Abstract
The kinetics of reduction of indigocarmine-dye-oxidized Fe protein of nitrogenase from Klebsiella pneumoniae (Kp2ox) by sodium dithionite in the presence and absence of MgADP were studied by stopped-flow spectrophotometry at 23 degrees C and at pH 7.4. Highly co-operative binding of 2MgADP (composite K greater than 4 X 10(10) M-2) to Kp2ox induced a rapid conformation change which caused the redox-active 4Fe-4S centre to be reduced by SO2-.(formed by the predissociation of dithionite ion) with k = 3 X 10(6) M-1.s-1. This rate constant is at least 30 times lower than that for the reduction of free Kp2ox (k greater than 10(8) M-1.s-1). Two mechanisms have been considered and limits obtained for the rate constants for MgADP binding/dissociation and a protein conformation change. Both mechanisms give rate constants (e.g. MgADP binding 3 X 10(5) less than k less than 3 X 10(6) M-1.s-1 and protein conformation change 6 X 10(2) less than k less than 6 X 10(3) s-1) that are similar to those reported for creatine kinase (EC 2.7.3.2). The kinetics also show that in the catalytic cycle of nitrogenase with sodium dithionite as reductant replacement of 2MgADP by 2MgATP occurs on reduced and not oxidized Kp2. Although the Kp2ox was reduced stoichiometrically by SO2-. and bound two equivalents of MgADP with complete conversion into the less-reactive conformation, it was only 45% active with respect to its ability to effect MgATP-dependent electron transfer to the MoFe protein.
Full text
PDF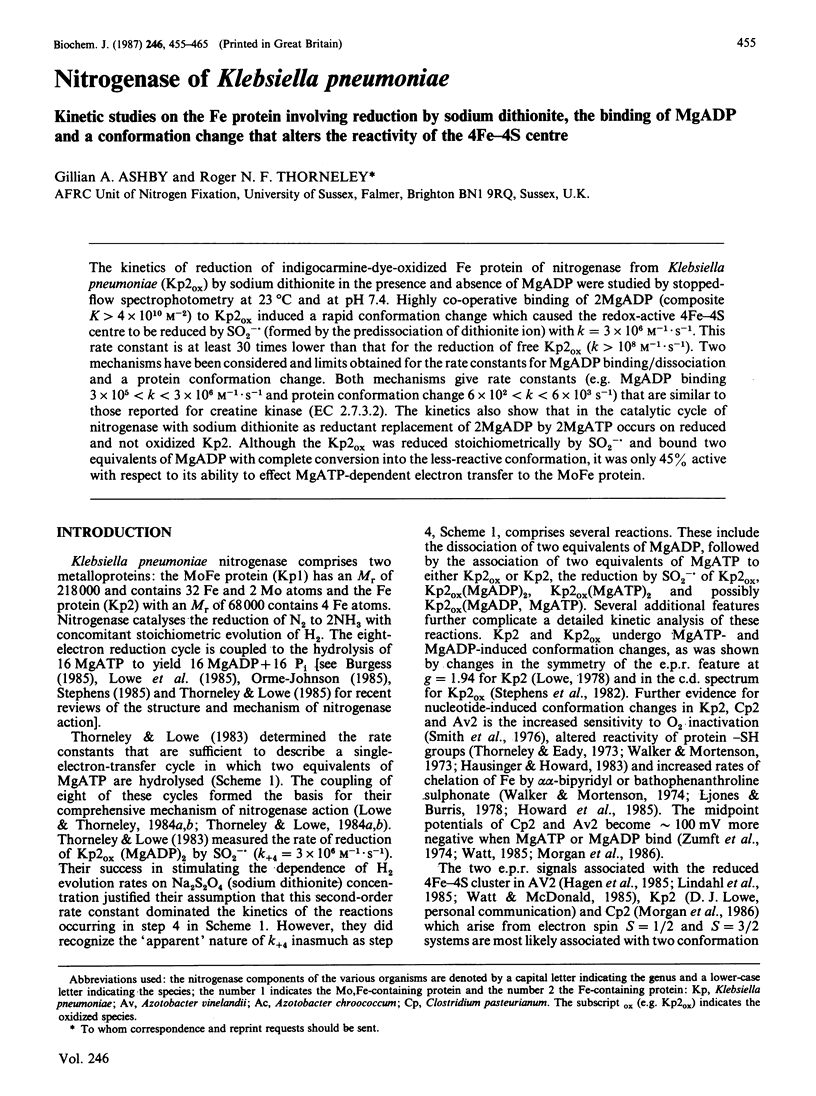
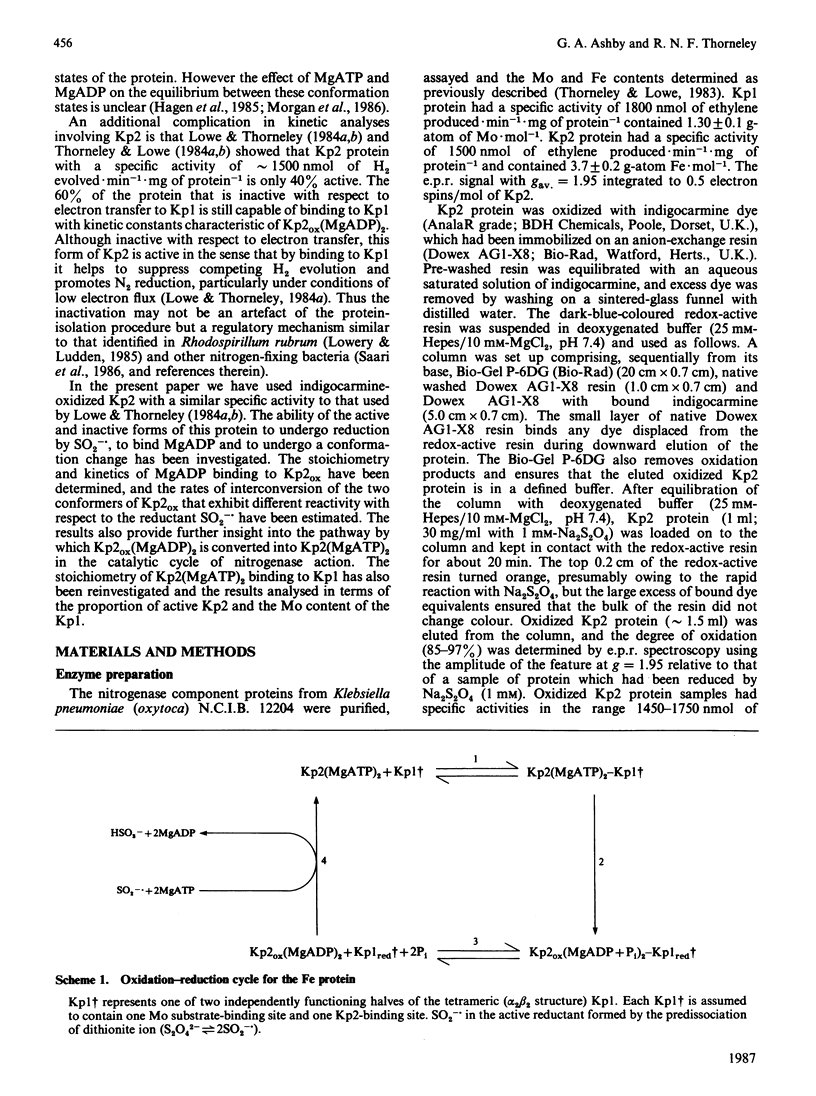
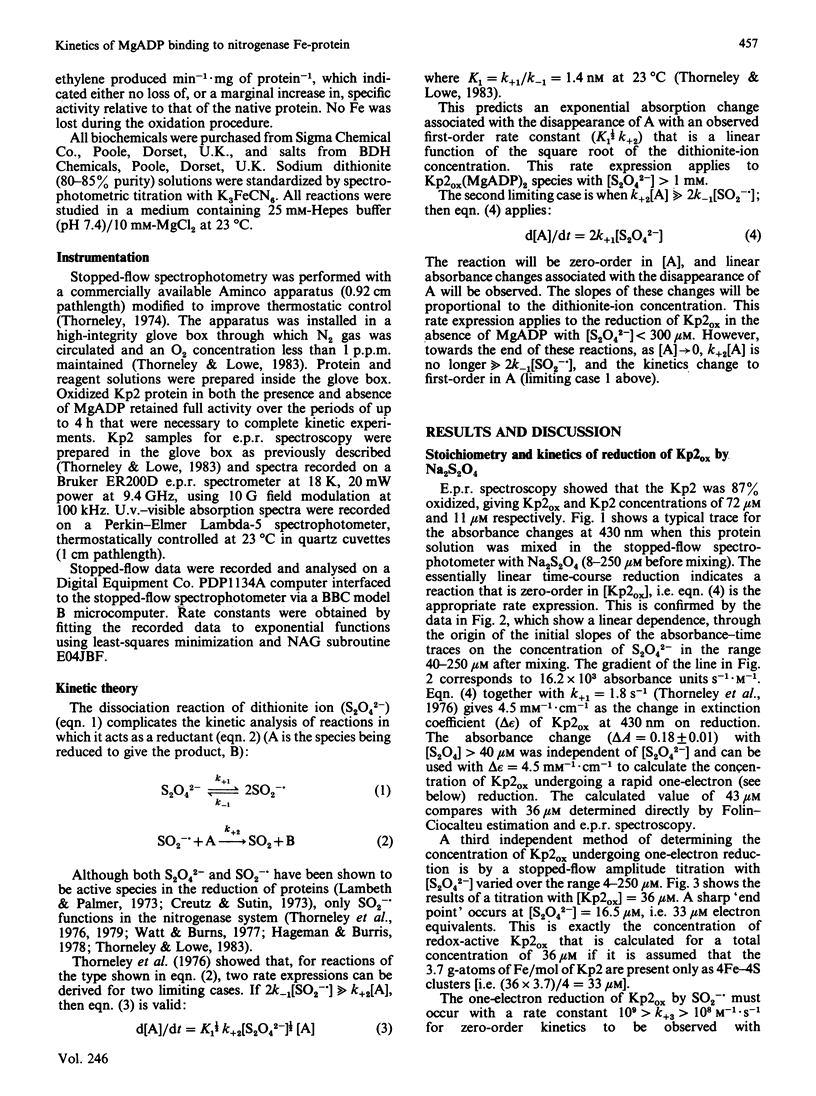
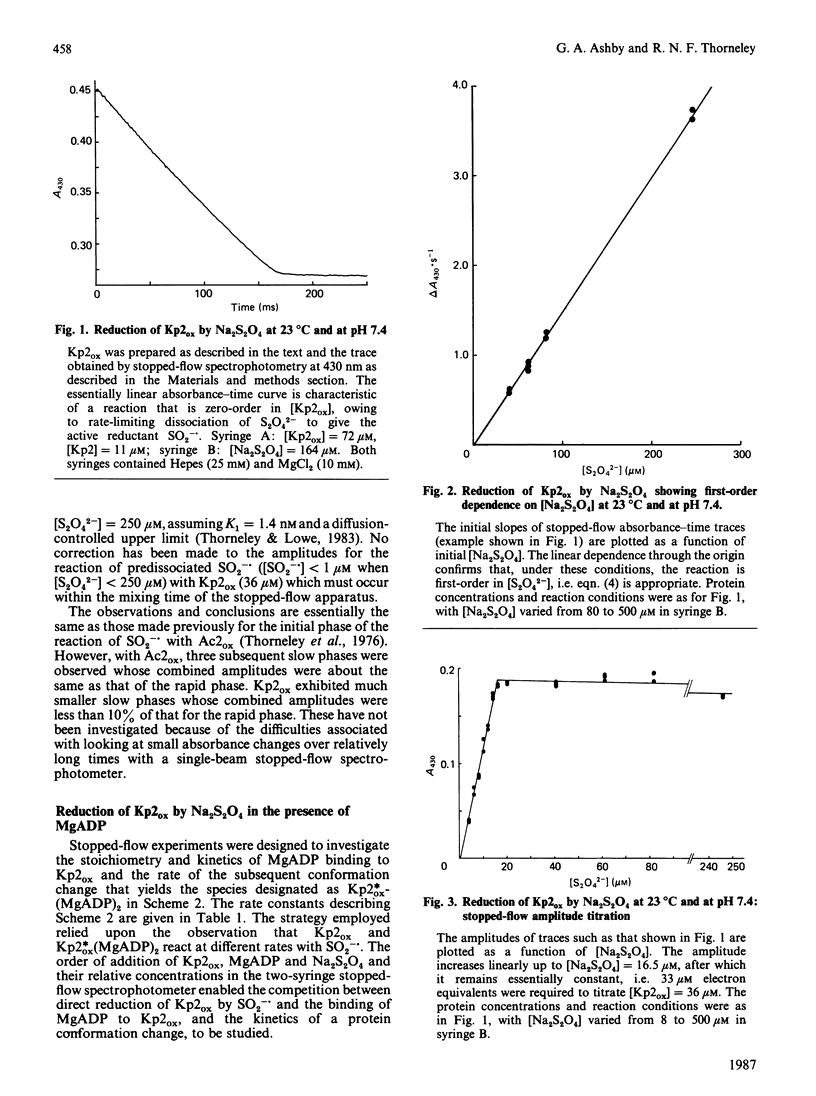
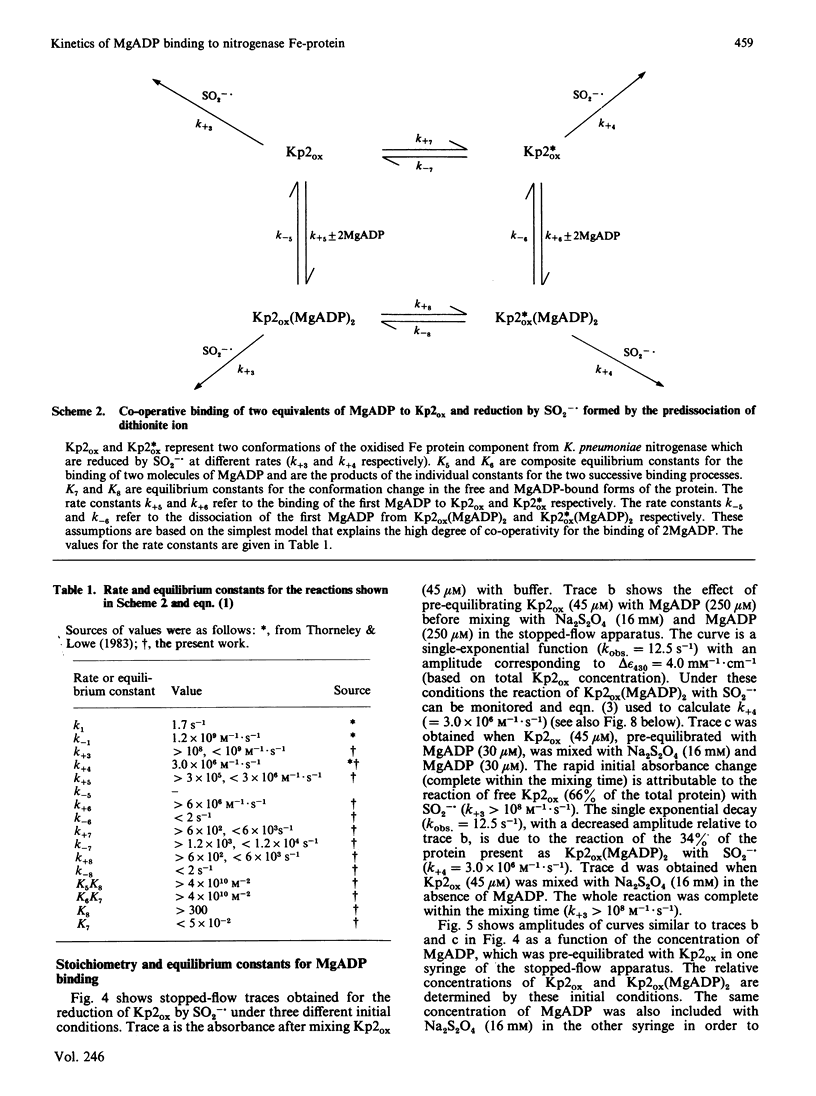
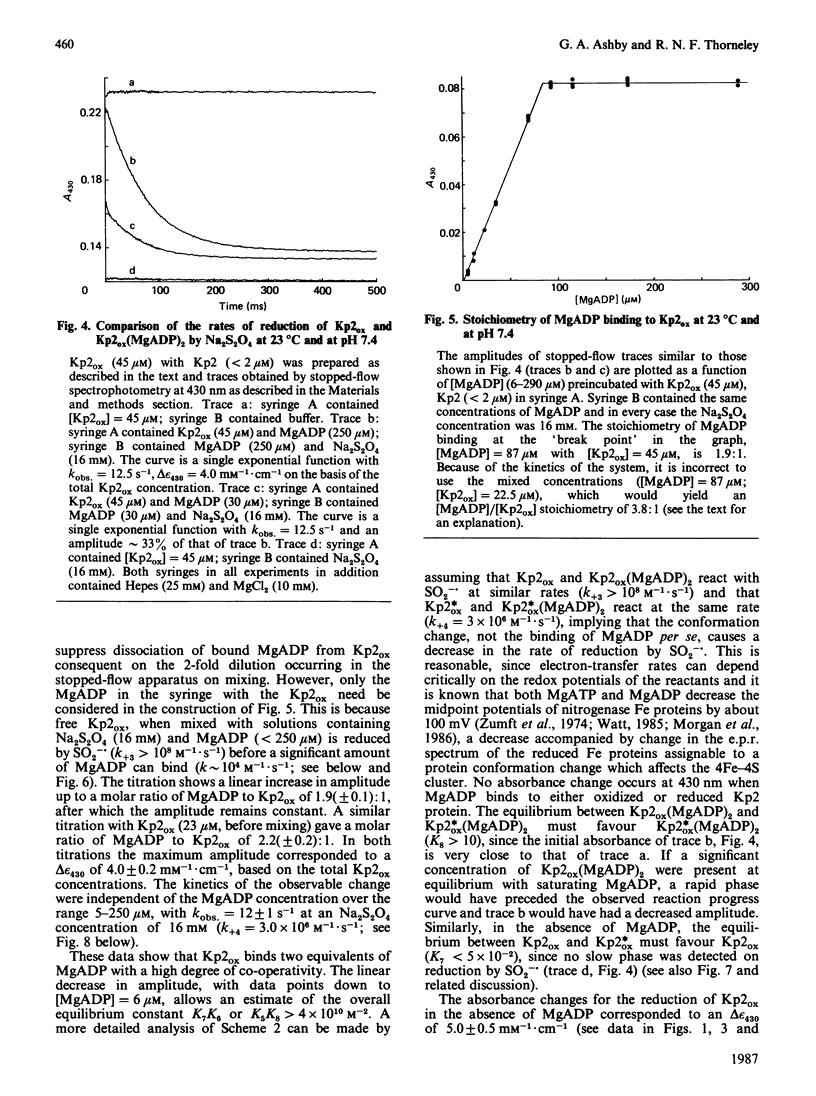
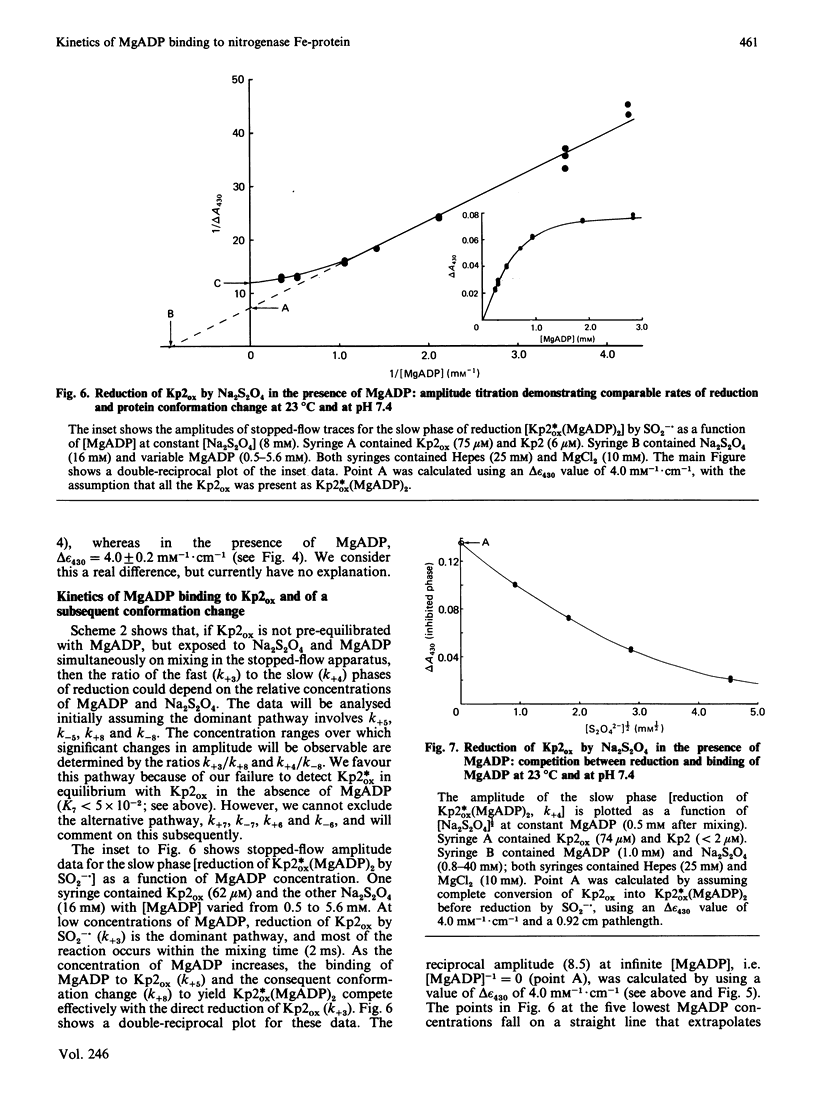
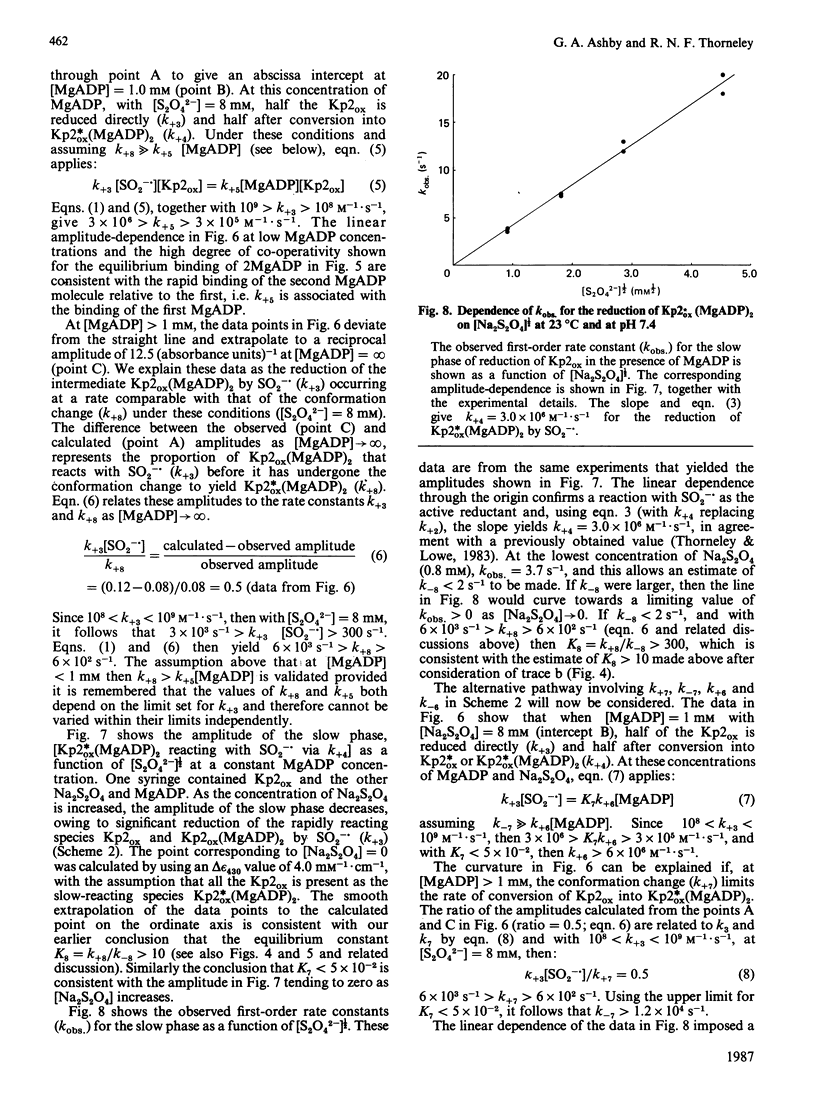
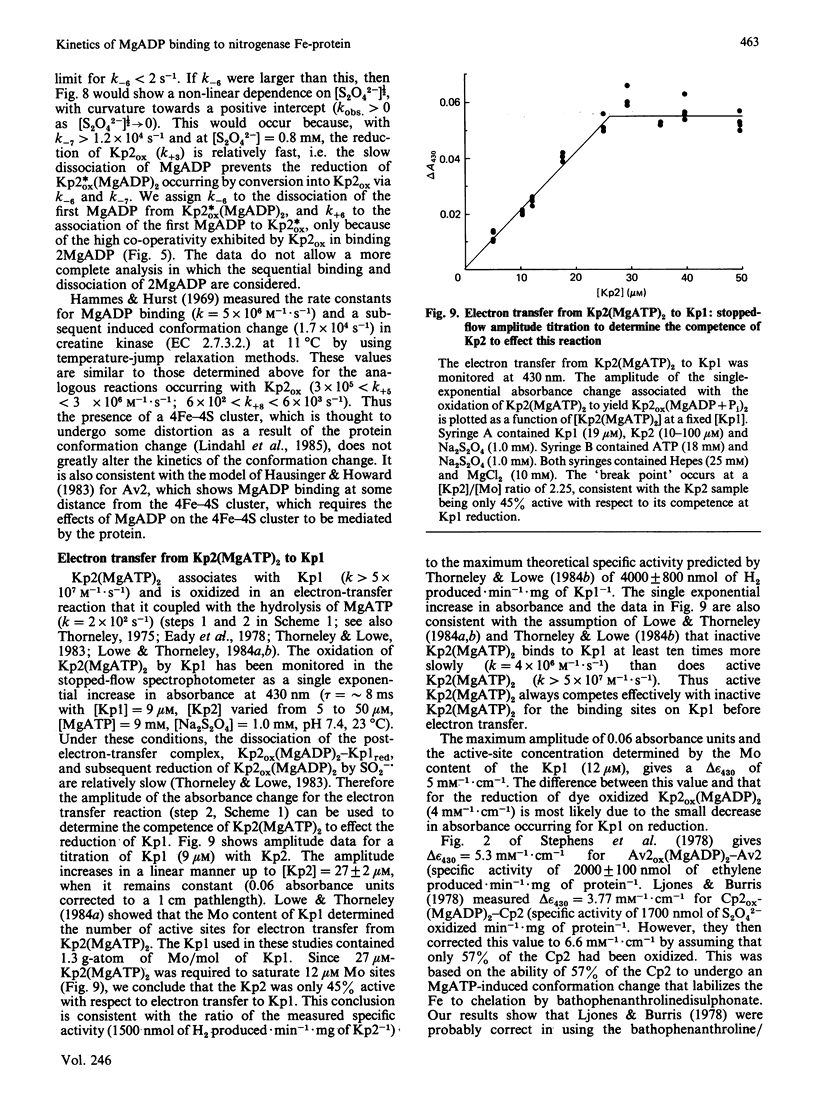
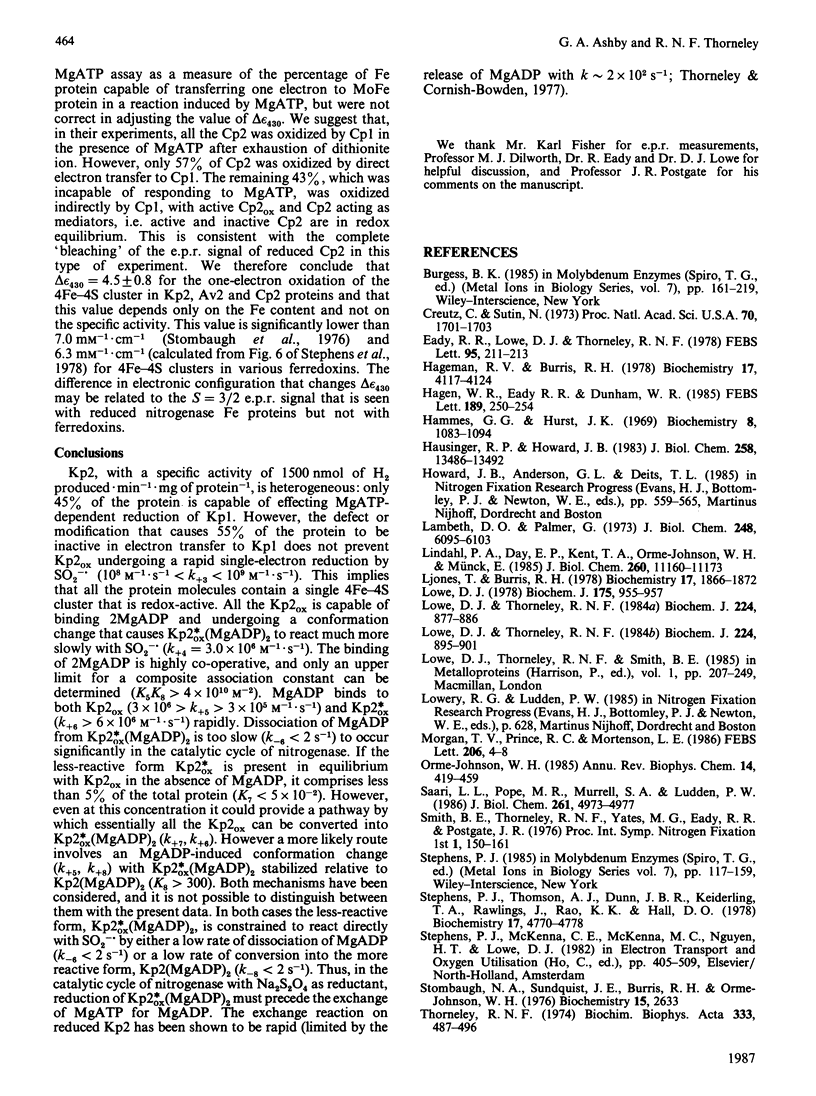
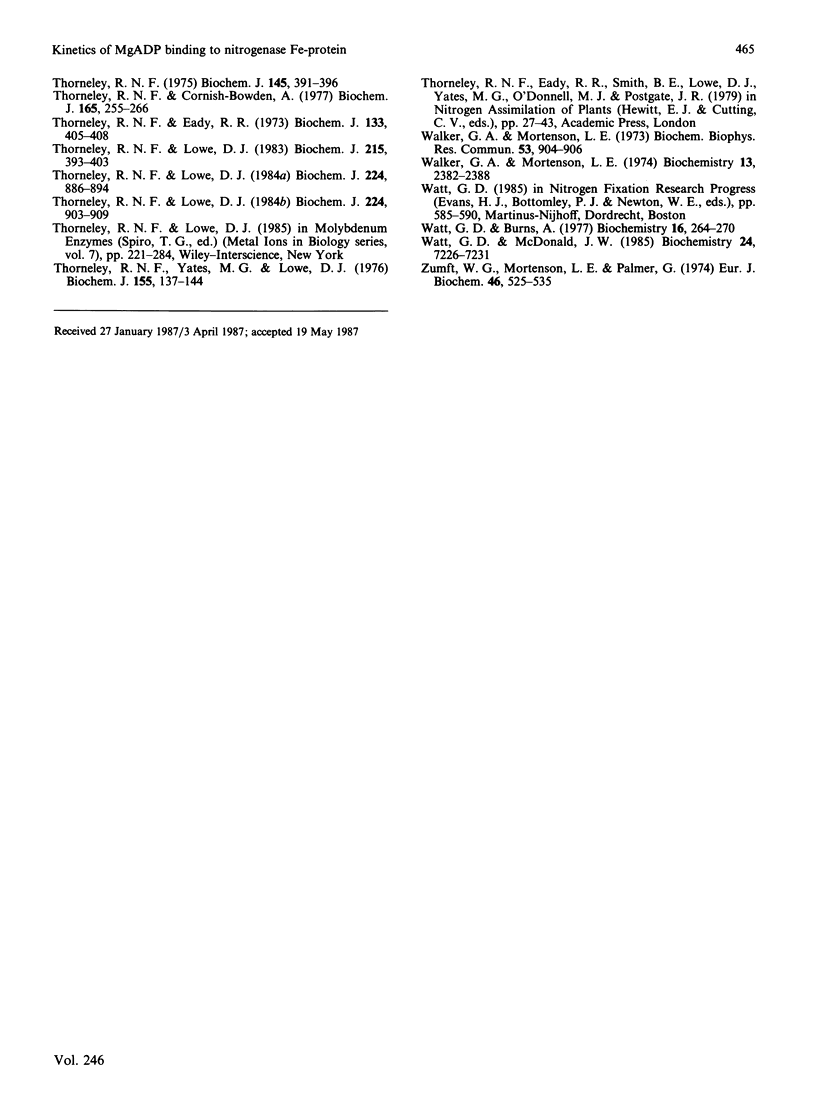
Selected References
These references are in PubMed. This may not be the complete list of references from this article.
- Creutz C., Sutin N. Reduction of ferricytochrome c by dithionite ion: electron transfer by parallel adjacent and remote pathways. Proc Natl Acad Sci U S A. 1973 Jun;70(6):1701–1703. doi: 10.1073/pnas.70.6.1701. [DOI] [PMC free article] [PubMed] [Google Scholar]
- Eady R. R., Lowe D. J., Thorneley R. N. Nitrogenase of Klebsiella pneumoniae: a pre-steady state burst of ATP hydrolysis is coupled to electron transfer between the component proteins. FEBS Lett. 1978 Nov 15;95(2):211–213. doi: 10.1016/0014-5793(78)80995-8. [DOI] [PubMed] [Google Scholar]
- Hageman R. V., Burris R. H. Kinetic studies on electron transfer and interaction between nitrogenase components from Azotobacter vinelandii. Biochemistry. 1978 Oct 3;17(20):4117–4124. doi: 10.1021/bi00613a002. [DOI] [PubMed] [Google Scholar]
- Hagen W. R., Eady R. R., Dunham W. R., Haaker H. A novel S = 3/2 EPR signal associated with native Fe-proteins of nitrogenase. FEBS Lett. 1985 Sep 23;189(2):250–254. doi: 10.1016/0014-5793(85)81033-4. [DOI] [PubMed] [Google Scholar]
- Hammes G. G., Hurst J. K. Relaxation spectra of adenosine triphosphate-creatine phosphotransferase. Biochemistry. 1969 Mar;8(3):1083–1094. doi: 10.1021/bi00831a040. [DOI] [PubMed] [Google Scholar]
- Hausinger R. P., Howard J. B. Thiol reactivity of the nitrogenase Fe-protein from Azotobacter vinelandii. J Biol Chem. 1983 Nov 25;258(22):13486–13492. [PubMed] [Google Scholar]
- Lambeth D. O., Palmer G. The kinetics and mechanism of reduction of electron transfer proteins and other compounds of biological interest by dithionite. J Biol Chem. 1973 Sep 10;248(17):6095–6103. [PubMed] [Google Scholar]
- Lindahl P. A., Day E. P., Kent T. A., Orme-Johnson W. H., Münck E. Mössbauer, EPR, and magnetization studies of the Azotobacter vinelandii Fe protein. Evidence for a [4Fe-4S]1+ cluster with spin S = 3/2. J Biol Chem. 1985 Sep 15;260(20):11160–11173. [PubMed] [Google Scholar]
- Ljones T., Burris R. H. Nitrogenase: the reaction between the Fe protein and bathophenanthrolinedisulfonate as a probe for interactions with MgATP. Biochemistry. 1978 May 16;17(10):1866–1872. doi: 10.1021/bi00603a010. [DOI] [PubMed] [Google Scholar]
- Lowe D. J. Simulation of the electron-paramagnetic-resonance spectrum of the iron-protein of nitrogenase. A prediction of the existence of a second paramagnetic centre. Biochem J. 1978 Dec 1;175(3):955–957. doi: 10.1042/bj1750955. [DOI] [PMC free article] [PubMed] [Google Scholar]
- Lowe D. J., Thorneley R. N. The mechanism of Klebsiella pneumoniae nitrogenase action. Pre-steady-state kinetics of H2 formation. Biochem J. 1984 Dec 15;224(3):877–886. doi: 10.1042/bj2240877. [DOI] [PMC free article] [PubMed] [Google Scholar]
- Lowe D. J., Thorneley R. N. The mechanism of Klebsiella pneumoniae nitrogenase action. The determination of rate constants required for the simulation of the kinetics of N2 reduction and H2 evolution. Biochem J. 1984 Dec 15;224(3):895–901. doi: 10.1042/bj2240895. [DOI] [PMC free article] [PubMed] [Google Scholar]
- Orme-Johnson W. H. Molecular basis of biological nitrogen fixation. Annu Rev Biophys Biophys Chem. 1985;14:419–459. doi: 10.1146/annurev.bb.14.060185.002223. [DOI] [PubMed] [Google Scholar]
- Saari L. L., Pope M. R., Murrell S. A., Ludden P. W. Studies on the activating enzyme for iron protein of nitrogenase from Rhodospirillum rubrum. J Biol Chem. 1986 Apr 15;261(11):4973–4977. [PubMed] [Google Scholar]
- Stephens P. J., Thomson A. J., Dunn J. B., Keiderling T. A., Rawlings J., Rao K. K., Hall D. O. Circular dichroism and magnetic circular dichroism of iron-sulfur proteins. Biochemistry. 1978 Oct 31;17(22):4770–4778. doi: 10.1021/bi00615a026. [DOI] [PubMed] [Google Scholar]
- Stombaugh N. A., Sundquist J. E., Burris R. H., Orme-Johnson W. H. Oxidation-reduction properties of several low potential iron-sulfur proteins and of methylviologen. Biochemistry. 1976 Jun 15;15(12):2633–2641. doi: 10.1021/bi00657a024. [DOI] [PubMed] [Google Scholar]
- Thorneley R. N. A convenient electrochemical preparation of reduced methyl viologen and a kinetic study of the reaction with oxygen using an anaerobic stopped-flow apparatus. Biochim Biophys Acta. 1974 Mar 26;333(3):487–496. doi: 10.1016/0005-2728(74)90133-9. [DOI] [PubMed] [Google Scholar]
- Thorneley R. N., Cornish-Bowden A. Kinetics of nitrogenase of Klebsiella pneumoniae. Heterotropic interactions between magnesium-adenosine 5'-diphosphate and magnesium-adenosine 5'-triphosphate. Biochem J. 1977 Aug 1;165(2):255–262. doi: 10.1042/bj1650255. [DOI] [PMC free article] [PubMed] [Google Scholar]
- Thorneley R. N., Eady R. R. Nitrogenase of Klebsiella pneumoniae: evidence for an adenosine triphosphate-induced association of the iron-sulphur protein. Biochem J. 1973 Jun;133(2):405–408. doi: 10.1042/bj1330405. [DOI] [PMC free article] [PubMed] [Google Scholar]
- Thorneley R. N., Lowe D. J. Nitrogenase of Klebsiella pneumoniae. Kinetics of the dissociation of oxidized iron protein from molybdenum-iron protein: identification of the rate-limiting step for substrate reduction. Biochem J. 1983 Nov 1;215(2):393–403. doi: 10.1042/bj2150393. [DOI] [PMC free article] [PubMed] [Google Scholar]
- Thorneley R. N., Lowe D. J. The mechanism of Klebsiella pneumoniae nitrogenase action. Pre-steady-state kinetics of an enzyme-bound intermediate in N2 reduction and of NH3 formation. Biochem J. 1984 Dec 15;224(3):887–894. doi: 10.1042/bj2240887. [DOI] [PMC free article] [PubMed] [Google Scholar]
- Thorneley R. N., Lowe D. J. The mechanism of Klebsiella pneumoniae nitrogenase action. Simulation of the dependences of H2-evolution rate on component-protein concentration and ratio and sodium dithionite concentration. Biochem J. 1984 Dec 15;224(3):903–909. doi: 10.1042/bj2240903. [DOI] [PMC free article] [PubMed] [Google Scholar]
- Thorneley R. N. Nitrogenase of Klebsiella pneumoniae. A stopped-flow study of magnesium-adenosine triphosphate-induce electron transfer between the compeonent proteins. Biochem J. 1975 Feb;145(2):391–396. doi: 10.1042/bj1450391. [DOI] [PMC free article] [PubMed] [Google Scholar]
- Thorneley R. N., Yates M. G., Lowe D. J. Nitrogenase of Azotobacter chroococcum. Kinetics of the reduction of oxidized iron-protein by sodium dithionite. Biochem J. 1976 Apr 1;155(1):137–144. doi: 10.1042/bj1550137. [DOI] [PMC free article] [PubMed] [Google Scholar]
- Walker G. A., Mortenson L. E. An effect of magnesium adenosine 5'-triphosphate on the structure of azoferredoxin from Clostridium pasteurianum. Biochem Biophys Res Commun. 1973 Aug 6;53(3):904–909. doi: 10.1016/0006-291x(73)90177-0. [DOI] [PubMed] [Google Scholar]
- Walker G. A., Mortenson L. E. Effect of magnesium adenosine 5'-triphosphate on the accessibility of the iron of clostridial azoferredoxin, a component of nitrogenase. Biochemistry. 1974 May 21;13(11):2382–2388. doi: 10.1021/bi00708a023. [DOI] [PubMed] [Google Scholar]
- Watt G. D., Burns A. Kinetics of dithionite ion utilization and ATP hydrolysis for reactions catalyzed by the nitrogenase complex from Azotobacter vinelandii. Biochemistry. 1977 Jan 25;16(2):264–270. doi: 10.1021/bi00621a017. [DOI] [PubMed] [Google Scholar]
- Zumft W. G., Mortenson L. E., Palmer G. Electron-paramagnetic-resonance studies on nitrogenase. Investigation of the oxidation-reduction behaviour of azoferredoxin and molybdoferredoxin with potentiometric and rapid-freeze techniques. Eur J Biochem. 1974 Aug 1;46(3):525–535. doi: 10.1111/j.1432-1033.1974.tb03646.x. [DOI] [PubMed] [Google Scholar]