Abstract
An organism growing at 88 degrees C that closely resembles Desulfurococcus mucosus produced a single extracellular proteinase. We have purified this enzyme and carried out a preliminary characterization. The proteinase, which is a serine-type enzyme, had a molecular mass of 52,000 Da by SDS/polyacrylamide-gel electrophoresis, but only 10,000-13,000 Da by gel-permeation chromatography. Molecular mass values from sucrose-gradient centrifugation were of the same order as those from SDS/polyacrylamide-gel electrophoresis. It had an isoelectric point of 8.7, and was inhibited by di-isopropyl phosphorofluoridate, phenylmethanesulphonyl fluoride and chymostatin. Substrate-specificity studies suggested a possible preference for hydrophobic residues on the C-terminal side of the splitting point. The thermostability of this enzyme is probably greater than any other reported proteinase (t1/2 at 95 degrees C, 70-90 min; t1/2 at 105 degrees C, 8-9 min). Ca2+ chelation does not appear to be implicated in stabilization of the protein structure. The stability of the Desulfurococcus proteinase was not greatly affected by the presence of reducing reagents (e.g. dithiothreitol), some chaotropic agents (e.g. NaSCN) and some detergents, but activity was lost rapidly at 95 degrees C in the presence of the oxidizing agent NaBO3. Proteolytic activity was readily detected at temperatures up to and including 125 degrees C, although denaturation was very rapid above 115 degrees C. A number of Figures supporting some of the findings reported in this paper have been deposited in supplement SUP 50137 (14 pages) at the British Library Lending Division, Boston Spa, Wetherby, West Yorkshire LS23 7BQ, U.K., from whom copies may be obtained on the terms indicated in Biochem. J. (1987) 241, 5.
Full text
PDF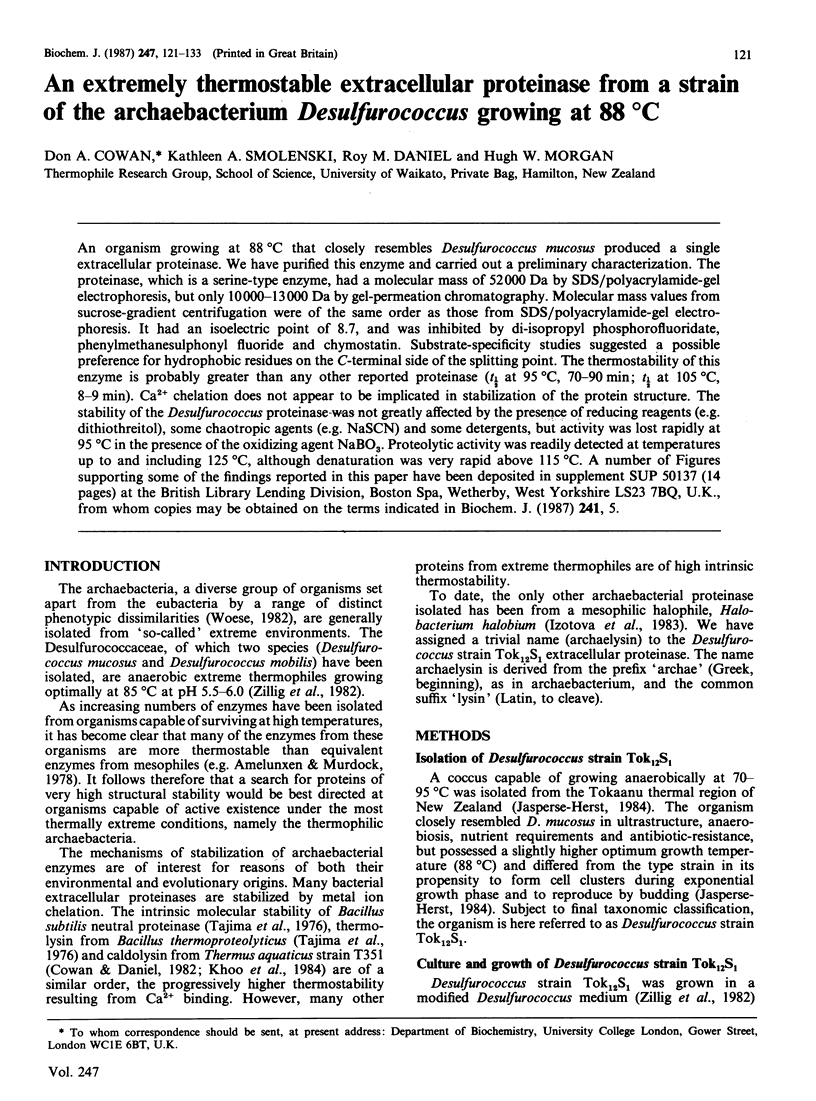
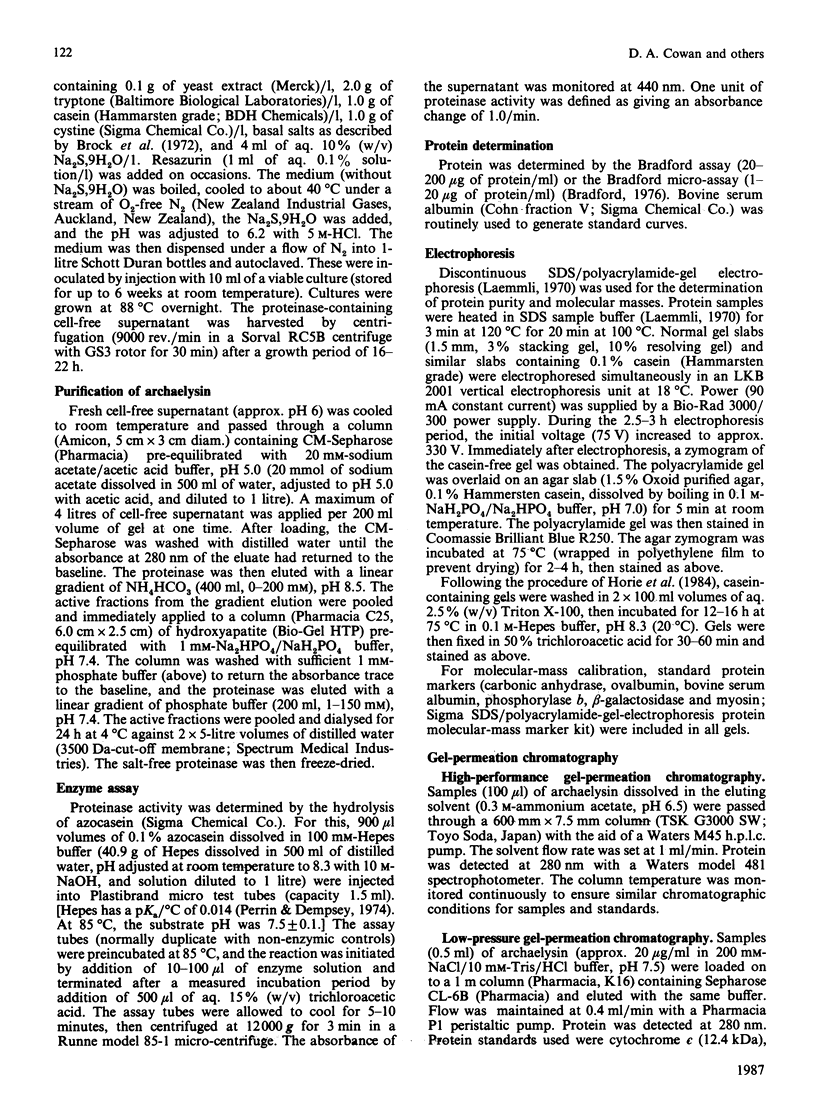
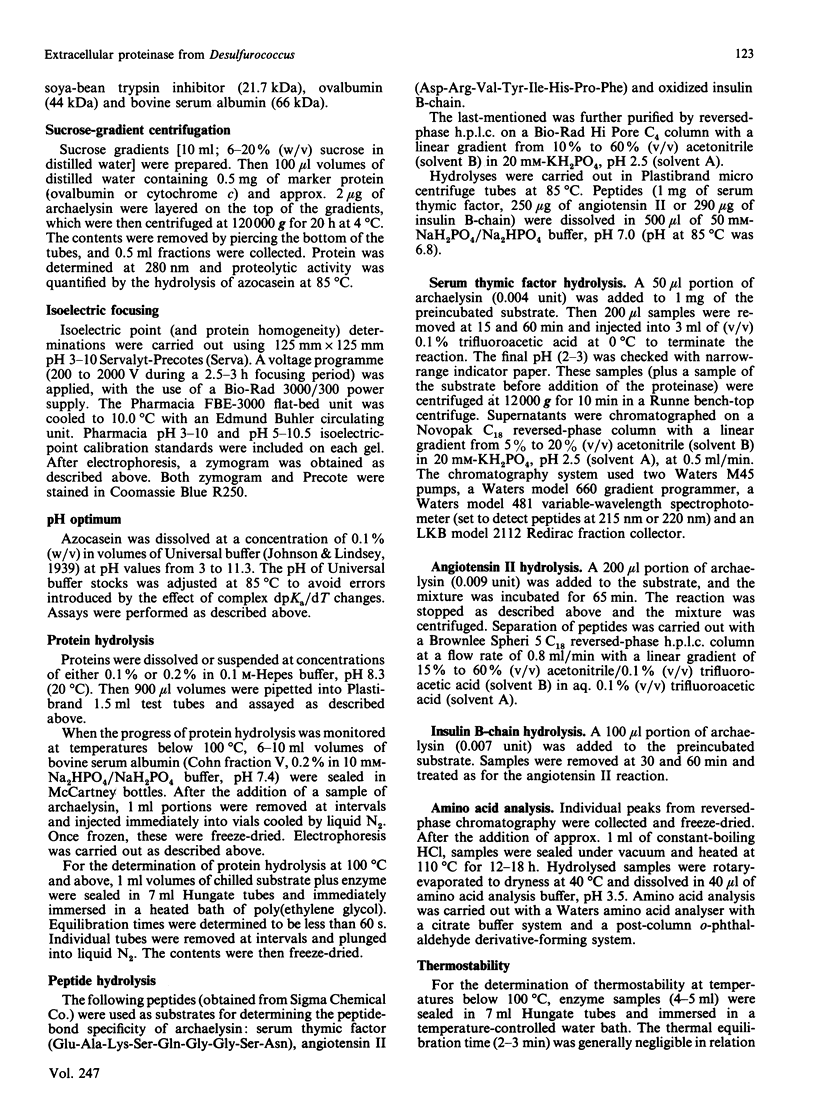
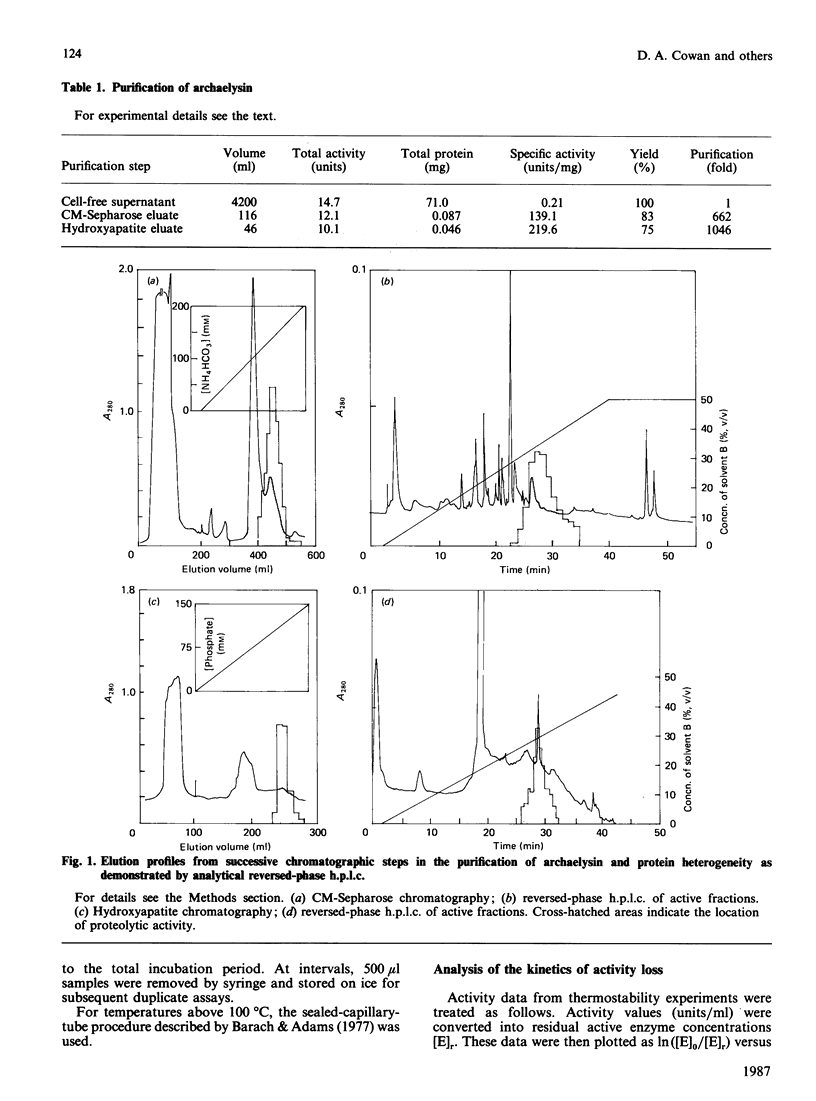
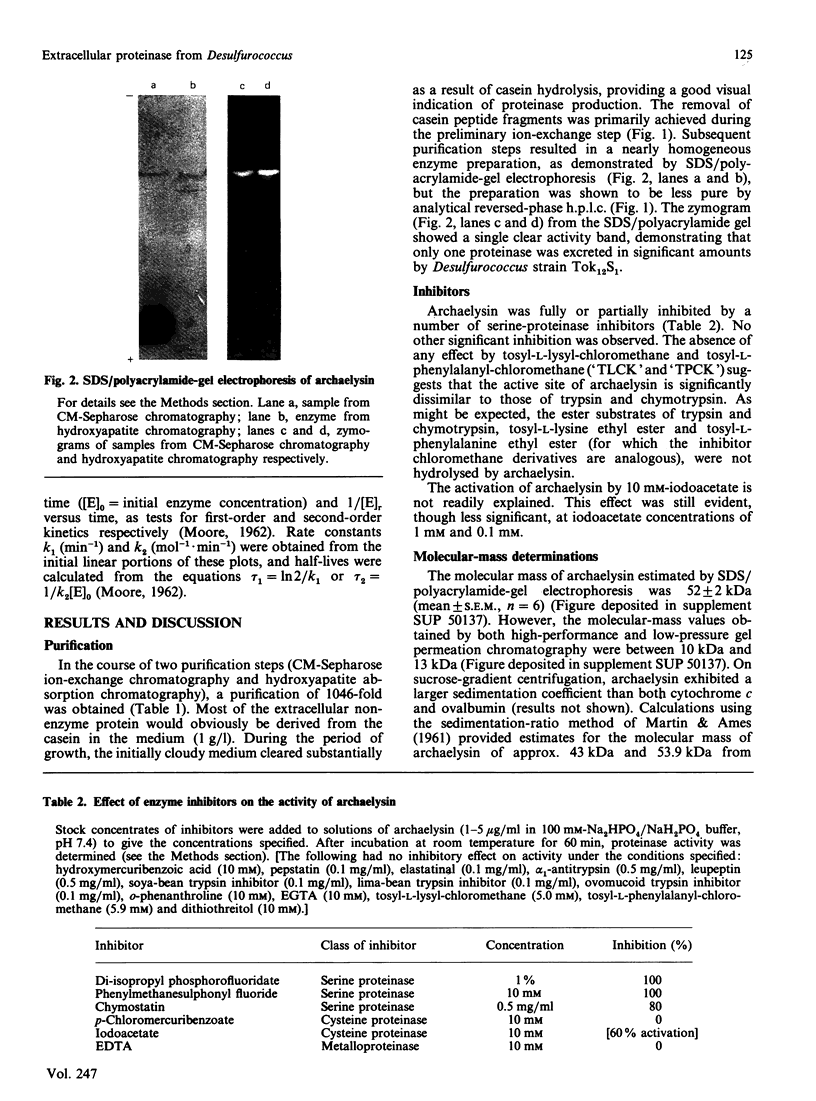
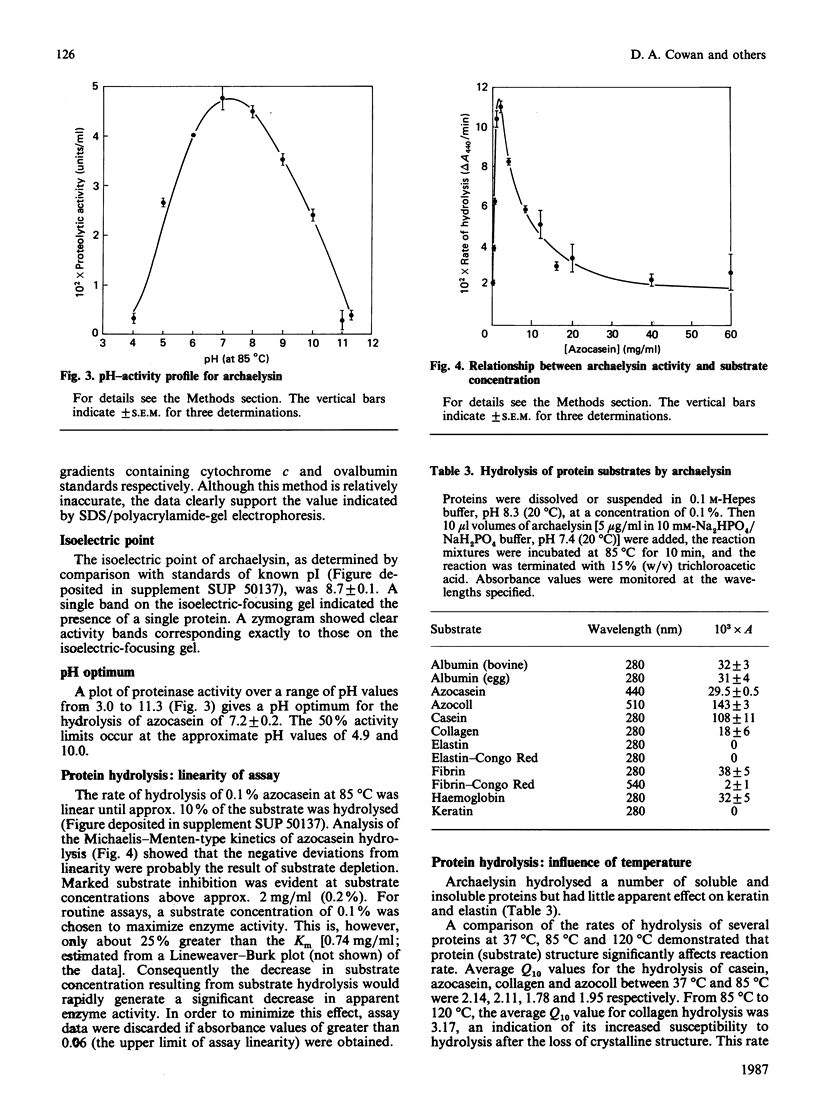
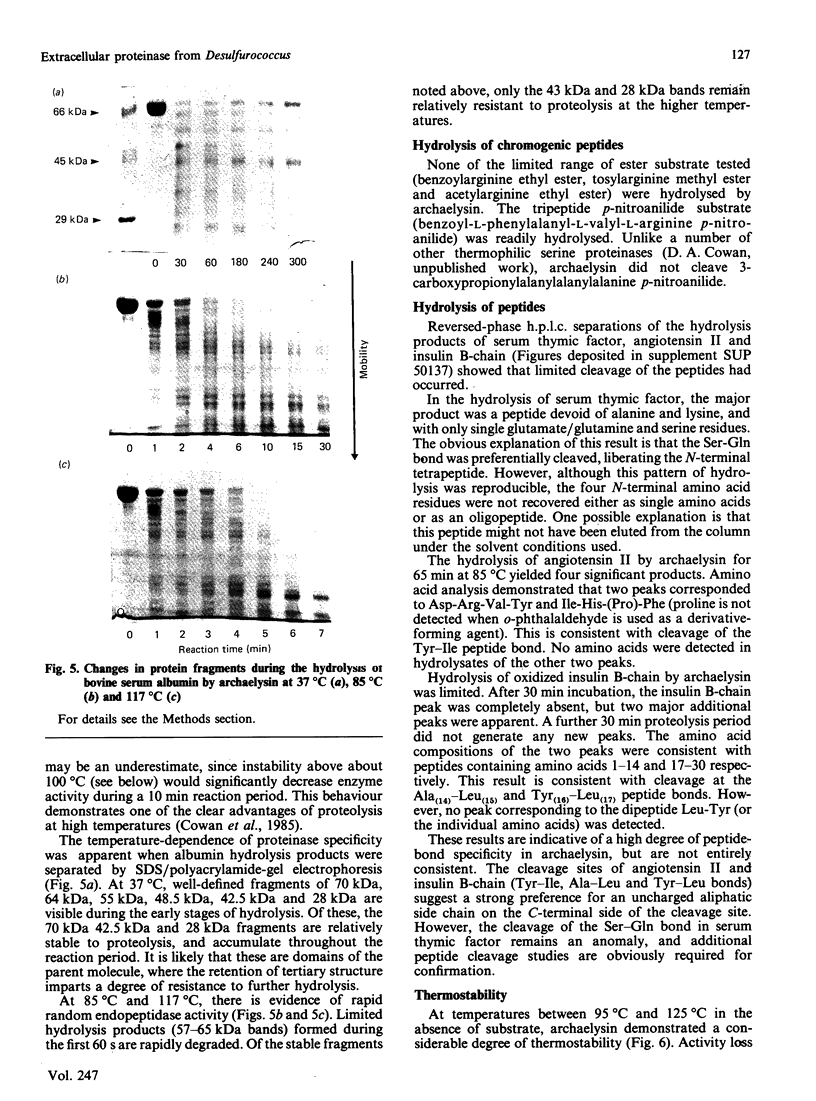
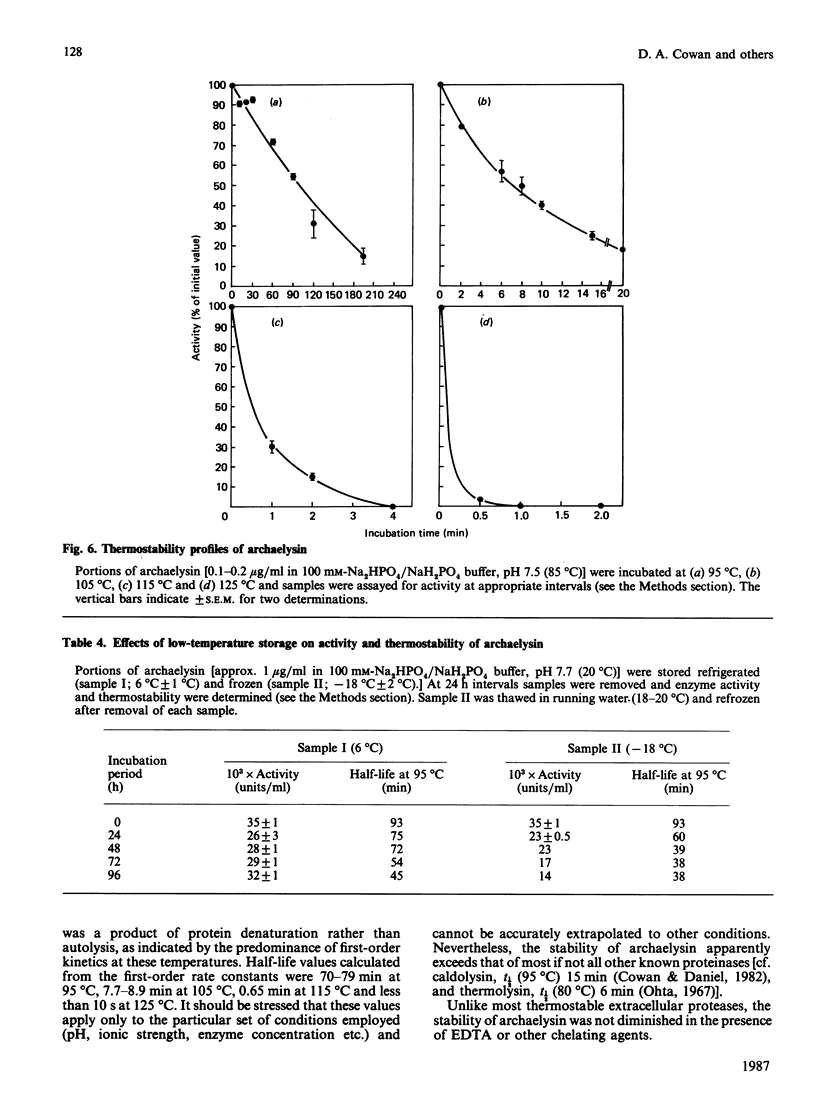
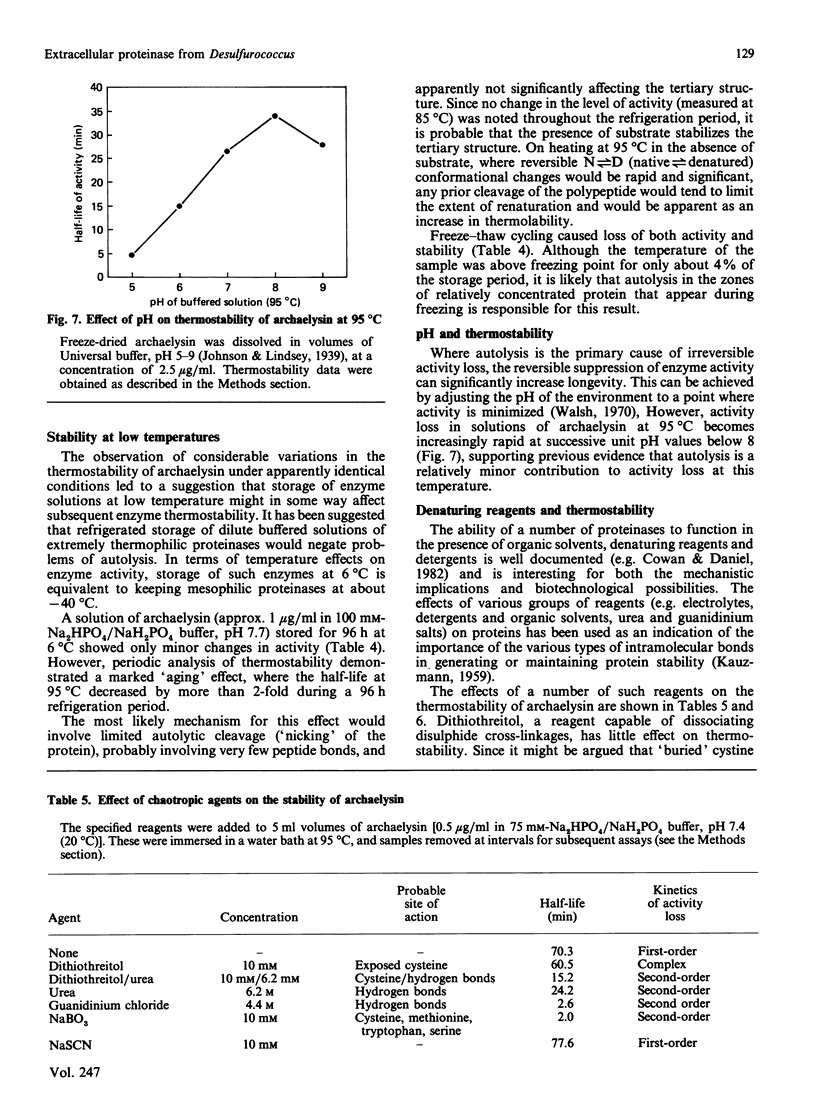
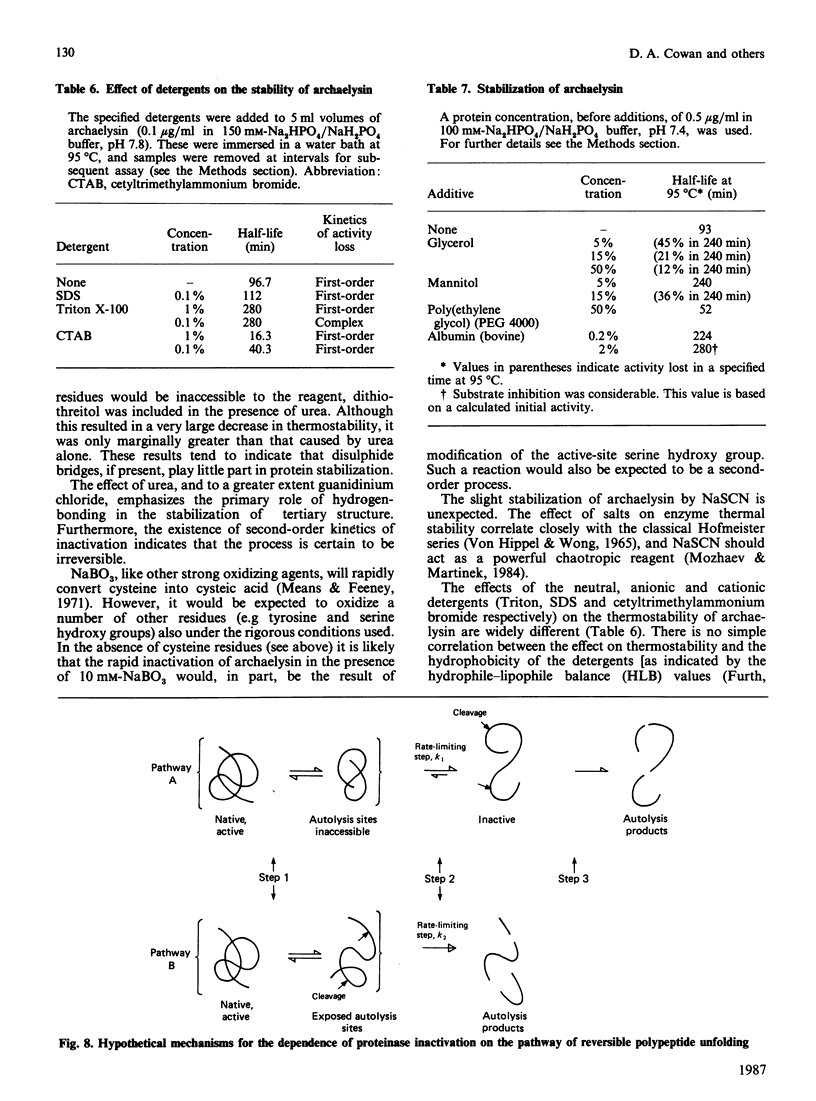
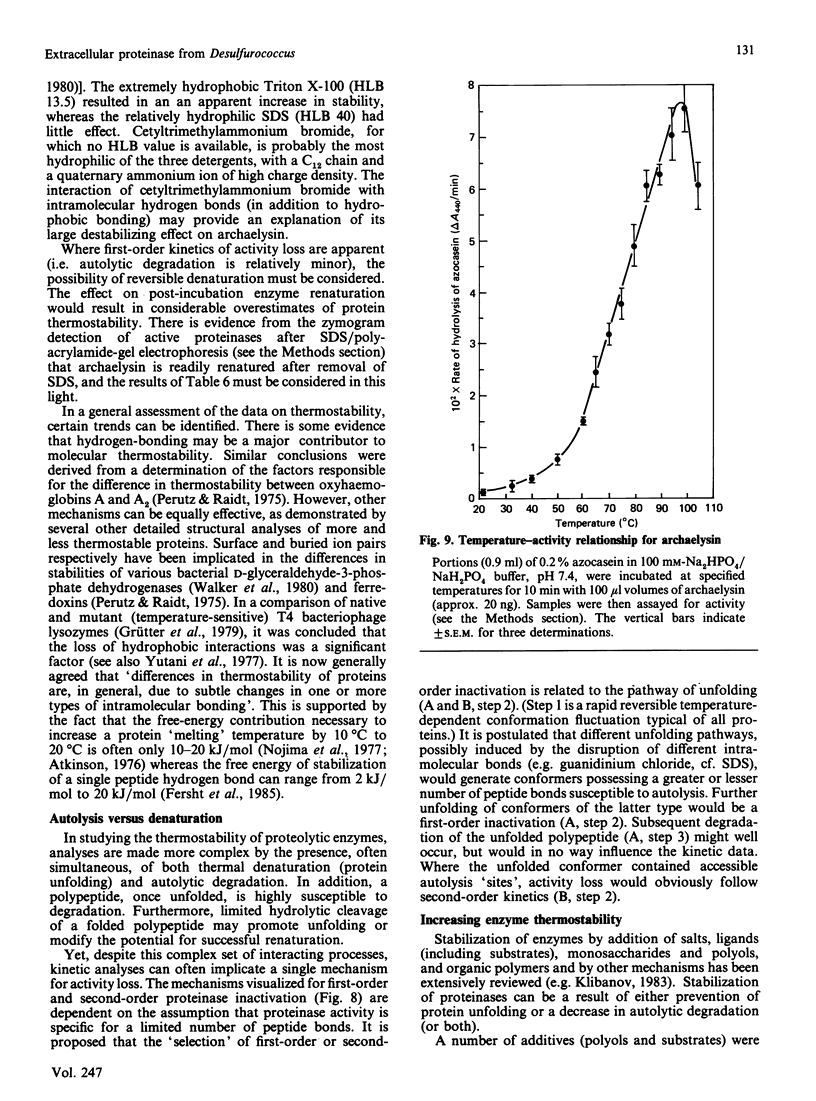
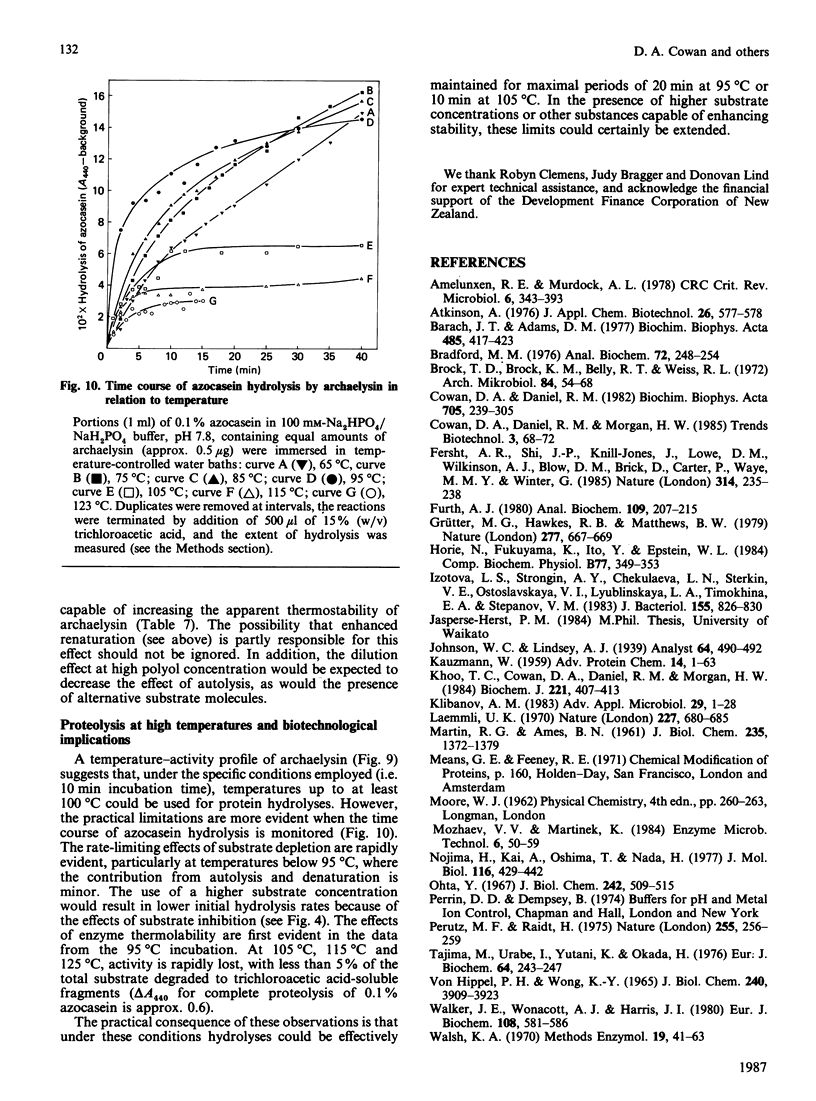
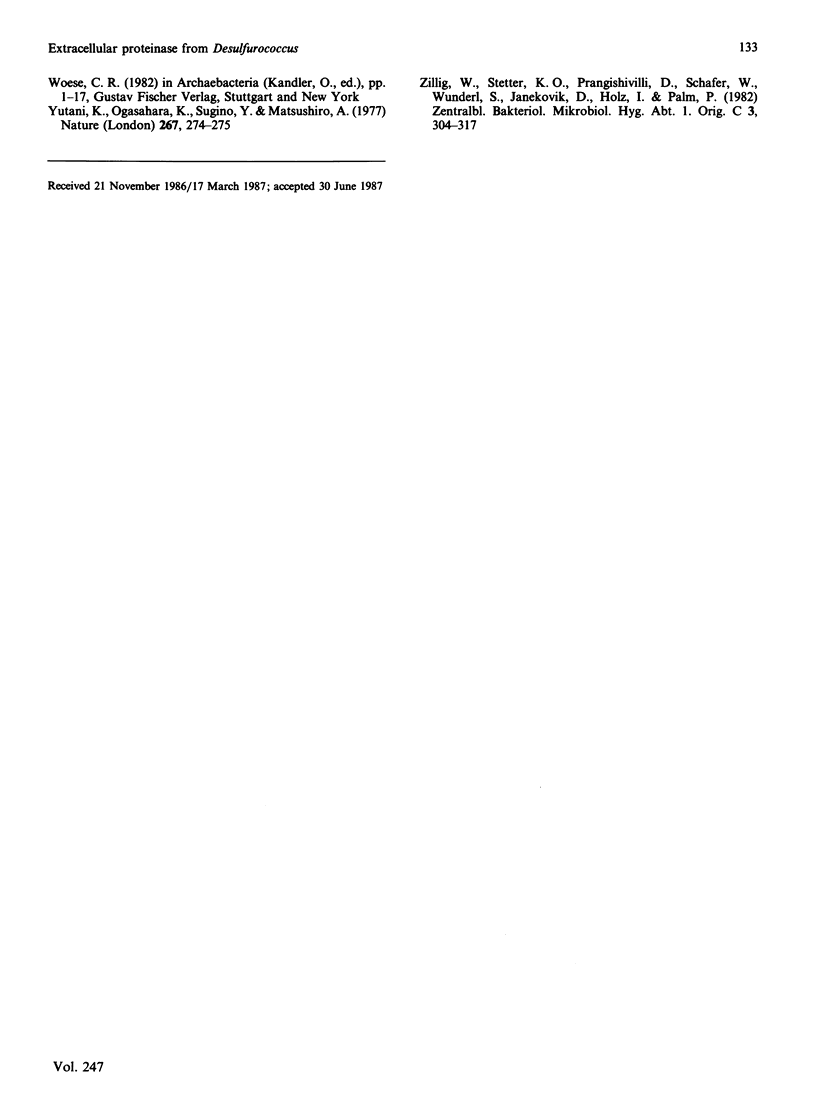
Images in this article
Selected References
These references are in PubMed. This may not be the complete list of references from this article.
- Amelunxen R. E., Murdock A. L. Mechanisms of thermophily. CRC Crit Rev Microbiol. 1978;6(4):343–393. doi: 10.3109/10408417809090626. [DOI] [PubMed] [Google Scholar]
- Barach J. T., Adams D. M. Thermostability at ultrahigh temperatures of thermolysin and a protease from a psychrotrophic Pseudomonas. Biochim Biophys Acta. 1977 Dec 8;485(2):417–423. doi: 10.1016/0005-2744(77)90177-2. [DOI] [PubMed] [Google Scholar]
- Bradford M. M. A rapid and sensitive method for the quantitation of microgram quantities of protein utilizing the principle of protein-dye binding. Anal Biochem. 1976 May 7;72:248–254. doi: 10.1006/abio.1976.9999. [DOI] [PubMed] [Google Scholar]
- Brock T. D., Brock K. M., Belly R. T., Weiss R. L. Sulfolobus: a new genus of sulfur-oxidizing bacteria living at low pH and high temperature. Arch Mikrobiol. 1972;84(1):54–68. doi: 10.1007/BF00408082. [DOI] [PubMed] [Google Scholar]
- Cowan D. A., Daniel R. M. Purification and some properties of an extracellular protease (caldolysin) from an extreme thermophile. Biochim Biophys Acta. 1982 Aug 10;705(3):293–305. doi: 10.1016/0167-4838(82)90251-5. [DOI] [PubMed] [Google Scholar]
- Fersht A. R., Shi J. P., Knill-Jones J., Lowe D. M., Wilkinson A. J., Blow D. M., Brick P., Carter P., Waye M. M., Winter G. Hydrogen bonding and biological specificity analysed by protein engineering. Nature. 1985 Mar 21;314(6008):235–238. doi: 10.1038/314235a0. [DOI] [PubMed] [Google Scholar]
- Furth A. J. Removing unbound detergent from hydrophobic proteins. Anal Biochem. 1980 Dec;109(2):207–215. doi: 10.1016/0003-2697(80)90638-7. [DOI] [PubMed] [Google Scholar]
- Grütter M. G., Hawkes R. B., Matthews B. W. Molecular basis of thermostability in the lysozyme from bacteriophage T4. Nature. 1979 Feb 22;277(5698):667–669. doi: 10.1038/277667a0. [DOI] [PubMed] [Google Scholar]
- Horie N., Fukuyama K., Ito Y., Epstein W. L. Detection and characterization of epidermal proteinases by polyacrylamide gel electrophoresis. Comp Biochem Physiol B. 1984;77(2):349–353. doi: 10.1016/0305-0491(84)90342-0. [DOI] [PubMed] [Google Scholar]
- Izotova L. S., Strongin A. Y., Chekulaeva L. N., Sterkin V. E., Ostoslavskaya V. I., Lyublinskaya L. A., Timokhina E. A., Stepanov V. M. Purification and properties of serine protease from Halobacterium halobium. J Bacteriol. 1983 Aug;155(2):826–830. doi: 10.1128/jb.155.2.826-830.1983. [DOI] [PMC free article] [PubMed] [Google Scholar]
- KAUZMANN W. Some factors in the interpretation of protein denaturation. Adv Protein Chem. 1959;14:1–63. doi: 10.1016/s0065-3233(08)60608-7. [DOI] [PubMed] [Google Scholar]
- Khoo T. C., Cowan D. A., Daniel R. M., Morgan H. W. Interactions of calcium and other metal ions with caldolysin, the thermostable proteinase from Thermus aquaticus strain T351. Biochem J. 1984 Jul 15;221(2):407–413. doi: 10.1042/bj2210407. [DOI] [PMC free article] [PubMed] [Google Scholar]
- Klibanov A. M. Stabilization of enzymes against thermal inactivation. Adv Appl Microbiol. 1983;29:1–28. doi: 10.1016/s0065-2164(08)70352-6. [DOI] [PubMed] [Google Scholar]
- Laemmli U. K. Cleavage of structural proteins during the assembly of the head of bacteriophage T4. Nature. 1970 Aug 15;227(5259):680–685. doi: 10.1038/227680a0. [DOI] [PubMed] [Google Scholar]
- MARTIN R. G., AMES B. N. A method for determining the sedimentation behavior of enzymes: application to protein mixtures. J Biol Chem. 1961 May;236:1372–1379. [PubMed] [Google Scholar]
- Nojima H., Ikai A., Oshima T., Noda H. Reversible thermal unfolding of thermostable phosphoglycerate kinase. Thermostability associated with mean zero enthalpy change. J Mol Biol. 1977 Nov 5;116(3):429–442. doi: 10.1016/0022-2836(77)90078-x. [DOI] [PubMed] [Google Scholar]
- Ohta Y. Thermostable protease from thermophilic bacteria. II. Studies on the stability of the protease. J Biol Chem. 1967 Feb 10;242(3):509–515. [PubMed] [Google Scholar]
- Perutz M. F., Raidt H. Stereochemical basis of heat stability in bacterial ferredoxins and in haemoglobin A2. Nature. 1975 May 15;255(5505):256–259. doi: 10.1038/255256a0. [DOI] [PubMed] [Google Scholar]
- Tajima M., Urabe I., Yutani K., Okada H. Role of calcium ions in the thermostability of thermolysin and Bacillus subtilis var. amylosacchariticus neutral protease. Eur J Biochem. 1976 Apr 15;64(1):243–247. doi: 10.1111/j.1432-1033.1976.tb10293.x. [DOI] [PubMed] [Google Scholar]
- Von Hippel P. H., Wong K. Y. On the conformational stability of globular proteins. The effects of various electrolytes and nonelectrolytes on the thermal ribonuclease transition. J Biol Chem. 1965 Oct;240(10):3909–3923. [PubMed] [Google Scholar]
- Walker J. E., Wonacott A. J., Harris J. I. Heat stability of a tetrameric enzyme, D-glyceraldehyde-3-phosphate dehydrogenase. Eur J Biochem. 1980 Jul;108(2):581–586. doi: 10.1111/j.1432-1033.1980.tb04753.x. [DOI] [PubMed] [Google Scholar]
- Yutani K., Ogasahara K., Sugino Y., Matsushiro A. Effect of a single amino acid substitution on stability of conformation of a protein. Nature. 1977 May 19;267(5608):274–275. doi: 10.1038/267274a0. [DOI] [PubMed] [Google Scholar]