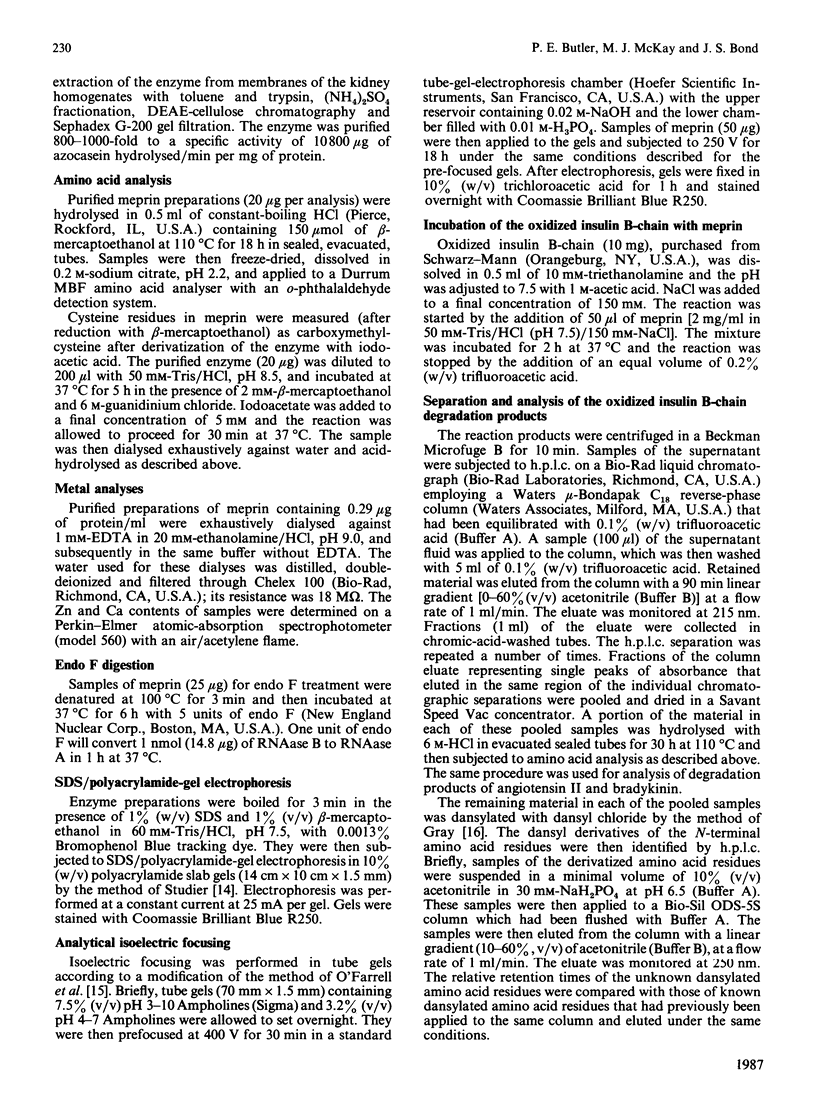
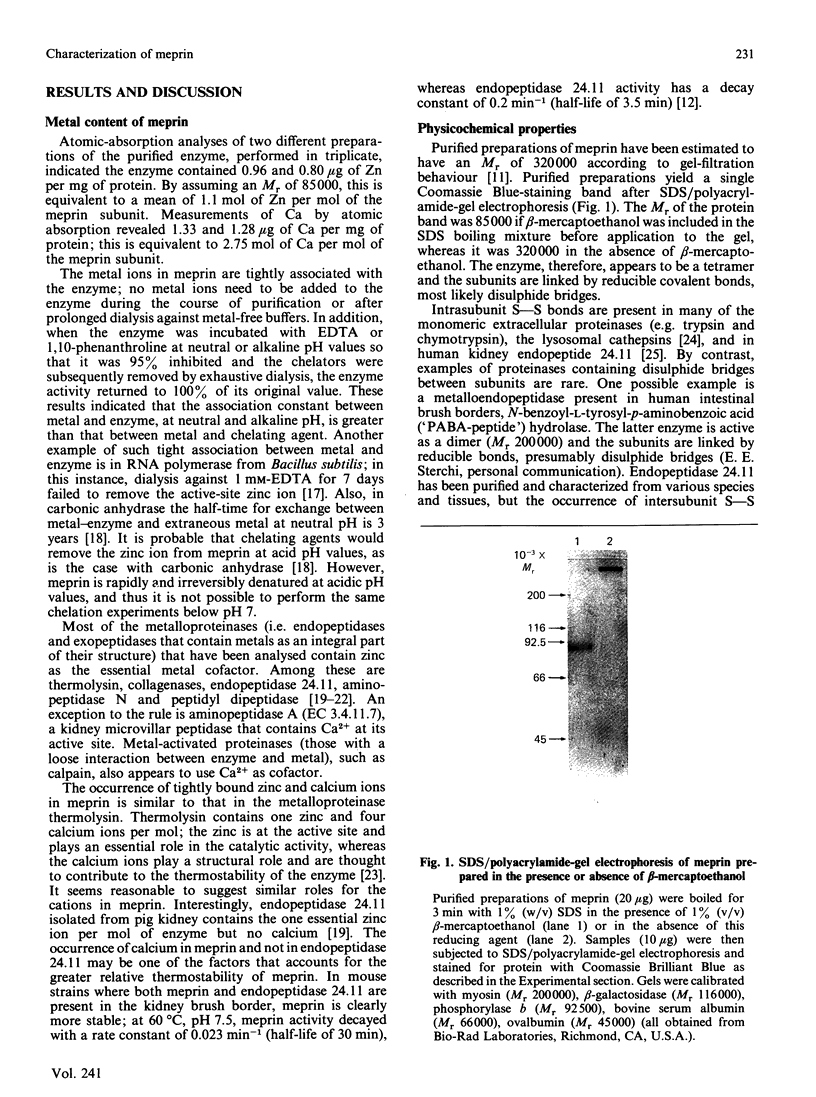
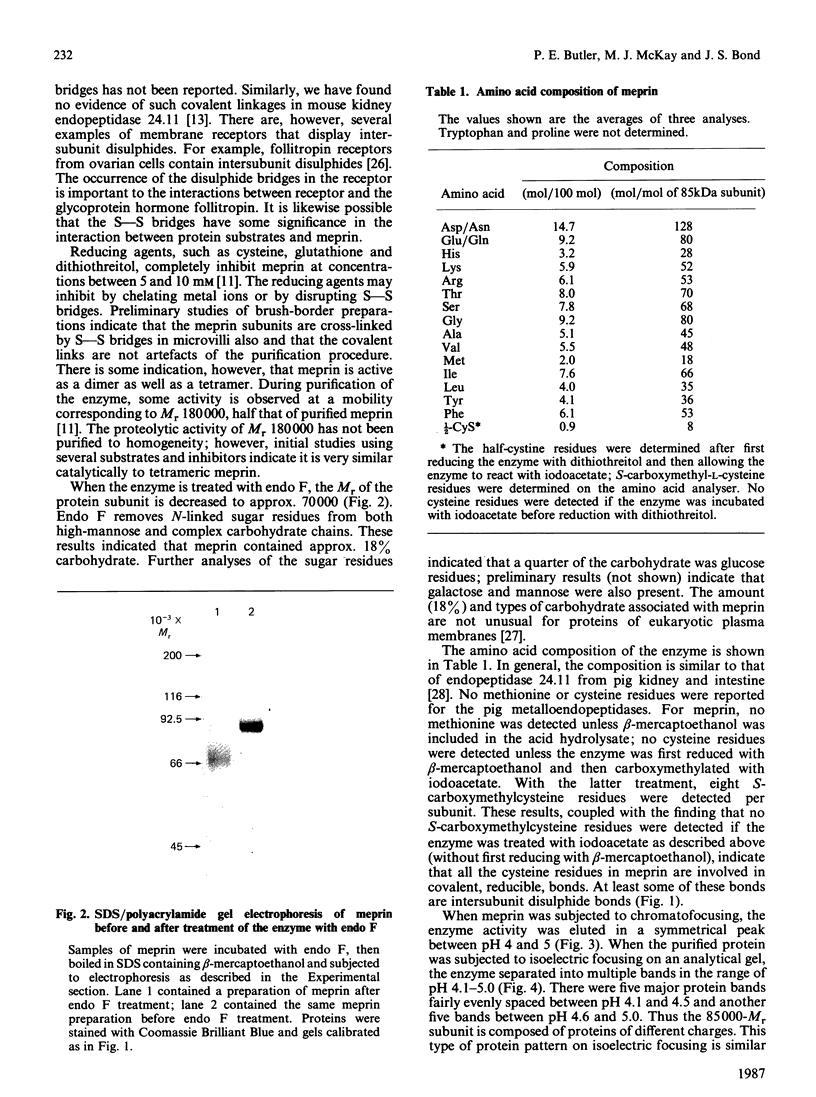
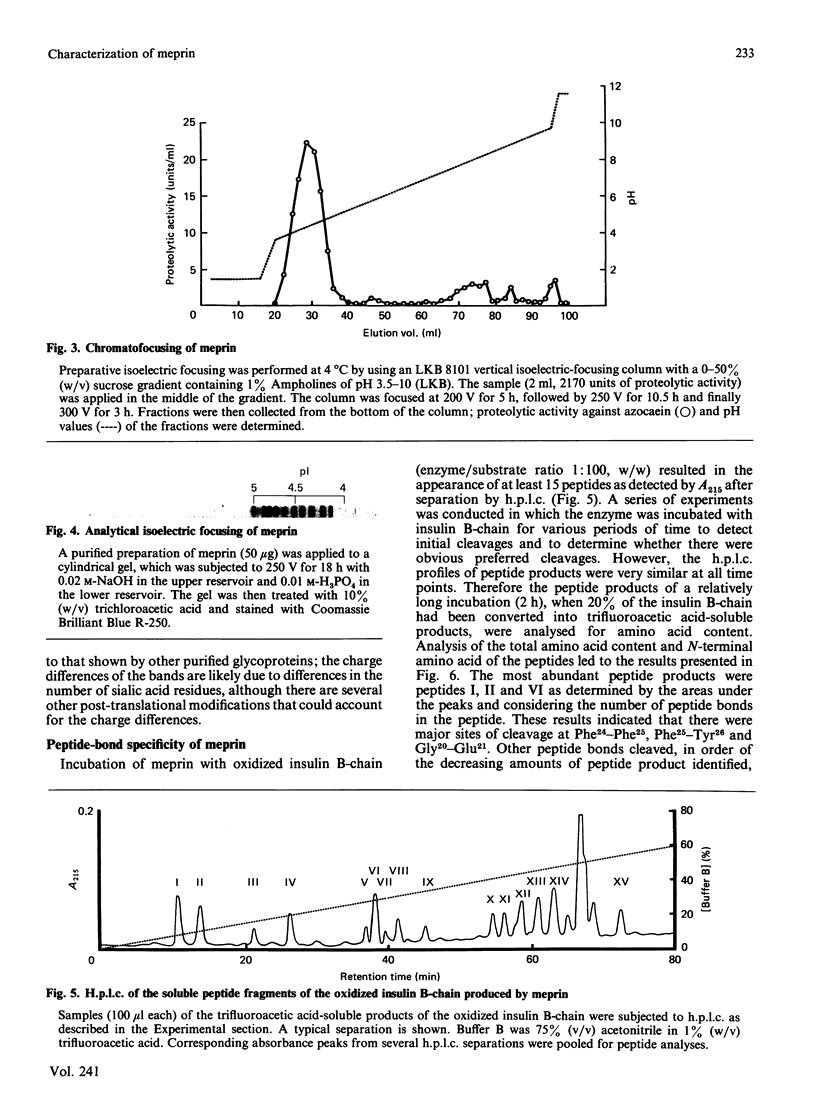
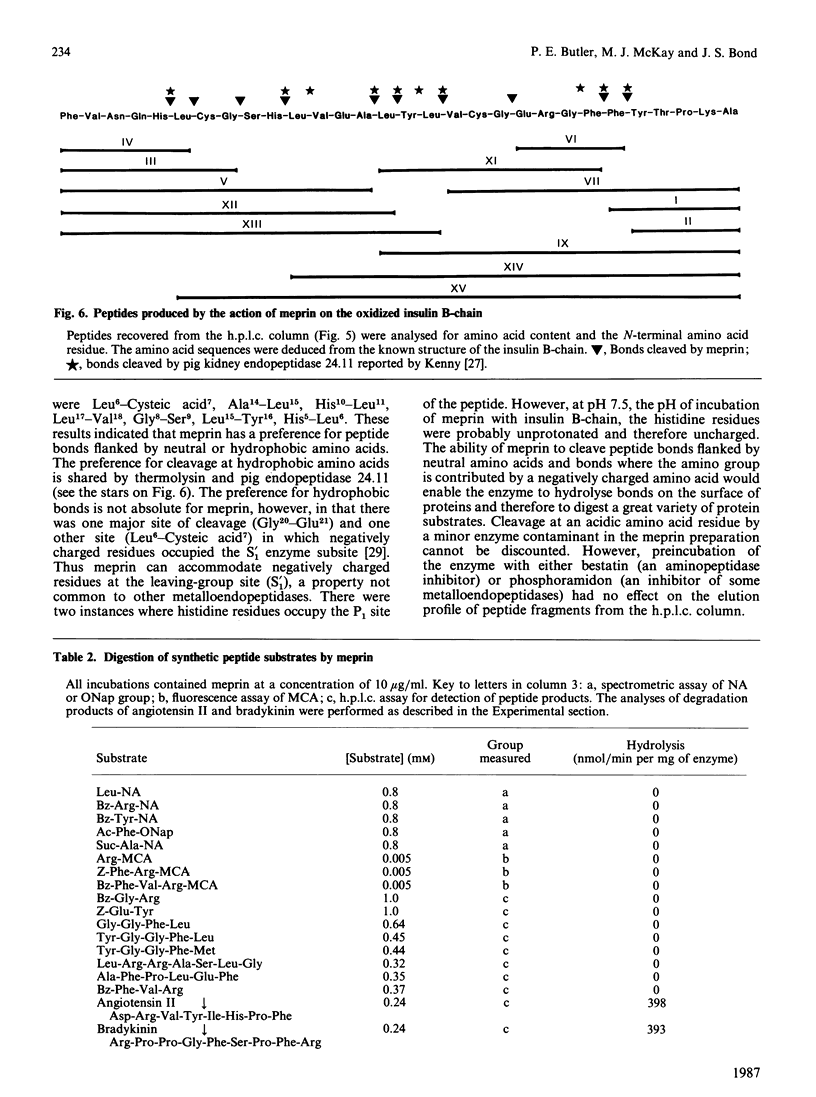
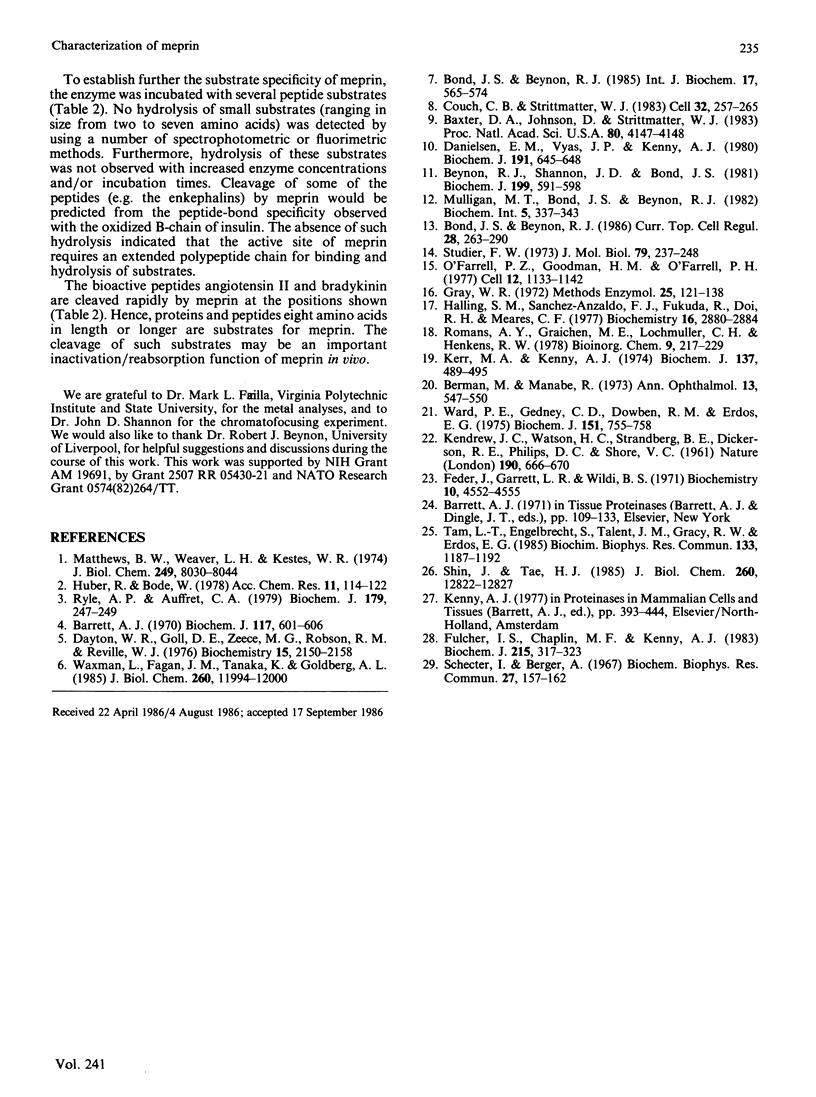
Images in this article
Selected References
These references are in PubMed. This may not be the complete list of references from this article.
- Barrett A. J. Cathepsin D. Purification of isoenzymes from human and chicken liver. Biochem J. 1970 Apr;117(3):601–607. doi: 10.1042/bj1170601. [DOI] [PMC free article] [PubMed] [Google Scholar]
- Beynon R. J., Shannon J. D., Bond J. S. Purification and characterization of a metallo-endoproteinase from mouse kidney. Biochem J. 1981 Dec 1;199(3):591–598. doi: 10.1042/bj1990591. [DOI] [PMC free article] [PubMed] [Google Scholar]
- Bond J. S., Beynon R. J. Mammalian metalloendopeptidases. Int J Biochem. 1985;17(5):565–574. doi: 10.1016/0020-711x(85)90287-3. [DOI] [PubMed] [Google Scholar]
- Bond J. S., Beynon R. J. Meprin: a membrane-bound metallo-endopeptidase. Curr Top Cell Regul. 1986;28:263–290. doi: 10.1016/b978-0-12-152828-7.50009-3. [DOI] [PubMed] [Google Scholar]
- Couch C. B., Strittmatter W. J. Rat myoblast fusion requires metalloendoprotease activity. Cell. 1983 Jan;32(1):257–265. doi: 10.1016/0092-8674(83)90516-0. [DOI] [PubMed] [Google Scholar]
- Danielsen E. M., Vyas J. P., Kenny A. J. A neutral endopeptidase in the microvillar membrane of pig intestine. Partial purification and properties. Biochem J. 1980 Nov 1;191(2):645–648. doi: 10.1042/bj1910645. [DOI] [PMC free article] [PubMed] [Google Scholar]
- Dayton W. R., Goll D. E., Zeece M. G., Robson R. M., Reville W. J. A Ca2+-activated protease possibly involved in myofibrillar protein turnover. Purification from porcine muscle. Biochemistry. 1976 May 18;15(10):2150–2158. doi: 10.1021/bi00655a019. [DOI] [PubMed] [Google Scholar]
- Feder J., Garrett L. R., Wildi B. S. Studies on the role of calcium in thermolysin. Biochemistry. 1971 Nov 23;10(24):4552–4556. doi: 10.1021/bi00800a032. [DOI] [PubMed] [Google Scholar]
- Fulcher I. S., Chaplin M. F., Kenny A. J. Endopeptidase-24.11 purified from pig intestine is differently glycosylated from that in kidney. Biochem J. 1983 Nov 1;215(2):317–323. doi: 10.1042/bj2150317. [DOI] [PMC free article] [PubMed] [Google Scholar]
- Halling S. M., Sanchez-Anzaldo F. J., Fukuda R., Doi R. H., Meares C. F. Zinc is associated with the beta subunit of DNA-dependent RNA polymerase of Bacillus subtilis. Biochemistry. 1977 Jun 28;16(13):2880–2884. doi: 10.1021/bi00632a012. [DOI] [PubMed] [Google Scholar]
- KENDREW J. C., WATSON H. C., STRANDBERG B. E., DICKERSON R. E., PHILLIPS D. C., SHORE V. C. The amino-acid sequence x-ray methods, and its correlation with chemical data. Nature. 1961 May 20;190:666–670. doi: 10.1038/190666a0. [DOI] [PubMed] [Google Scholar]
- Kerr M. A., Kenny A. J. The molecular weight and properties of a neutral metallo-endopeptidase from rabbit kidney brush border. Biochem J. 1974 Mar;137(3):489–495. doi: 10.1042/bj1370489. [DOI] [PMC free article] [PubMed] [Google Scholar]
- Matthews B. W., Weaver L. H., Kester W. R. The conformation of thermolysin. J Biol Chem. 1974 Dec 25;249(24):8030–8044. [PubMed] [Google Scholar]
- O'Farrell P. Z., Goodman H. M., O'Farrell P. H. High resolution two-dimensional electrophoresis of basic as well as acidic proteins. Cell. 1977 Dec;12(4):1133–1141. doi: 10.1016/0092-8674(77)90176-3. [DOI] [PubMed] [Google Scholar]
- Romans A. Y., Graichen M. E., Lochmüller C. H., Henkens R. W. Kinetics and mechanism of dissociation of zinc ion from carbonic anhydrase. Bioinorg Chem. 1978 Sep;9(3):217–229. doi: 10.1016/s0006-3061(78)80007-6. [DOI] [PubMed] [Google Scholar]
- Ryle A. P., Auffret C. A. The specificity of some pig and human pepsins towards synthetic peptide substrates. Biochem J. 1979 Apr 1;179(1):247–249. doi: 10.1042/bj1790247. [DOI] [PMC free article] [PubMed] [Google Scholar]
- Schechter I., Berger A. On the size of the active site in proteases. I. Papain. Biochem Biophys Res Commun. 1967 Apr 20;27(2):157–162. doi: 10.1016/s0006-291x(67)80055-x. [DOI] [PubMed] [Google Scholar]
- Shin J., Ji T. H. Composition of cross-linked 125I-follitropin-receptor complexes. J Biol Chem. 1985 Oct 15;260(23):12822–12827. [PubMed] [Google Scholar]
- Studier F. W. Analysis of bacteriophage T7 early RNAs and proteins on slab gels. J Mol Biol. 1973 Sep 15;79(2):237–248. doi: 10.1016/0022-2836(73)90003-x. [DOI] [PubMed] [Google Scholar]
- Tam L. T., Engelbrecht S., Talent J. M., Gracy R. W., Erdös E. G. The importance of disulfide bridges in human endopeptidase (enkephalinase) after proteolytic cleavage. Biochem Biophys Res Commun. 1985 Dec 31;133(3):1187–1192. doi: 10.1016/0006-291x(85)91262-8. [DOI] [PubMed] [Google Scholar]
- Ward P. E., Gedney C. D., Dowben R. M., Erdös E. G. Isolation of membrane-bound renal kallikrein and kininase. Biochem J. 1975 Dec;151(3):755–758. doi: 10.1042/bj1510755. [DOI] [PMC free article] [PubMed] [Google Scholar]
- Waxman L., Fagan J. M., Tanaka K., Goldberg A. L. A soluble ATP-dependent system for protein degradation from murine erythroleukemia cells. Evidence for a protease which requires ATP hydrolysis but not ubiquitin. J Biol Chem. 1985 Oct 5;260(22):11994–12000. [PubMed] [Google Scholar]