Abstract
The CO2-ratios method is applied to the analysis of abnormalities of TCA (tricarboxylic acid)-cycle metabolism in AS-30D rat ascites-hepatoma cells. This method utilizes steady-state 14CO2-production rates from pairs of tracers of the same compound to evaluate TCA-cycle flux patterns. Equations are presented that quantitatively convert CO2 ratios into estimates of probability of flux through TCA-cycle-related pathways. Results of this study indicated that the ratio of 14CO2 produced from [1,4-14C]succinate to 14CO2 produced from [2,3-14C]succinate was increased by the addition of glutamine (5 mM) to the medium. An increase in the succinate CO2 ratio is quantitatively related to an increased flux of unlabelled carbon into the TCA-cycle-intermediate pools. Analysis of 14C distribution in [14C]citrate derived from [2,3-14C]succinate indicated that flux from the TCA cycle to the acetyl-CoA-derived carbons of citrate was insignificant. Thus the increased succinate CO2 ratio observed in the presence of glutamine could only result from an increased flux of carbon into the span of the TCA cycle from citrate to oxaloacetate. This result is consistent with increased flux of glutamine to alpha-oxoglutarate in the incubation medium containing exogenous glutamine. Comparison of the pyruvate CO2 ratio, steady-state 14CO2 production from [2-14C]pyruvate versus [3-14C]pyruvate, with the succinate 14CO2 ratio detected flux of pyruvate to C4 TCA-cycle intermediates in the medium containing glutamine. This result was consistent with the observation that [14C]aspartate derived from [2-14C]pyruvate was labelled in C-2 and C-3. 14C analysis also produced evidence for flux of TCA-cycle carbon to alanine. This study demonstrates that the CO2-ratios method is applicable in the analysis of the metabolic properties of AS-30D cells. This methodology has verified that the atypical TCA-cycle metabolism previously described for AS-30D-cell mitochondria occurs in intact AS-30D rat hepatoma cells.
Full text
PDF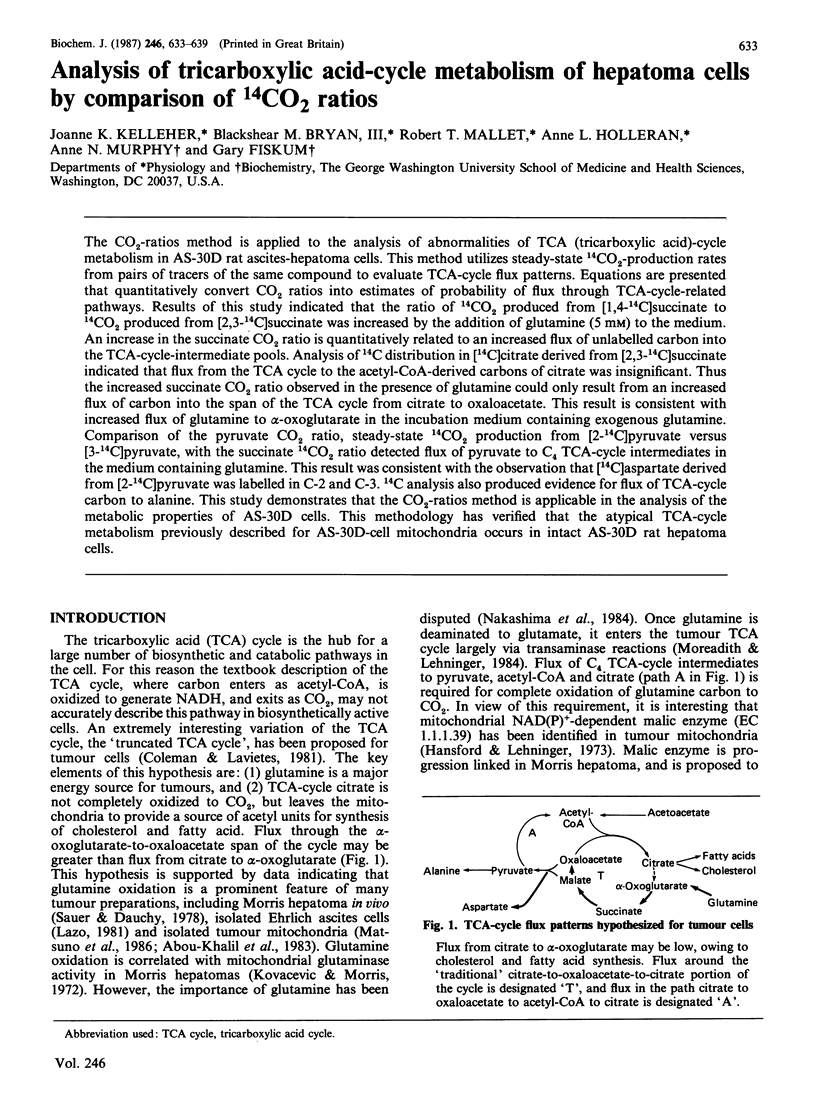
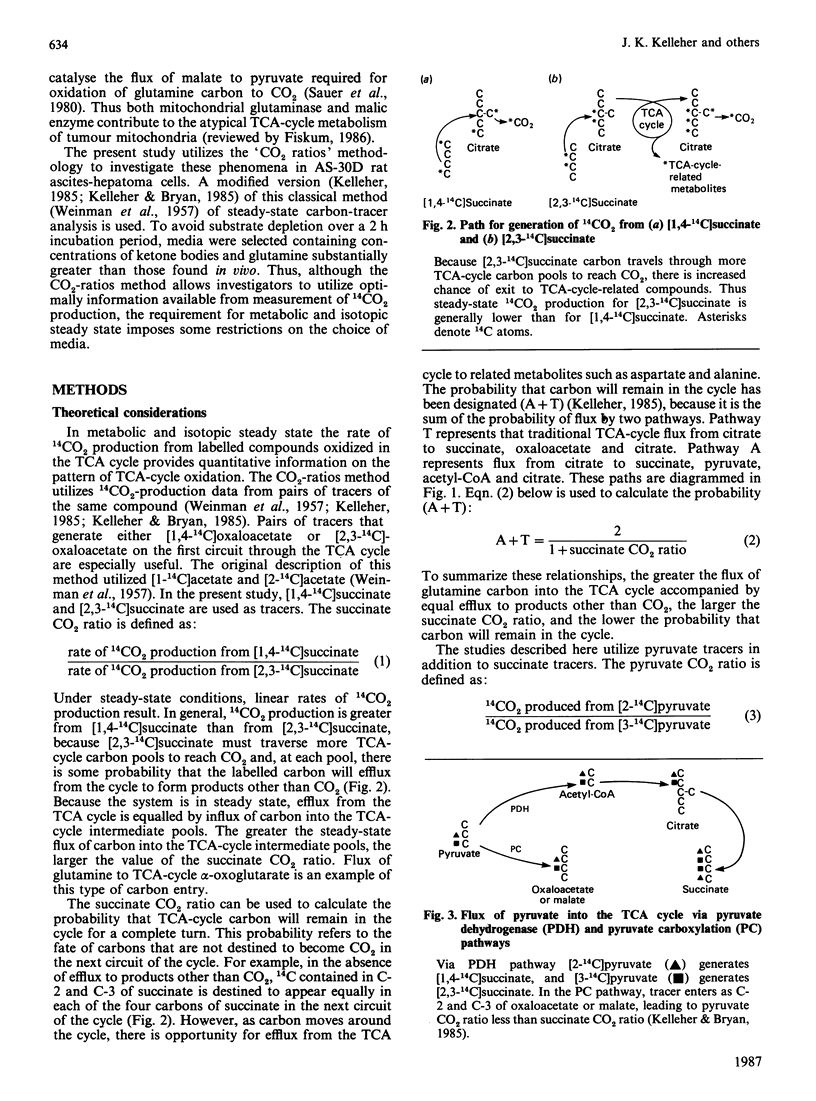
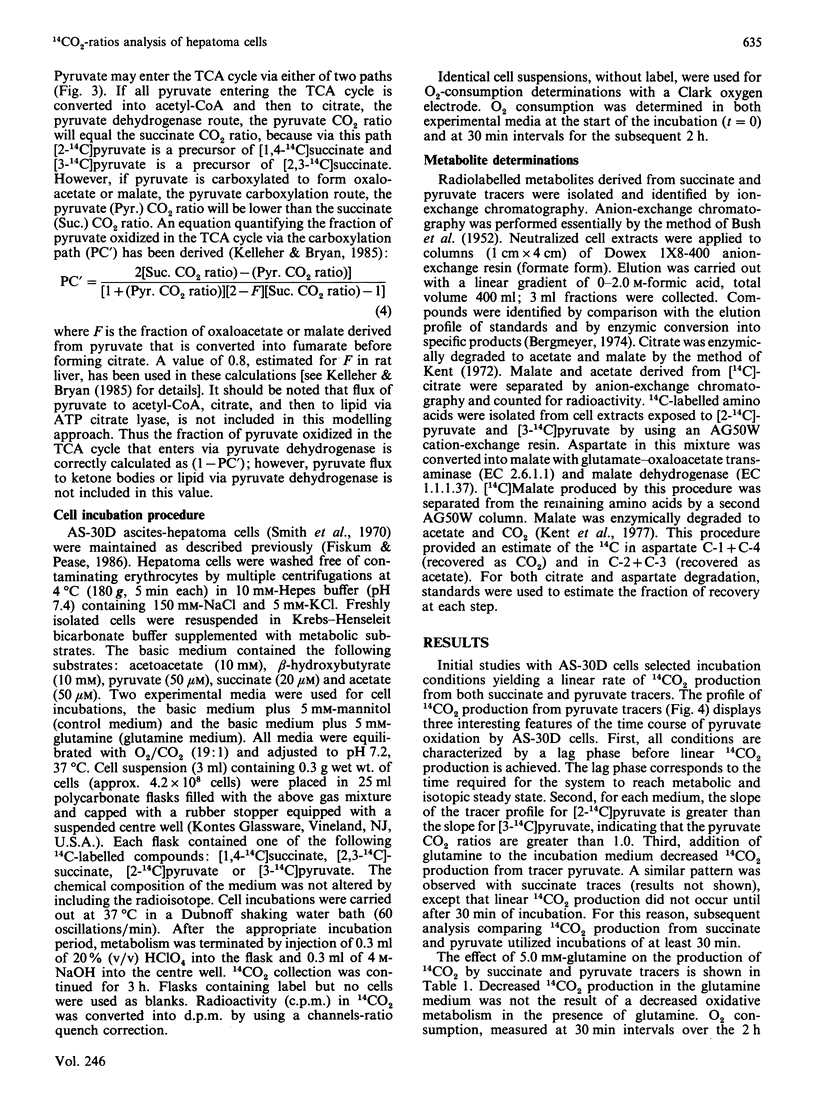
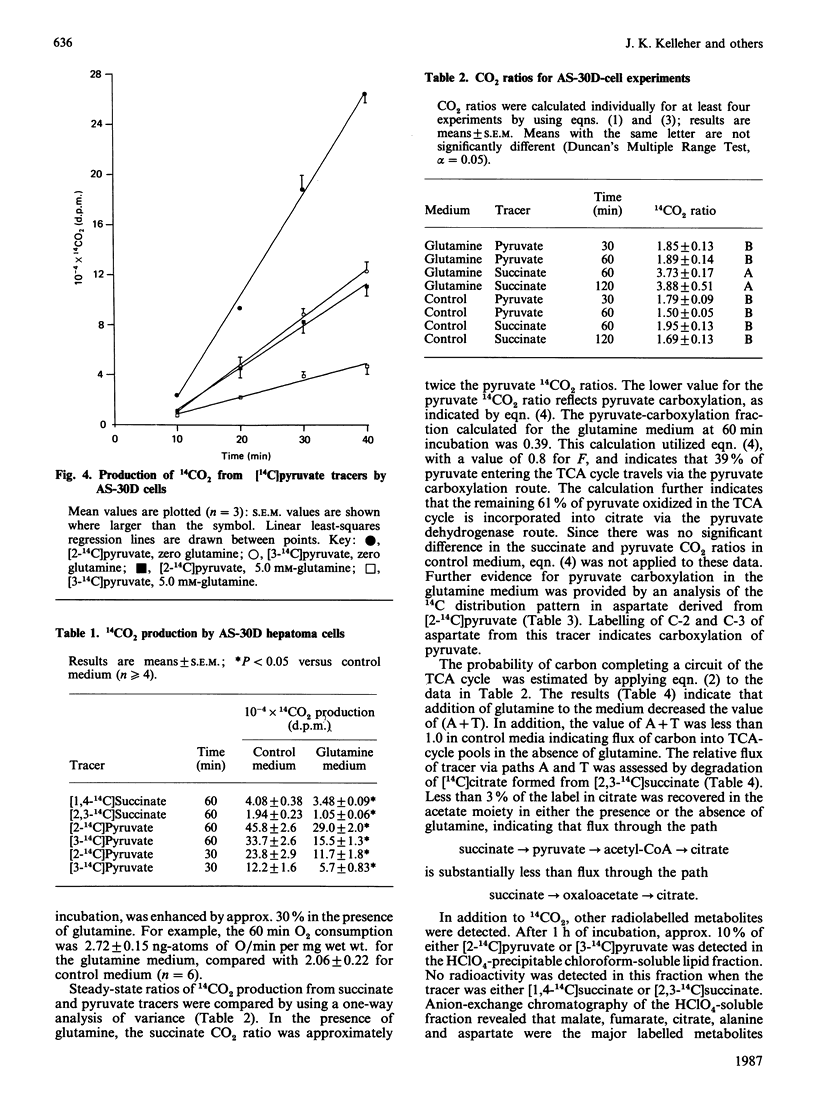
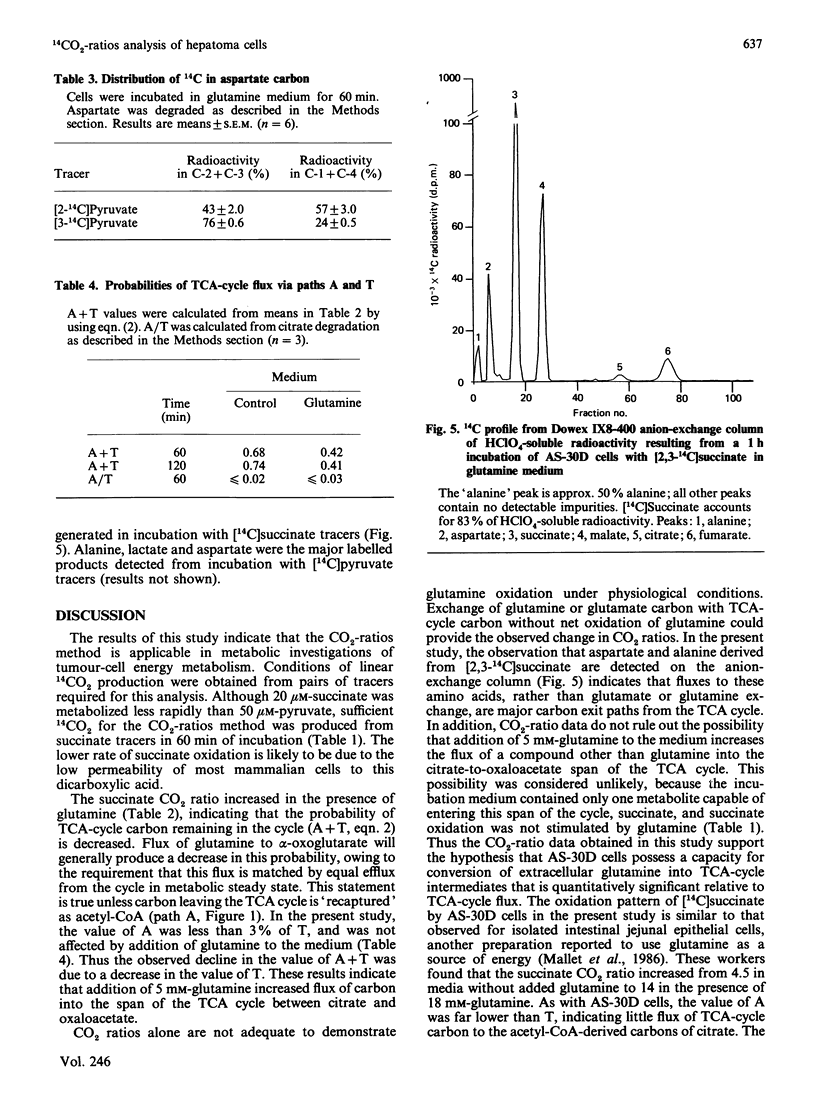
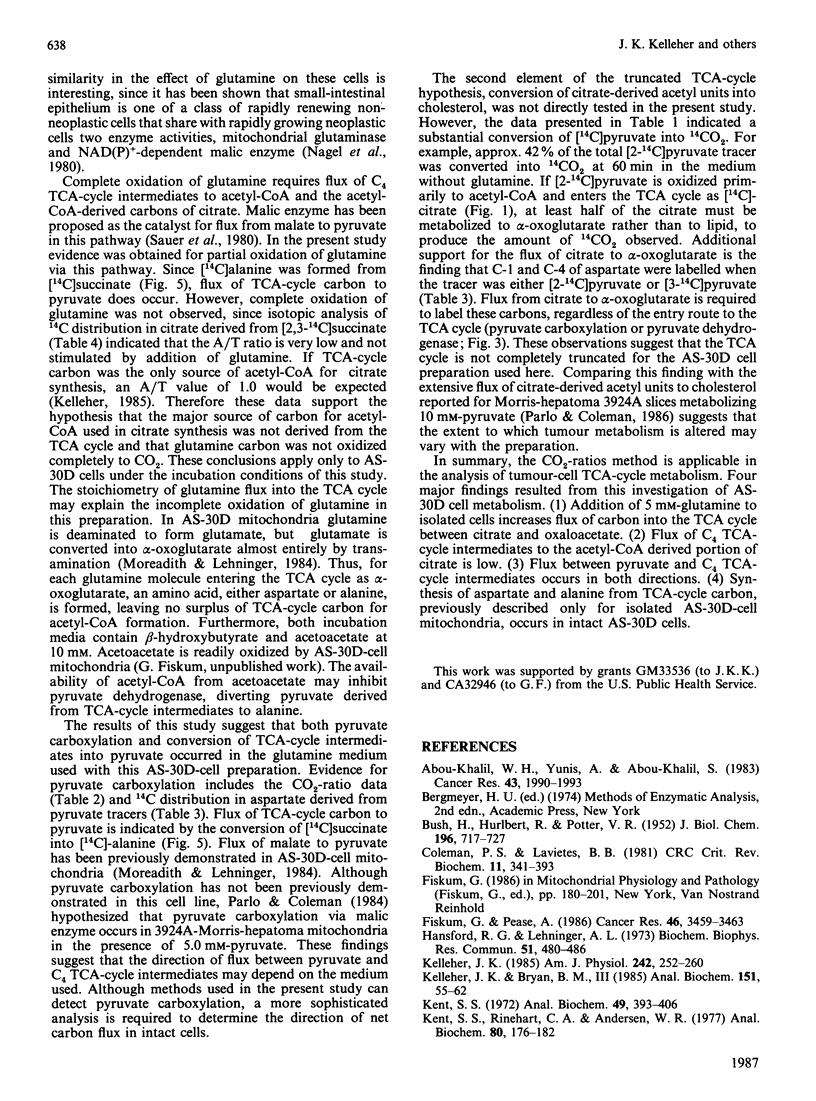
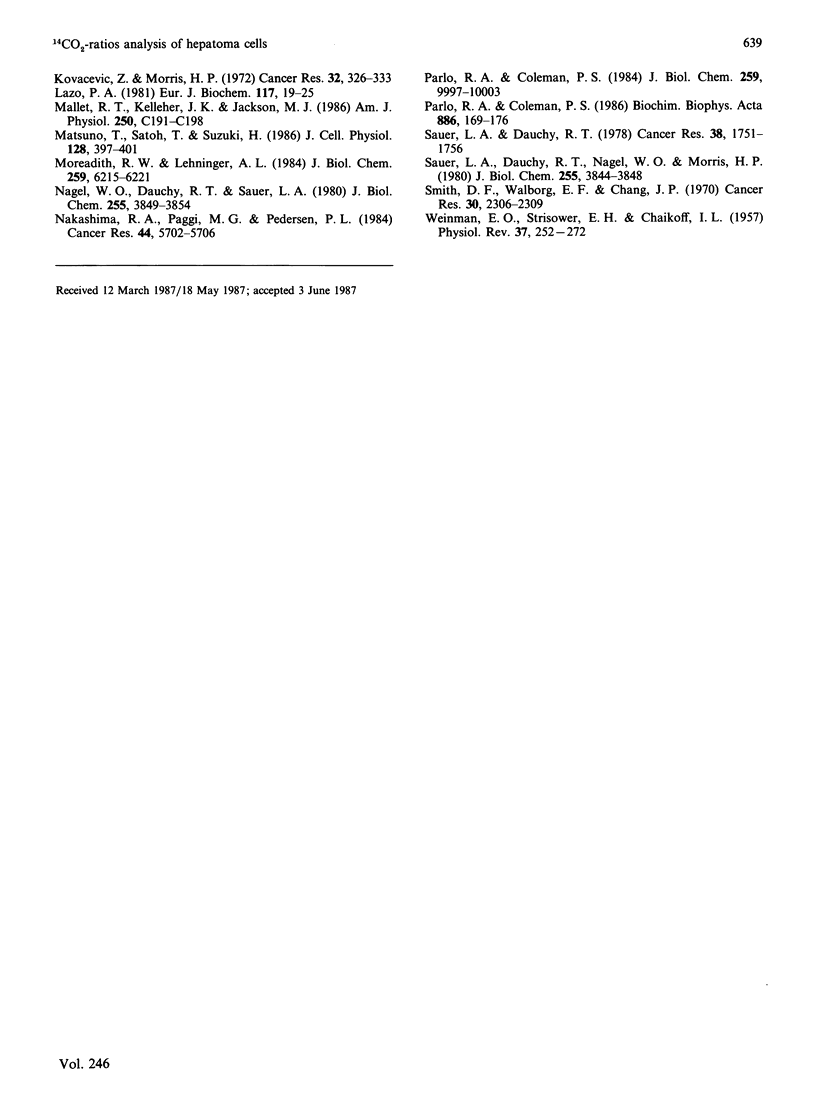
Selected References
These references are in PubMed. This may not be the complete list of references from this article.
- Abou-Khalil W. H., Yunis A. A., Abou-Khalil S. Prominent glutamine oxidation activity in mitochondria of hematopoietic tumors. Cancer Res. 1983 May;43(5):1990–1993. [PubMed] [Google Scholar]
- BUSCH H., HURLBERT R. B., POTTER V. R. Anion exchange chromatography of acids of the citric acid cycle. J Biol Chem. 1952 May;196(2):717–727. [PubMed] [Google Scholar]
- Coleman P. S., Lavietes B. B. Membrane cholesterol, tumorigenesis, and the biochemical phenotype of neoplasia. CRC Crit Rev Biochem. 1981;11(4):341–393. doi: 10.1080/10409238109104421. [DOI] [PubMed] [Google Scholar]
- Fiskum G., Pease A. Hydroperoxide-stimulated release of calcium from rat liver and AS-30D hepatoma mitochondria. Cancer Res. 1986 Jul;46(7):3459–3463. [PubMed] [Google Scholar]
- Hansford R. G., Lehninger A. L. Active oxidative decarboxylation of malate by mitochondria isolated from L-1210 ascites tumor cells. Biochem Biophys Res Commun. 1973 Mar 17;51(2):480–486. doi: 10.1016/0006-291x(73)91282-5. [DOI] [PubMed] [Google Scholar]
- Kelleher J. K., Bryan B. M., 3rd A 14CO2 ratios method for detecting pyruvate carboxylation. Anal Biochem. 1985 Nov 15;151(1):55–62. doi: 10.1016/0003-2697(85)90052-1. [DOI] [PubMed] [Google Scholar]
- Kent S. S. Complete stereochemical distribution of 14 C-isotope in citrate. Anal Biochem. 1972 Oct;49(2):393–406. doi: 10.1016/0003-2697(72)90442-3. [DOI] [PubMed] [Google Scholar]
- Kent S. S., Rinehart C. A., Andersen W. R. A method for obtaining the 14C-isotope distribution in malate(C-2,3). Anal Biochem. 1977 May 15;80(1):176–182. doi: 10.1016/0003-2697(77)90637-6. [DOI] [PubMed] [Google Scholar]
- Kovacević Z., Morris H. P. The role of glutamine in the oxidative metabolism of malignant cells. Cancer Res. 1972 Feb;32(2):326–333. [PubMed] [Google Scholar]
- Lazo P. A. Amino acids and glucose utilization by different metabolic pathways in ascites-tumour cells. Eur J Biochem. 1981 Jun;117(1):19–25. doi: 10.1111/j.1432-1033.1981.tb06297.x. [DOI] [PubMed] [Google Scholar]
- Mallet R. T., Kelleher J. K., Jackson M. J. Substrate metabolism of isolated jejunal epithelium: conservation of three-carbon units. Am J Physiol. 1986 Feb;250(2 Pt 1):C191–C198. doi: 10.1152/ajpcell.1986.250.2.C191. [DOI] [PubMed] [Google Scholar]
- Matsuno T., Satoh T., Suzuki H. Prominent glutamine oxidation activity in mitochondria of avian transplantable hepatoma induced by MC-29 virus. J Cell Physiol. 1986 Sep;128(3):397–401. doi: 10.1002/jcp.1041280308. [DOI] [PubMed] [Google Scholar]
- Moreadith R. W., Lehninger A. L. The pathways of glutamate and glutamine oxidation by tumor cell mitochondria. Role of mitochondrial NAD(P)+-dependent malic enzyme. J Biol Chem. 1984 May 25;259(10):6215–6221. [PubMed] [Google Scholar]
- Nagel W. O., Dauchy R. T., Sauer L. A. Mitochondrial malic enzymes. An association between NAD(P)+-dependent malic enzyme and cell renewal in Sprague-Dawley rat tissues. J Biol Chem. 1980 May 10;255(9):3849–3854. [PubMed] [Google Scholar]
- Nakashima R. A., Paggi M. G., Pedersen P. L. Contributions of glycolysis and oxidative phosphorylation to adenosine 5'-triphosphate production in AS-30D hepatoma cells. Cancer Res. 1984 Dec;44(12 Pt 1):5702–5706. [PubMed] [Google Scholar]
- Parlo R. A., Coleman P. S. Continuous pyruvate carbon flux to newly synthesized cholesterol and the suppressed evolution of pyruvate-generated CO2 in tumors: further evidence for a persistent truncated Krebs cycle in hepatomas. Biochim Biophys Acta. 1986 Apr 29;886(2):169–176. doi: 10.1016/0167-4889(86)90134-5. [DOI] [PubMed] [Google Scholar]
- Parlo R. A., Coleman P. S. Enhanced rate of citrate export from cholesterol-rich hepatoma mitochondria. The truncated Krebs cycle and other metabolic ramifications of mitochondrial membrane cholesterol. J Biol Chem. 1984 Aug 25;259(16):9997–10003. [PubMed] [Google Scholar]
- Sauer L. A., Dauchy R. T. Identification and properties of the nicotinamide adenine dinucleotide (phosphate)+-dependent malic enzyme in mouse ascites tumor mitochondria. Cancer Res. 1978 Jun;38(6):1751–1756. [PubMed] [Google Scholar]
- Sauer L. A., Dauchy R. T., Nagel W. O., Morris H. P. Mitochondrial malic enzymes. Mitochondrial NAD(P)+-dependent malic enzyme activity and malate-dependent pyruvate formation are progression-linked in Morris hepatomas. J Biol Chem. 1980 May 10;255(9):3844–3848. [PubMed] [Google Scholar]
- Smith D. F., Walborg E. F., Jr, Chang J. P. Establishment of a transplantable ascites variant of a rat hepatoma induced by 3'-methyl-4-dimethylaminoazobenzene. Cancer Res. 1970 Sep;30(9):2306–2309. [PubMed] [Google Scholar]
- WEINMAN E. O., STRISOWER E. H., CHAIKOFF I. L. Conversion of fatty acids to carbohydrate; application of isotopes to this problem and role of the Krebs cycle as a synthetic pathway. Physiol Rev. 1957 Apr;37(2):252–272. doi: 10.1152/physrev.1957.37.2.252. [DOI] [PubMed] [Google Scholar]