Abstract
Ubiquitin was purified from chicken testis and its content, biosynthesis and formation of conjugates was determined in germinal cells at successive stages of spermatogenesis. Free ubiquitin increased markedly during spermatogenesis, reaching its maximum level in early spermatids. High levels of ubiquitin were still present in late spermatids but were not detectable in mature spermatozoa. Biosynthesis of ubiquitin occurred in vitro in a fraction containing meiotic and pre-meiotic cells, and during spermiogenesis, in early and late spermatids. The cellular content of free ubiquitin increased after ATP depletion, especially in early spermatids. Lysates of chicken testis cells, particularly those obtained from spermatids, were able to form nuclear (24 and 27 kDa) and extranuclear (55-90 kDa) ubiquitin conjugates in vitro. The presence of increasing levels of ubiquitin and ubiquitin conjugates in chicken spermatids may suggest a possible involvement of this protein in the marked changes of protein turnover, chromatin structure and cell-cell interactions that spermatids undergo during spermiogenesis.
Full text
PDF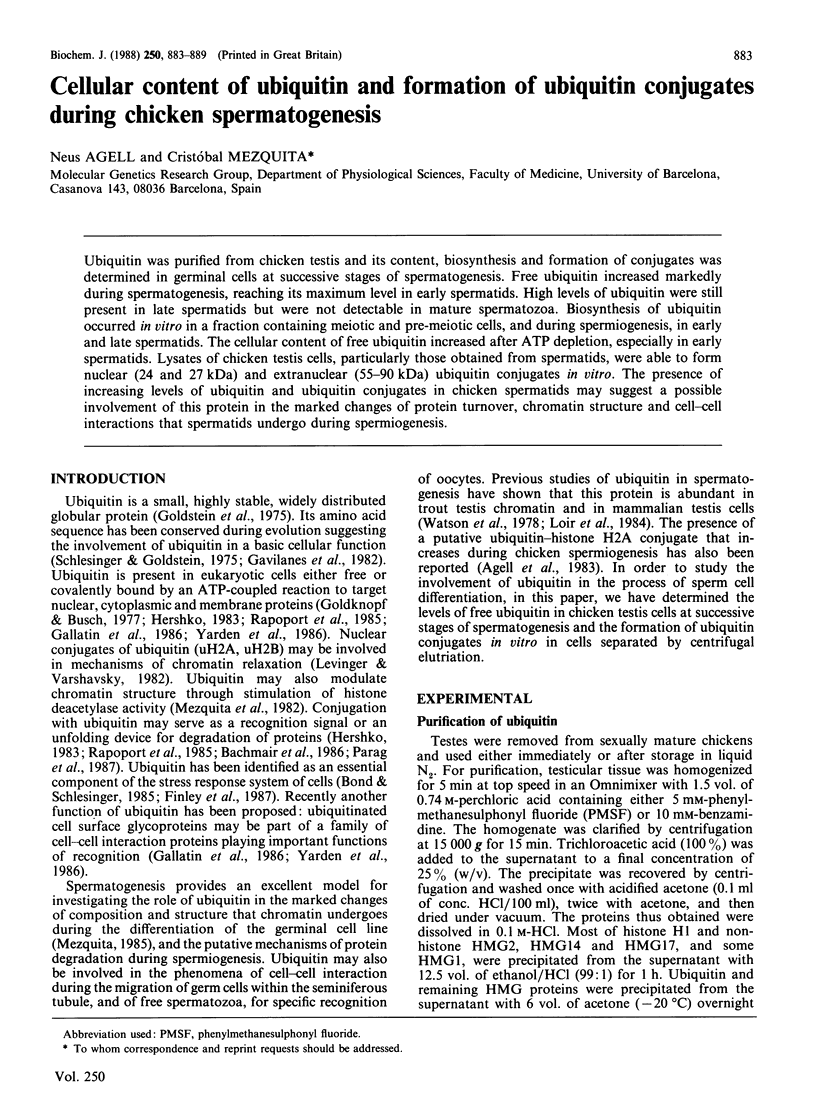
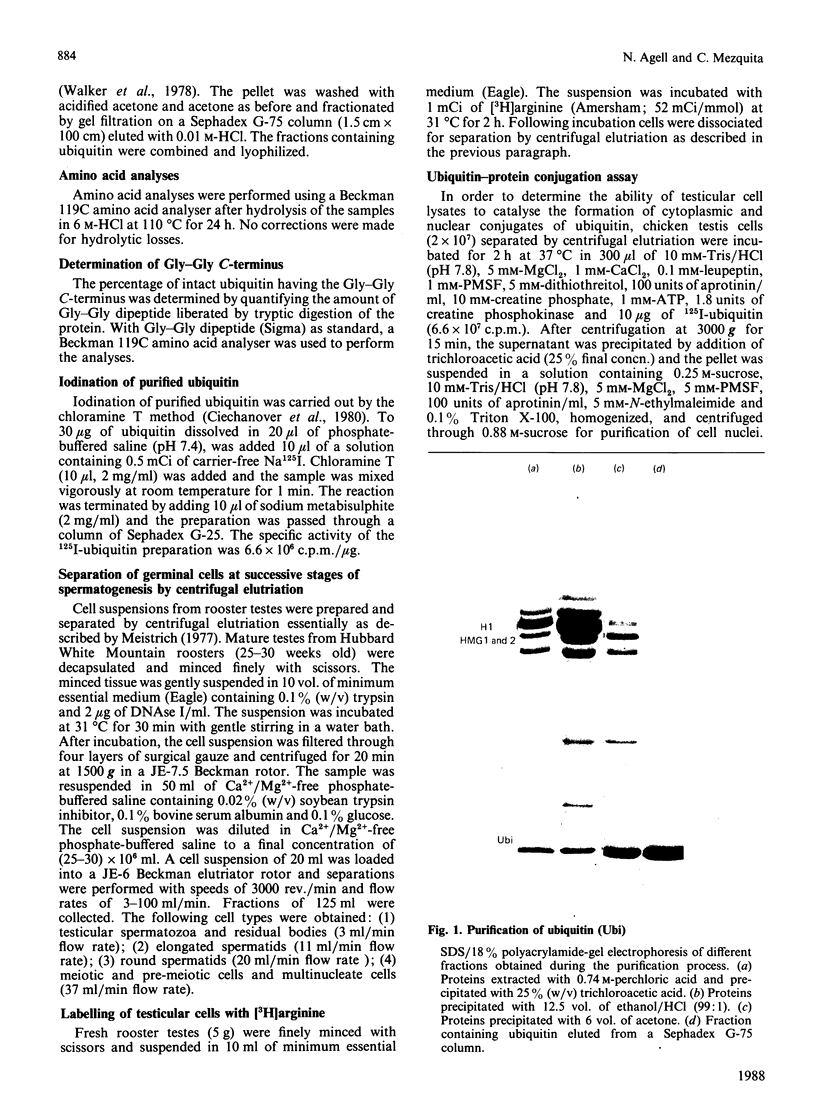
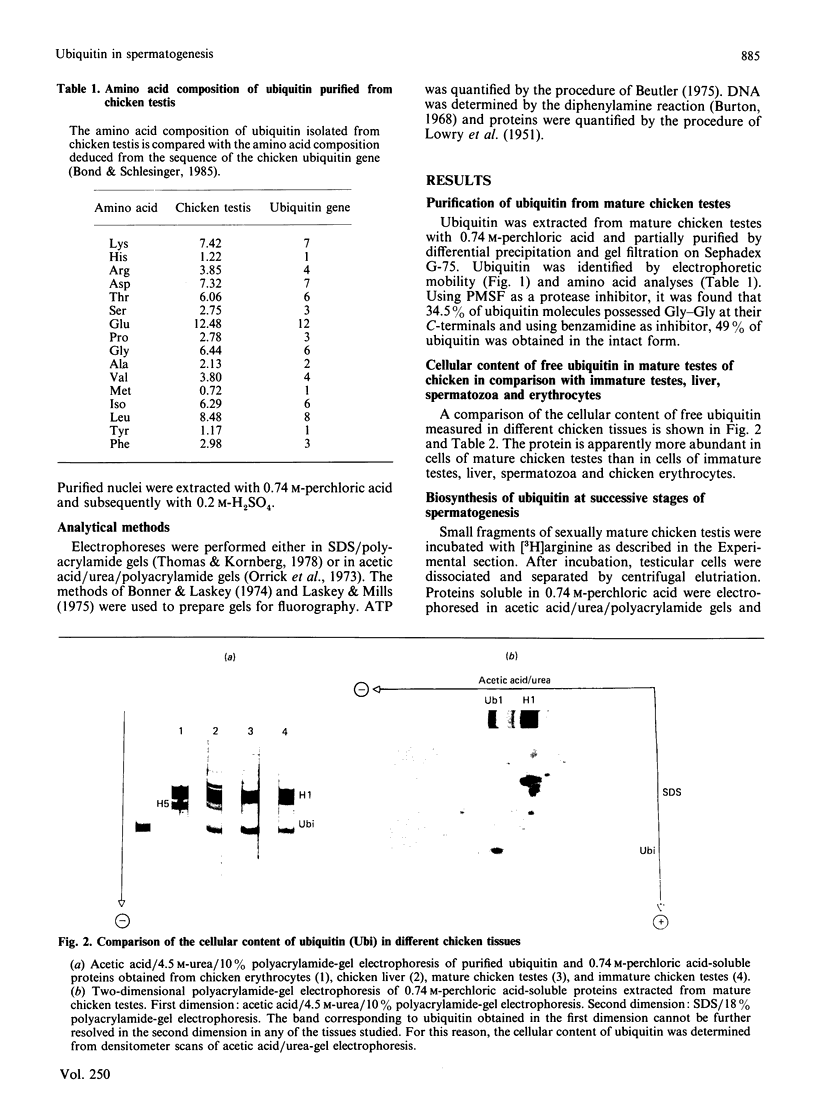
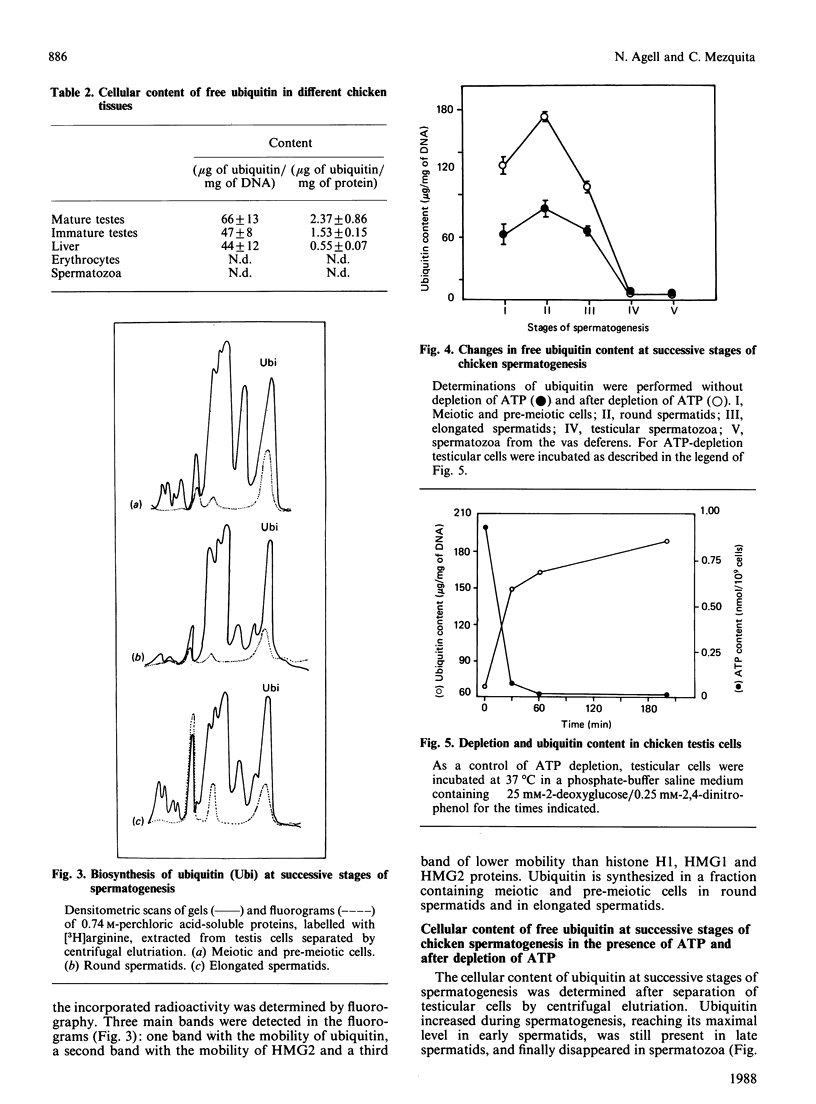
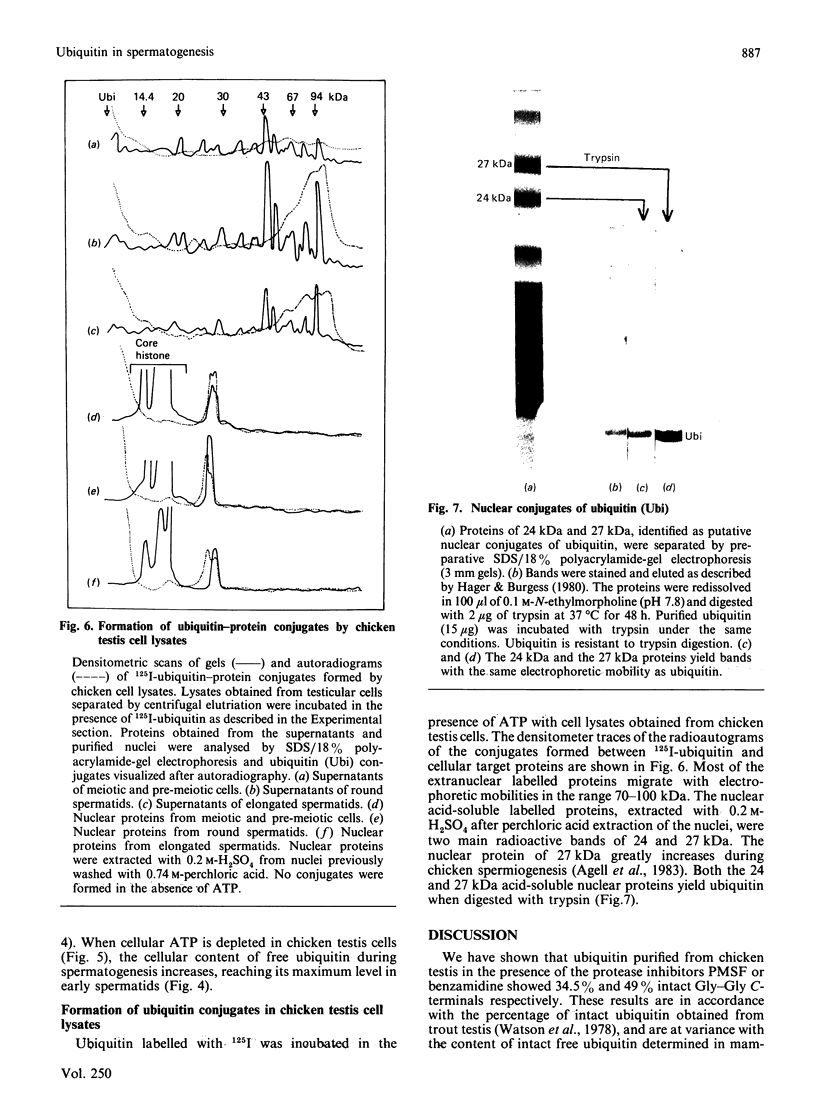
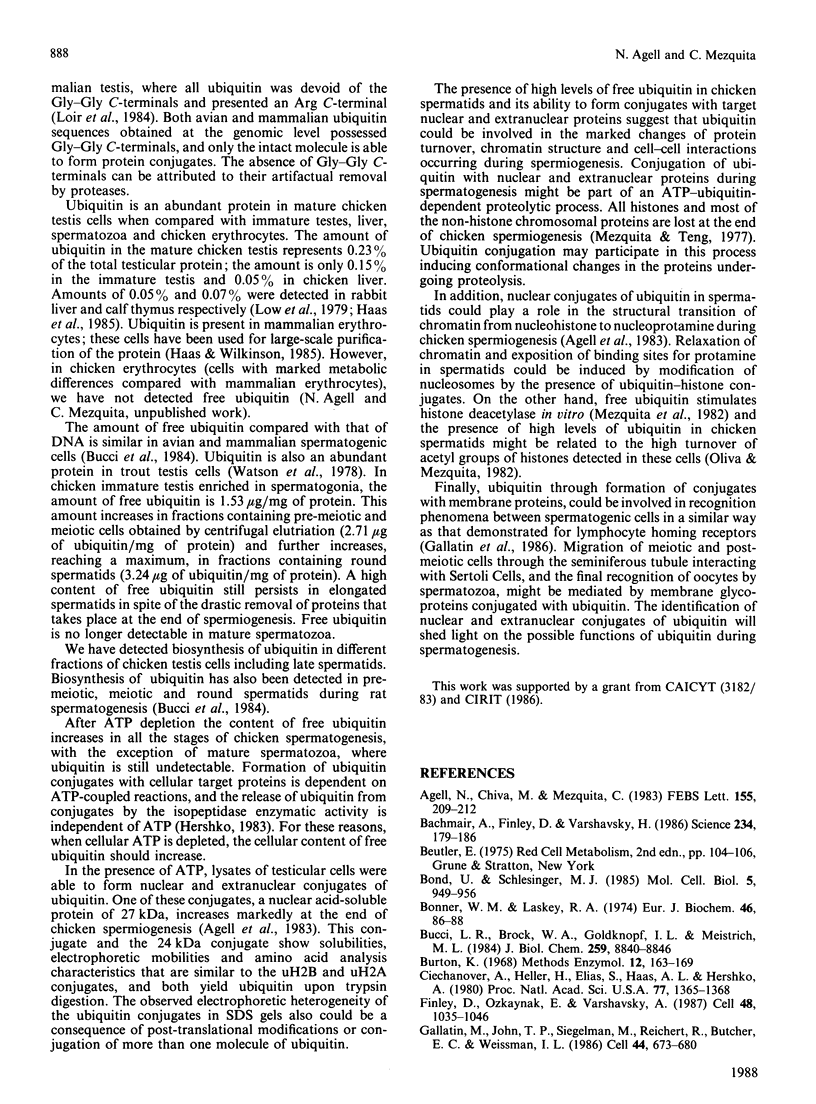
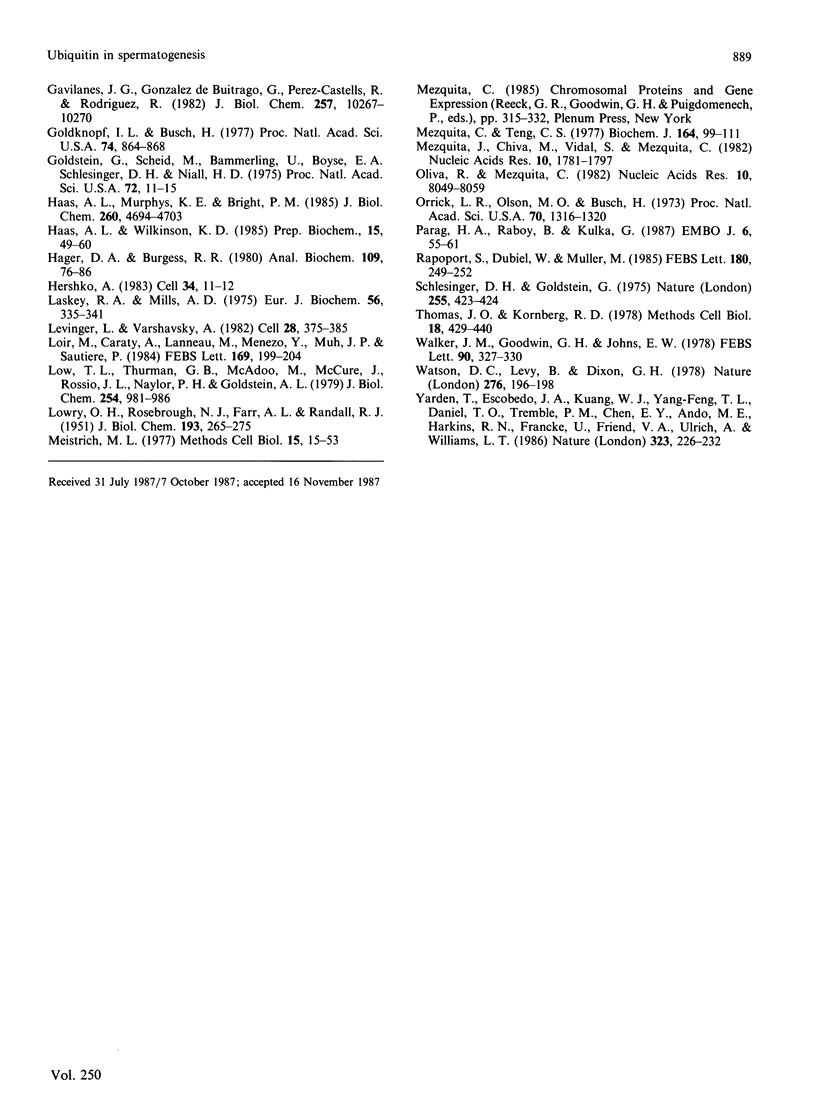
Images in this article
Selected References
These references are in PubMed. This may not be the complete list of references from this article.
- Agell N., Chiva M., Mezquita C. Changes in nuclear content of protein conjugate histone H2A-ubiquitin during rooster spermatogenesis. FEBS Lett. 1983 May 8;155(2):209–212. doi: 10.1016/0014-5793(82)80604-2. [DOI] [PubMed] [Google Scholar]
- Bachmair A., Finley D., Varshavsky A. In vivo half-life of a protein is a function of its amino-terminal residue. Science. 1986 Oct 10;234(4773):179–186. doi: 10.1126/science.3018930. [DOI] [PubMed] [Google Scholar]
- Bond U., Schlesinger M. J. Ubiquitin is a heat shock protein in chicken embryo fibroblasts. Mol Cell Biol. 1985 May;5(5):949–956. doi: 10.1128/mcb.5.5.949. [DOI] [PMC free article] [PubMed] [Google Scholar]
- Bonner W. M., Laskey R. A. A film detection method for tritium-labelled proteins and nucleic acids in polyacrylamide gels. Eur J Biochem. 1974 Jul 1;46(1):83–88. doi: 10.1111/j.1432-1033.1974.tb03599.x. [DOI] [PubMed] [Google Scholar]
- Bucci L. R., Brock W. A., Goldknopf I. L., Meistrich M. L. Characterization of high mobility group protein levels during spermatogenesis in the rat. J Biol Chem. 1984 Jul 25;259(14):8840–8846. [PubMed] [Google Scholar]
- Ciechanover A., Heller H., Elias S., Haas A. L., Hershko A. ATP-dependent conjugation of reticulocyte proteins with the polypeptide required for protein degradation. Proc Natl Acad Sci U S A. 1980 Mar;77(3):1365–1368. doi: 10.1073/pnas.77.3.1365. [DOI] [PMC free article] [PubMed] [Google Scholar]
- Finley D., Ozkaynak E., Varshavsky A. The yeast polyubiquitin gene is essential for resistance to high temperatures, starvation, and other stresses. Cell. 1987 Mar 27;48(6):1035–1046. doi: 10.1016/0092-8674(87)90711-2. [DOI] [PubMed] [Google Scholar]
- Gallatin M., St John T. P., Siegelman M., Reichert R., Butcher E. C., Weissman I. L. Lymphocyte homing receptors. Cell. 1986 Mar 14;44(5):673–680. doi: 10.1016/0092-8674(86)90832-9. [DOI] [PubMed] [Google Scholar]
- Gavilanes J. G., Gonzalez de Buitrago G., Perez-Castells R., Rodriguez R. Isolation, characterization, and amino acid sequence of a ubiquitin-like protein from insect eggs. J Biol Chem. 1982 Sep 10;257(17):10267–10270. [PubMed] [Google Scholar]
- Goldknopf I. L., Busch H. Isopeptide linkage between nonhistone and histone 2A polypeptides of chromosomal conjugate-protein A24. Proc Natl Acad Sci U S A. 1977 Mar;74(3):864–868. doi: 10.1073/pnas.74.3.864. [DOI] [PMC free article] [PubMed] [Google Scholar]
- Goldstein G., Scheid M., Hammerling U., Schlesinger D. H., Niall H. D., Boyse E. A. Isolation of a polypeptide that has lymphocyte-differentiating properties and is probably represented universally in living cells. Proc Natl Acad Sci U S A. 1975 Jan;72(1):11–15. doi: 10.1073/pnas.72.1.11. [DOI] [PMC free article] [PubMed] [Google Scholar]
- Haas A. L., Murphy K. E., Bright P. M. The inactivation of ubiquitin accounts for the inability to demonstrate ATP, ubiquitin-dependent proteolysis in liver extracts. J Biol Chem. 1985 Apr 25;260(8):4694–4703. [PubMed] [Google Scholar]
- Haas A. L., Wilkinson K. D. The large scale purification of ubiquitin from human erythrocytes. Prep Biochem. 1985;15(1-2):49–60. doi: 10.1080/00327488508062433. [DOI] [PubMed] [Google Scholar]
- Hager D. A., Burgess R. R. Elution of proteins from sodium dodecyl sulfate-polyacrylamide gels, removal of sodium dodecyl sulfate, and renaturation of enzymatic activity: results with sigma subunit of Escherichia coli RNA polymerase, wheat germ DNA topoisomerase, and other enzymes. Anal Biochem. 1980 Nov 15;109(1):76–86. doi: 10.1016/0003-2697(80)90013-5. [DOI] [PubMed] [Google Scholar]
- Hershko A. Ubiquitin: roles in protein modification and breakdown. Cell. 1983 Aug;34(1):11–12. doi: 10.1016/0092-8674(83)90131-9. [DOI] [PubMed] [Google Scholar]
- LOWRY O. H., ROSEBROUGH N. J., FARR A. L., RANDALL R. J. Protein measurement with the Folin phenol reagent. J Biol Chem. 1951 Nov;193(1):265–275. [PubMed] [Google Scholar]
- Laskey R. A., Mills A. D. Quantitative film detection of 3H and 14C in polyacrylamide gels by fluorography. Eur J Biochem. 1975 Aug 15;56(2):335–341. doi: 10.1111/j.1432-1033.1975.tb02238.x. [DOI] [PubMed] [Google Scholar]
- Lehman W. Hybrid troponin reconstituted from vertebrate and arthropod subunits. Nature. 1975 May 29;255(5507):424–426. doi: 10.1038/255424a0. [DOI] [PubMed] [Google Scholar]
- Levinger L., Varshavsky A. Selective arrangement of ubiquitinated and D1 protein-containing nucleosomes within the Drosophila genome. Cell. 1982 Feb;28(2):375–385. doi: 10.1016/0092-8674(82)90355-5. [DOI] [PubMed] [Google Scholar]
- Loir M., Caraty A., Lanneau M., Menezo Y., Muh J. P., Sautiere P. Purification and characterization of ubiquitin from mammalian testis. FEBS Lett. 1984 Apr 24;169(2):199–204. doi: 10.1016/0014-5793(84)80318-x. [DOI] [PubMed] [Google Scholar]
- Low T. L., Thurman G. B., McAdoo M., McClure J., Rossio J. L., Naylor P. H., Goldstein A. L. The chemistry and biology of thymosin. I. Isolation, characterization, and biological activities of thymosin alpha1 and polypeptide beta1 from calf thymus. J Biol Chem. 1979 Feb 10;254(3):981–986. [PubMed] [Google Scholar]
- Meistrich M. L. Separation of spermatogenic cells and nuclei from rodent testes. Methods Cell Biol. 1977;15:15–54. doi: 10.1016/s0091-679x(08)60207-1. [DOI] [PubMed] [Google Scholar]
- Mezquita C., Teng C. S. Studies on sex-organ development. Changes in nuclear and chromatin composition and genomic activity during spermatogenesis in the maturing rooster testis. Biochem J. 1977 Apr 15;164(1):99–111. doi: 10.1042/bj1640099. [DOI] [PMC free article] [PubMed] [Google Scholar]
- Mezquita J., Chiva M., Vidal S., Mezquita C. Effect of high mobility group nonhistone proteins HMG-20 (ubiquitin) and HMG-17 on histone deacetylase activity assayed in vitro. Nucleic Acids Res. 1982 Mar 11;10(5):1781–1797. doi: 10.1093/nar/10.5.1781. [DOI] [PMC free article] [PubMed] [Google Scholar]
- Oliva R., Mezquita C. Histone H4 hyperacetylation and rapid turnover of its acetyl groups in transcriptionally inactive rooster testis spermatids. Nucleic Acids Res. 1982 Dec 20;10(24):8049–8059. doi: 10.1093/nar/10.24.8049. [DOI] [PMC free article] [PubMed] [Google Scholar]
- Orrick L. R., Olson M. O., Busch H. Comparison of nucleolar proteins of normal rat liver and Novikoff hepatoma ascites cells by two-dimensional polyacrylamide gel electrophoresis. Proc Natl Acad Sci U S A. 1973 May;70(5):1316–1320. doi: 10.1073/pnas.70.5.1316. [DOI] [PMC free article] [PubMed] [Google Scholar]
- Parag H. A., Raboy B., Kulka R. G. Effect of heat shock on protein degradation in mammalian cells: involvement of the ubiquitin system. EMBO J. 1987 Jan;6(1):55–61. doi: 10.1002/j.1460-2075.1987.tb04718.x. [DOI] [PMC free article] [PubMed] [Google Scholar]
- Rapoport S., Dubiel W., Müller M. Proteolysis of mitochondria in reticulocytes during maturation is ubiquitin-dependent and is accompanied by a high rate of ATP hydrolysis. FEBS Lett. 1985 Jan 28;180(2):249–252. doi: 10.1016/0014-5793(85)81080-2. [DOI] [PubMed] [Google Scholar]
- Thomas J. O., Kornberg R. D. The study of histone--histone associations by chemical cross-linking. Methods Cell Biol. 1978;18:429–440. [PubMed] [Google Scholar]
- Walker J. M., Goodwin G. H., Johns E. W. The isolation and identification of ubiquitin from the high mobility group (HMG) non-histone protein fraction. FEBS Lett. 1978 Jun 15;90(2):327–330. doi: 10.1016/0014-5793(78)80397-4. [DOI] [PubMed] [Google Scholar]
- Watson D. C., Levy W. B., Dixon G. H. Free ubiquitin is a non-histone protein of trout testis chromatin. Nature. 1978 Nov 9;276(5684):196–198. doi: 10.1038/276196a0. [DOI] [PubMed] [Google Scholar]
- Yarden Y., Escobedo J. A., Kuang W. J., Yang-Feng T. L., Daniel T. O., Tremble P. M., Chen E. Y., Ando M. E., Harkins R. N., Francke U. Structure of the receptor for platelet-derived growth factor helps define a family of closely related growth factor receptors. Nature. 1986 Sep 18;323(6085):226–232. doi: 10.1038/323226a0. [DOI] [PubMed] [Google Scholar]