Abstract
The permeability of simian erythrocytes to choline was found to be considerably increased after infection by the malaria parasite, Plasmodium knowlesi. Choline entry occurs by a facilitated-diffusion system involving a carrier, which displays temperature-dependence, saturability with choline (Km = 8.5 +/- 0.7 microM) and specificity. This carrier can also be inhibited by a thiol reagent, N-ethylmaleimide, at an inactivation rate which is, in the absence of choline, the same as in normal erythrocytes. Inactivation by N-ethylmaleimide can be accelerated by external choline and prevented by decamethonium, which acts as an inhibitor of choline entry in infected cells (as with dodecyltrimethylammonium). Both ethanolamine and imidazole act as inhibitors or activators of choline entry in infected erythrocytes, depending on the relative concentrations of choline and of the competing compound (i.e. ethanolamine or imidazole). After infection, the maximum velocity reached 2.84 +/- 0.5 nmol/min per 10(10) infected cells, which is more than 10 times the Vmax. of normal erythrocytes. Impairing the biosynthesis of phosphatidylcholine de novo in Plasmodium-infected erythrocytes by various methods (glucose or ATP depletion, high ethanolamine concentrations) did not result in any alteration of choline transport (Km or Vmax.), indicating that the constant triggering and transformation of choline into phosphatidylcholine by the parasite is not directly responsible for the increase in the choline transport rate after infection. This high increase in choline transport activity is more likely related to modifications in choline carriers and/or in their environment after Plasmodium infection.
Full text
PDF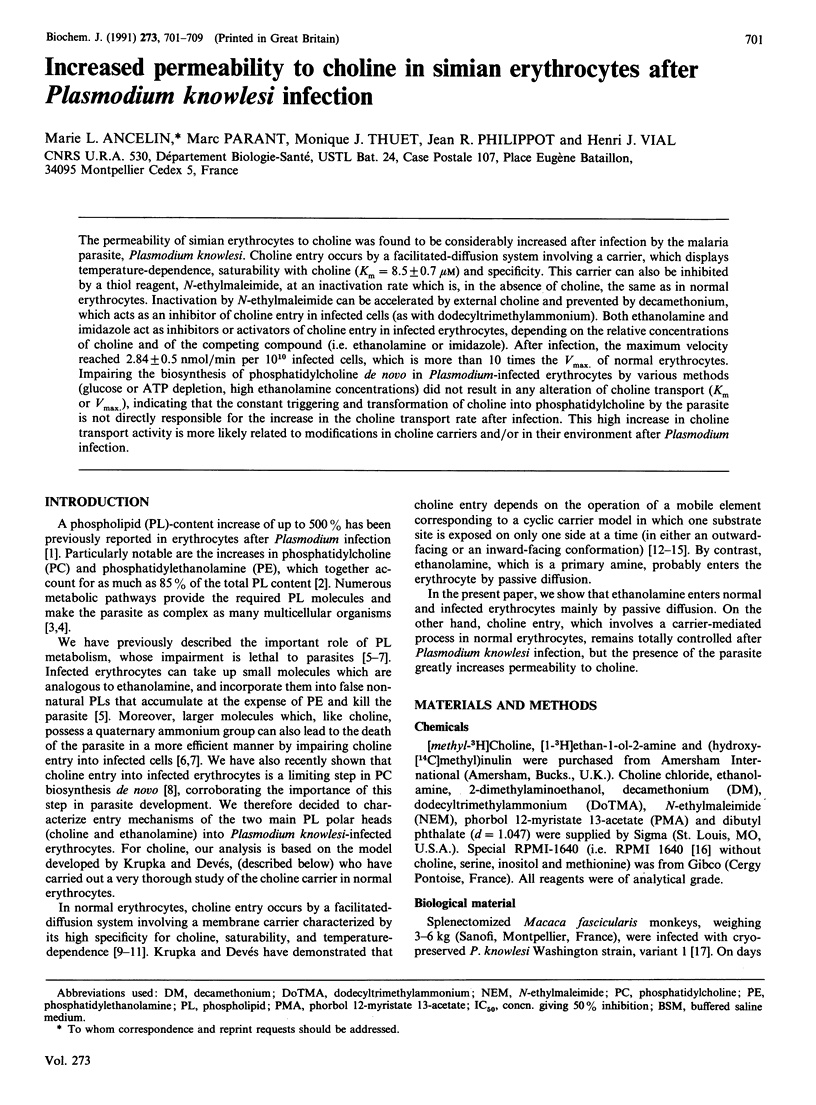
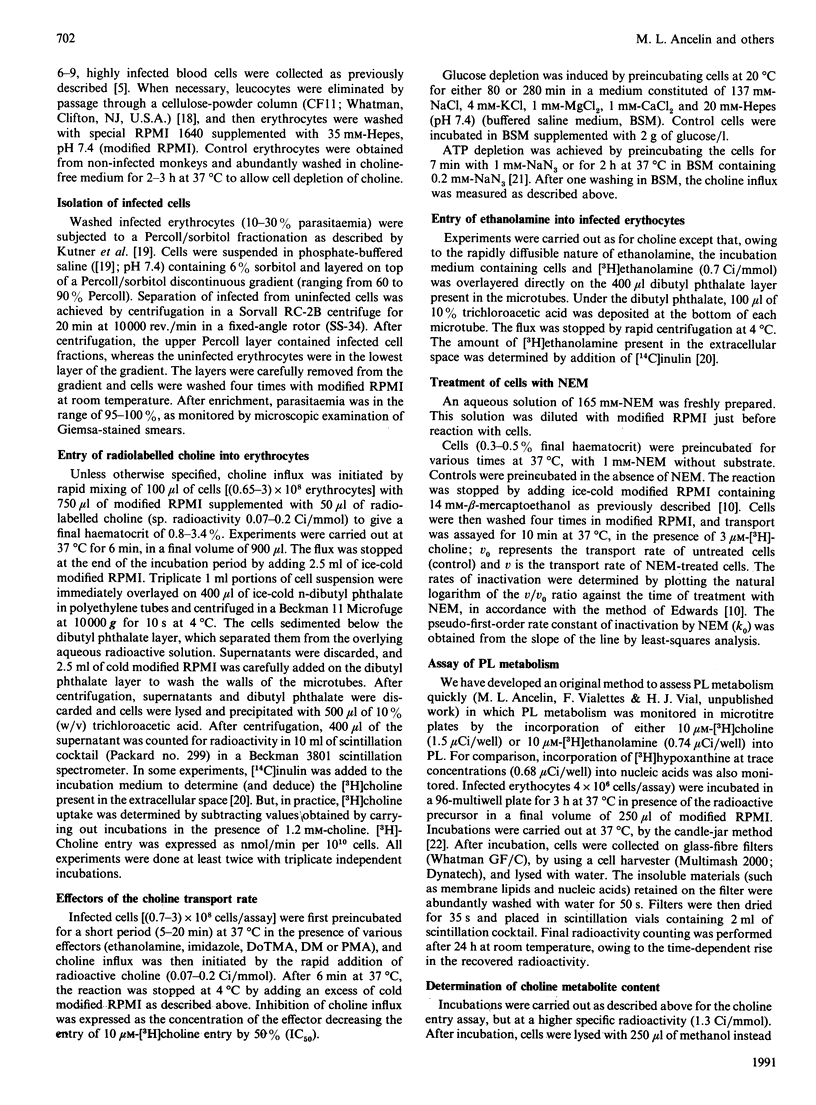
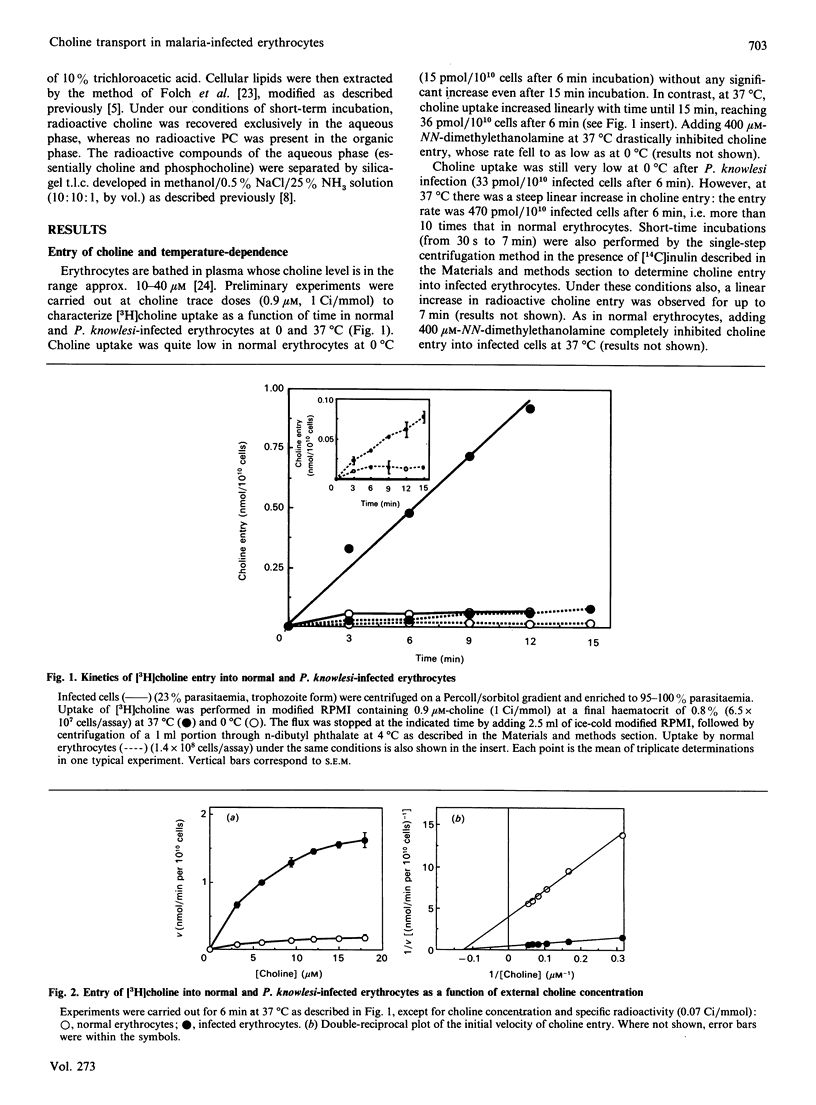
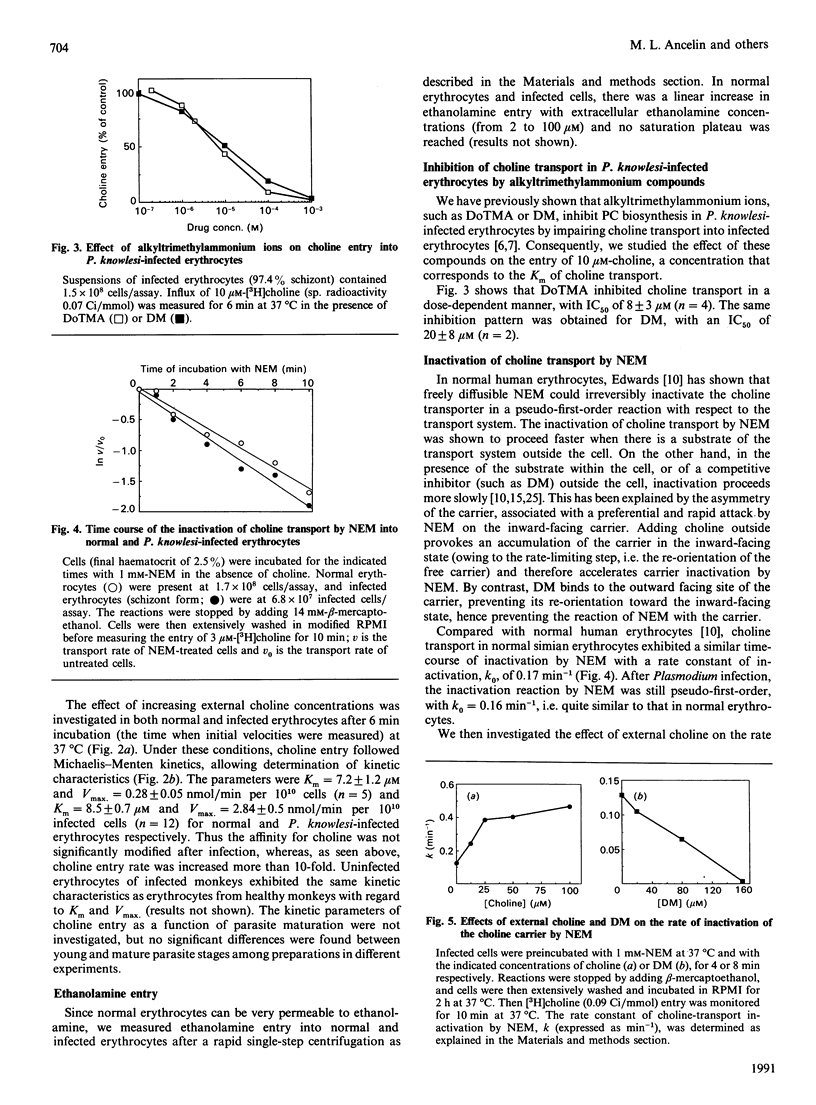
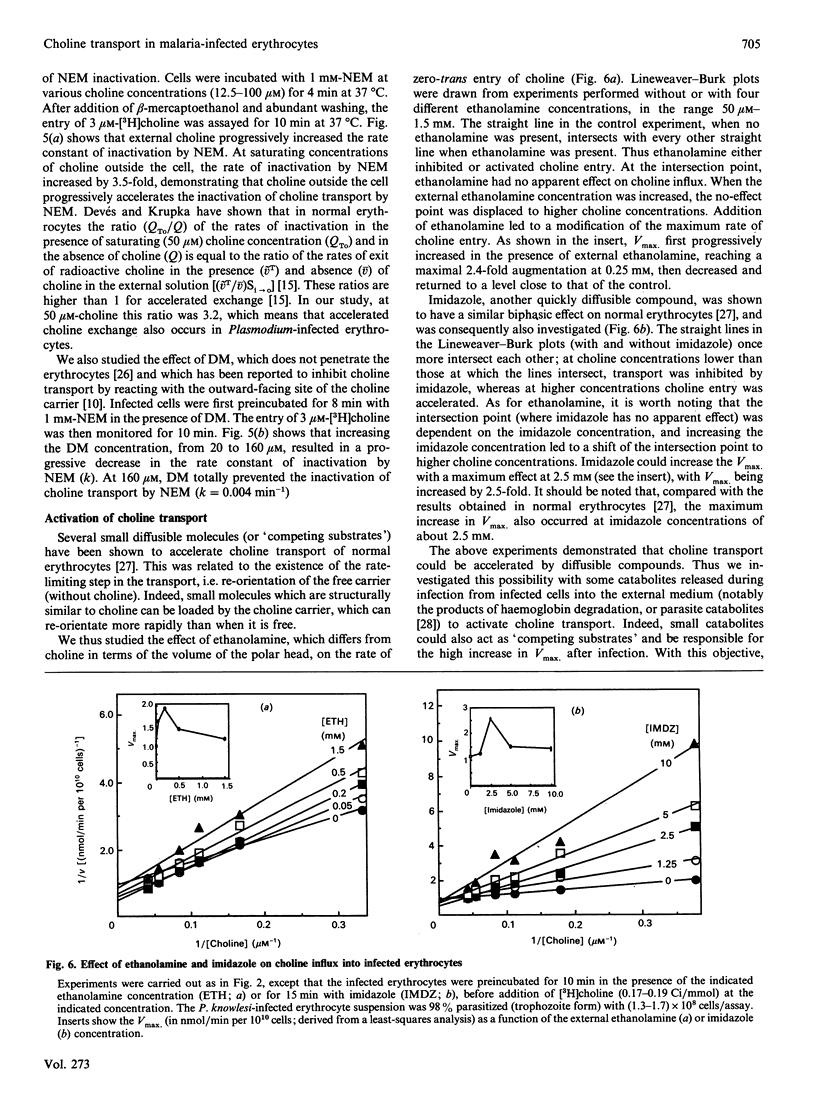
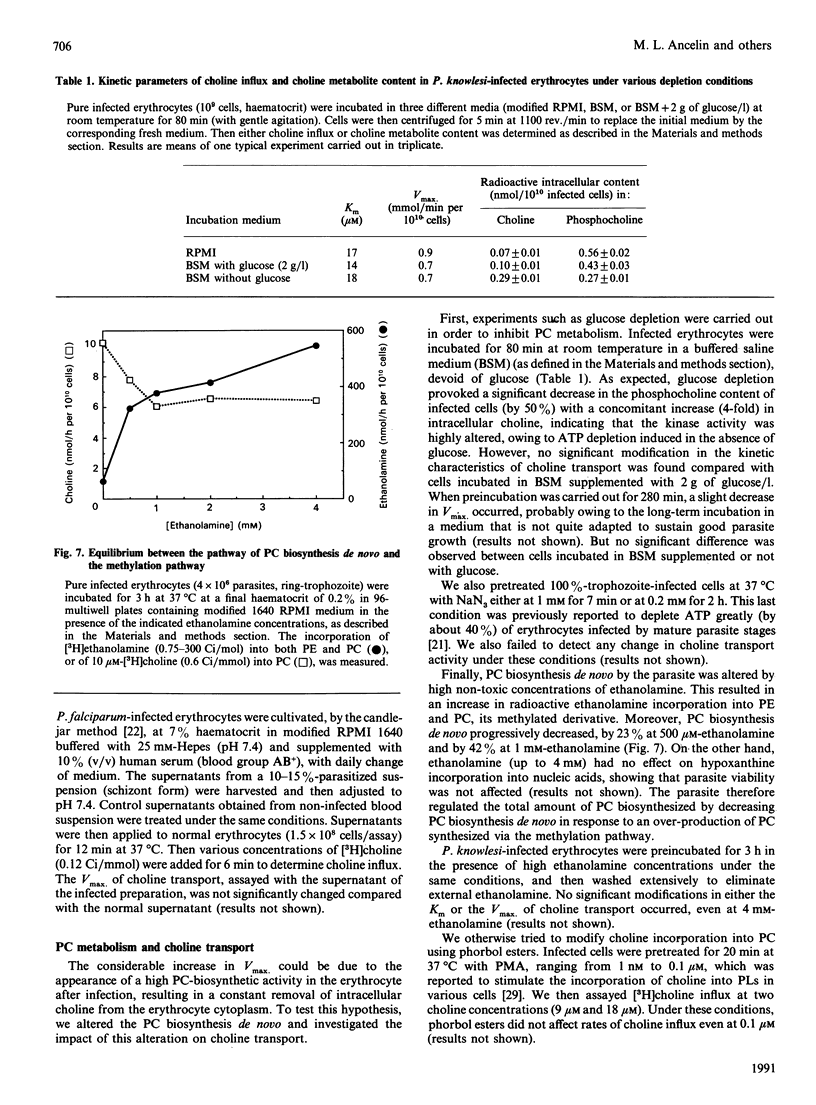
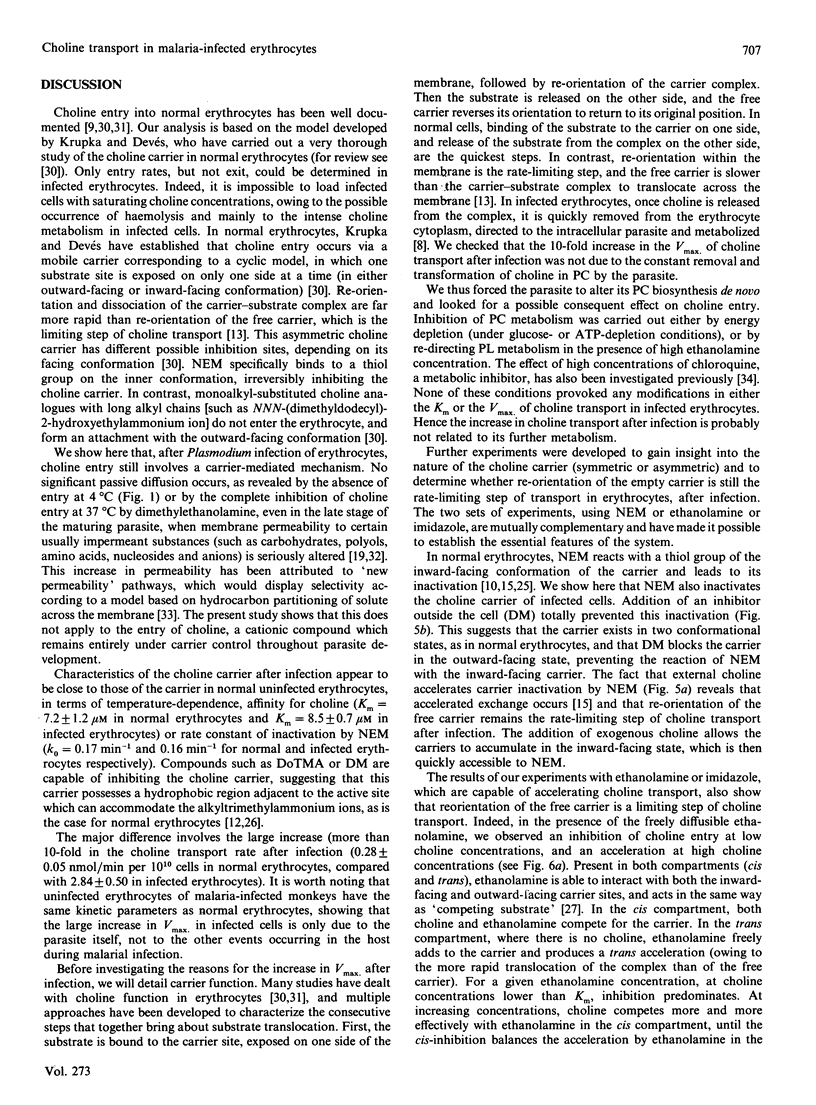
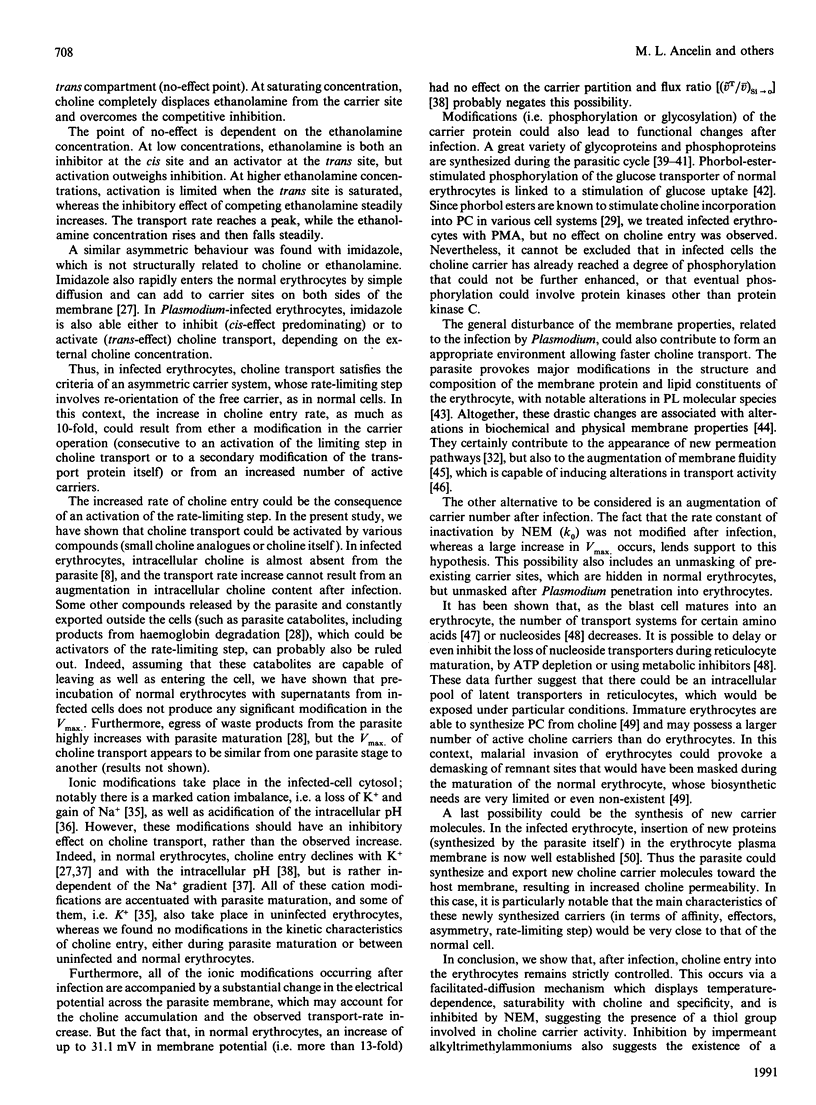
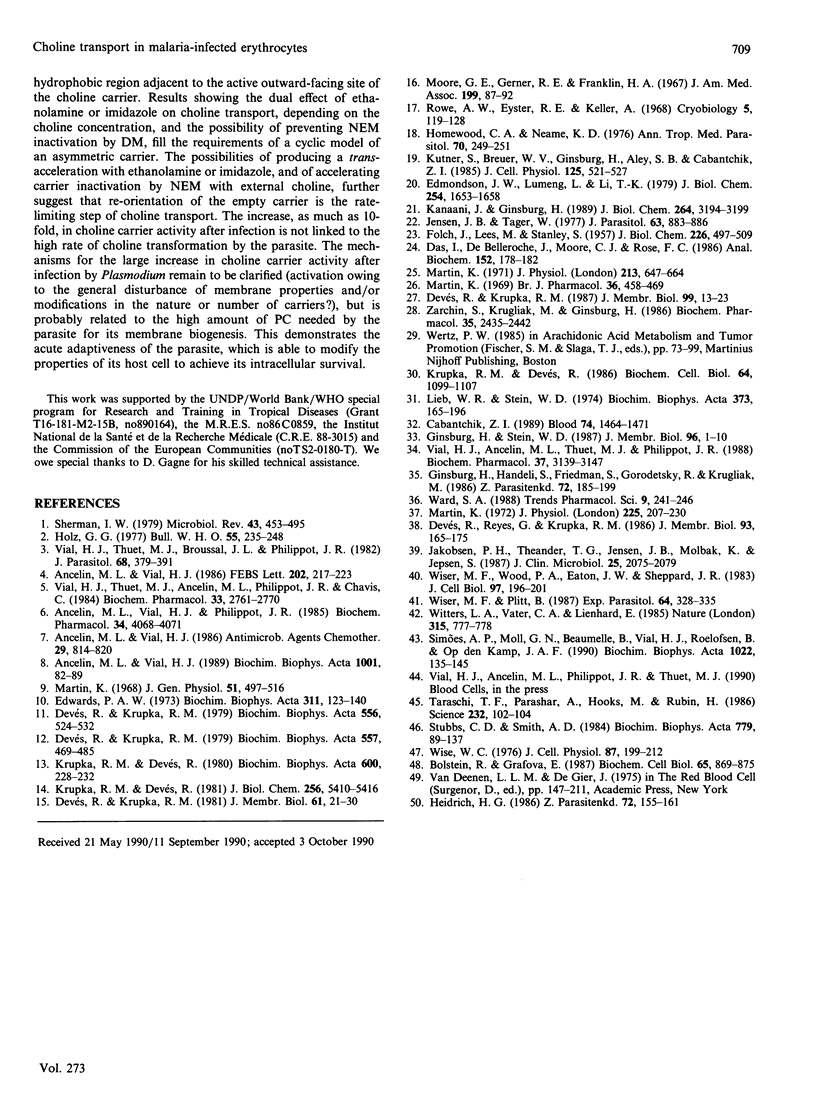
Selected References
These references are in PubMed. This may not be the complete list of references from this article.
- Ancelin M. L., Vial H. J., Philippot J. R. Inhibitors of choline transport into Plasmodium-infected erythrocytes are effective antiplasmodial compounds in vitro. Biochem Pharmacol. 1985 Nov 15;34(22):4068–4071. doi: 10.1016/0006-2952(85)90390-9. [DOI] [PubMed] [Google Scholar]
- Ancelin M. L., Vial H. J. Quaternary ammonium compounds efficiently inhibit Plasmodium falciparum growth in vitro by impairment of choline transport. Antimicrob Agents Chemother. 1986 May;29(5):814–820. doi: 10.1128/aac.29.5.814. [DOI] [PMC free article] [PubMed] [Google Scholar]
- Ancelin M. L., Vial H. J. Regulation of phosphatidylcholine biosynthesis in Plasmodium-infected erythrocytes. Biochim Biophys Acta. 1989 Jan 23;1001(1):82–89. doi: 10.1016/0005-2760(89)90310-x. [DOI] [PubMed] [Google Scholar]
- Ancelin M. L., Vial H. J. Several lines of evidence demonstrating that Plasmodium falciparum, a parasitic organism, has distinct enzymes for the phosphorylation of choline and ethanolamine. FEBS Lett. 1986 Jul 7;202(2):217–223. doi: 10.1016/0014-5793(86)80690-1. [DOI] [PubMed] [Google Scholar]
- Blostein R., Grafova E. Characteristics of membrane transport losses during reticulocyte maturation. Biochem Cell Biol. 1987 Oct;65(10):869–875. doi: 10.1139/o87-113. [DOI] [PubMed] [Google Scholar]
- Cabantchik Z. L. Altered membrane transport of malaria-infected erythrocytes: a possible pharmacologic target. Blood. 1989 Oct;74(5):1464–1471. [PubMed] [Google Scholar]
- Das I., de Belleroche J., Moore C. J., Rose F. C. Determination of free choline in plasma and erythrocyte samples and choline derived from membrane phosphatidylcholine by a chemiluminescence method. Anal Biochem. 1986 Jan;152(1):178–182. doi: 10.1016/0003-2697(86)90138-7. [DOI] [PubMed] [Google Scholar]
- Devés R., Krupka R. M. A simple experimental approach to the determination of carrier transport parameters for unlabeled substrate analogs. Biochim Biophys Acta. 1979 Oct 5;556(3):524–532. doi: 10.1016/0005-2736(79)90138-x. [DOI] [PubMed] [Google Scholar]
- Devés R., Krupka R. M. Effects on transport of rapidly penetrating, competing substrates: activation and inhibition of the choline carrier in erythrocytes by imidazole. J Membr Biol. 1987;99(1):13–23. doi: 10.1007/BF01870618. [DOI] [PubMed] [Google Scholar]
- Devés R., Krupka R. M. Evidence for a two-state mobile carrier mechanism in erythrocyte choline transport: effects of substrate analogs on inactivation of the carrier by N-ethylmaleimide. J Membr Biol. 1981;61(1):21–30. doi: 10.1007/BF01870749. [DOI] [PubMed] [Google Scholar]
- Devés R., Krupka R. M. The binding and translocation steps in transport as related to substrate structure. A study of the choline carrier of erythrocytes. Biochim Biophys Acta. 1979 Nov 2;557(2):469–485. doi: 10.1016/0005-2736(79)90344-4. [DOI] [PubMed] [Google Scholar]
- Devés R., Reyes G., Krupka R. M. The carrier reorientation step in erythrocyte choline transport: pH effects and the involvement of a carrier ionizing group. J Membr Biol. 1986;93(2):165–175. doi: 10.1007/BF01870808. [DOI] [PubMed] [Google Scholar]
- Edmondson J. W., Lumeng L., Li T. K. Comparative studies of alanine and alpha-aminoisobutyric acid uptake by freshly isolated rat liver cells. J Biol Chem. 1979 Mar 10;254(5):1653–1658. [PubMed] [Google Scholar]
- Edwards P. A. Evidence for the carrier model of transport from the inhibition by N-ethylmaleimide of choline transport across the human red cell membrane. Biochim Biophys Acta. 1973 Jun 7;311(1):123–140. doi: 10.1016/0005-2736(73)90260-5. [DOI] [PubMed] [Google Scholar]
- FOLCH J., LEES M., SLOANE STANLEY G. H. A simple method for the isolation and purification of total lipides from animal tissues. J Biol Chem. 1957 May;226(1):497–509. [PubMed] [Google Scholar]
- Ginsburg H., Handeli S., Friedman S., Gorodetsky R., Krugliak M. Effects of red blood cell potassium and hypertonicity on the growth of Plasmodium falciparum in culture. Z Parasitenkd. 1986;72(2):185–199. doi: 10.1007/BF00931146. [DOI] [PubMed] [Google Scholar]
- Ginsburg H., Stein W. D. Biophysical analysis of novel transport pathways induced in red blood cell membranes. J Membr Biol. 1987;96(1):1–10. doi: 10.1007/BF01869329. [DOI] [PubMed] [Google Scholar]
- Homewood C. A., Neame K. D. A comparison of methods used for the removal of white cells from malaria-infected blood. Ann Trop Med Parasitol. 1976 Jun;70(2):249–251. doi: 10.1080/00034983.1976.11687119. [DOI] [PubMed] [Google Scholar]
- Jakobsen P. H., Theander T. G., Jensen J. B., Mølbak K., Jepsen S. Soluble Plasmodium falciparum antigens contain carbohydrate moieties important for immune reactivity. J Clin Microbiol. 1987 Nov;25(11):2075–2079. doi: 10.1128/jcm.25.11.2075-2079.1987. [DOI] [PMC free article] [PubMed] [Google Scholar]
- Jensen J. B., Trager W. Plasmodium falciparum in culture: use of outdated erthrocytes and description of the candle jar method. J Parasitol. 1977 Oct;63(5):883–886. [PubMed] [Google Scholar]
- Kanaani J., Ginsburg H. Metabolic interconnection between the human malarial parasite Plasmodium falciparum and its host erythrocyte. Regulation of ATP levels by means of an adenylate translocator and adenylate kinase. J Biol Chem. 1989 Feb 25;264(6):3194–3199. [PubMed] [Google Scholar]
- Krupka R. M., Devés R. An experimental test for cyclic versus linear transport models. The mechanisms of glucose and choline transport in erythrocytes. J Biol Chem. 1981 Jun 10;256(11):5410–5416. [PubMed] [Google Scholar]
- Krupka R. M., Devés R. Looking for probes of gated channels: studies of the inhibition of glucose and choline transport in erythrocytes. Biochem Cell Biol. 1986 Nov;64(11):1099–1107. doi: 10.1139/o86-145. [DOI] [PubMed] [Google Scholar]
- Krupka R. M., Devés R. The choline transport system of erythrocytes distribution of the free carrier in the membrane. Biochim Biophys Acta. 1980 Jul 16;600(1):228–232. doi: 10.1016/0005-2736(80)90427-7. [DOI] [PubMed] [Google Scholar]
- Kutner S., Breuer W. V., Ginsburg H., Aley S. B., Cabantchik Z. I. Characterization of permeation pathways in the plasma membrane of human erythrocytes infected with early stages of Plasmodium falciparum: association with parasite development. J Cell Physiol. 1985 Dec;125(3):521–527. doi: 10.1002/jcp.1041250323. [DOI] [PubMed] [Google Scholar]
- Lieb W. R., Stein W. D. Testing and characterizing the simple carrier. Biochim Biophys Acta. 1974 Dec 10;373(2):178–196. doi: 10.1016/0005-2736(74)90144-8. [DOI] [PubMed] [Google Scholar]
- Martin K. Concentrative accumulation of choline by human erythrocytes. J Gen Physiol. 1968 Apr;51(4):497–516. doi: 10.1085/jgp.51.4.497. [DOI] [PMC free article] [PubMed] [Google Scholar]
- Martin K. Effects of quaternary ammonium compounds on choline transport in red cells. Br J Pharmacol. 1969 Jul;36(3):458–469. doi: 10.1111/j.1476-5381.1969.tb08002.x. [DOI] [PMC free article] [PubMed] [Google Scholar]
- Martin K. Extracellular cations and the movement of choline across the erythrocyte membrane. J Physiol. 1972 Jul;224(1):207–230. doi: 10.1113/jphysiol.1972.sp009890. [DOI] [PMC free article] [PubMed] [Google Scholar]
- Martin K. Some properties of an SH group essential for choline transport in human erythrocytes. J Physiol. 1971 Mar;213(3):647–664. doi: 10.1113/jphysiol.1971.sp009406. [DOI] [PMC free article] [PubMed] [Google Scholar]
- Rowe A. W., Eyster E., Kellner A. Liquid nitrogen preservation of red blood cells for transfusion; a low glycerol-rapid freeze procedure. Cryobiology. 1968 Sep-Oct;5(2):119–128. doi: 10.1016/s0011-2240(68)80154-3. [DOI] [PubMed] [Google Scholar]
- Sherman I. W. Biochemistry of Plasmodium (malarial parasites). Microbiol Rev. 1979 Dec;43(4):453–495. doi: 10.1128/mr.43.4.453-495.1979. [DOI] [PMC free article] [PubMed] [Google Scholar]
- Simões A. P., Moll G. N., Beaumelle B., Vial H. J., Roelofsen B., Op den Kamp J. A. Plasmodium knowlesi induces alterations in phosphatidylcholine and phosphatidylethanolamine molecular species composition of parasitized monkey erythrocytes. Biochim Biophys Acta. 1990 Feb 28;1022(2):135–145. doi: 10.1016/0005-2736(90)90107-y. [DOI] [PubMed] [Google Scholar]
- Stubbs C. D., Smith A. D. The modification of mammalian membrane polyunsaturated fatty acid composition in relation to membrane fluidity and function. Biochim Biophys Acta. 1984 Jan 27;779(1):89–137. doi: 10.1016/0304-4157(84)90005-4. [DOI] [PubMed] [Google Scholar]
- Taraschi T. F., Parashar A., Hooks M., Rubin H. Perturbation of red cell membrane structure during intracellular maturation of Plasmodium falciparum. Science. 1986 Apr 4;232(4746):102–104. doi: 10.1126/science.3006251. [DOI] [PubMed] [Google Scholar]
- Vial H. J., Ancelin M. L., Thuet M. J., Philippot J. R. Differential effects of chloroquine on the phospholipid metabolism of Plasmodium-infected erythrocytes. Biochem Pharmacol. 1988 Aug 15;37(16):3139–3147. doi: 10.1016/0006-2952(88)90312-7. [DOI] [PubMed] [Google Scholar]
- Vial H. J., Thuet M. J., Ancelin M. L., Philippot J. R., Chavis C. Phospholipid metabolism as a new target for malaria chemotherapy. Mechanism of action of D-2-amino-1-butanol. Biochem Pharmacol. 1984 Sep 1;33(17):2761–2770. doi: 10.1016/0006-2952(84)90693-2. [DOI] [PubMed] [Google Scholar]
- Vial H. J., Thuet M. J., Broussal J. L., Philippot J. R. Phospholipid biosynthesis by Plasmodium knowlesi-infected erythrocytes: the incorporation of phospohlipid precursors and the identification of previously undetected metabolic pathways. J Parasitol. 1982 Jun;68(3):379–391. [PubMed] [Google Scholar]
- Ward S. A. Mechanisms of chloroquine resistance in malarial chemotherapy. Trends Pharmacol Sci. 1988 Jul;9(7):241–246. doi: 10.1016/0165-6147(88)90153-8. [DOI] [PubMed] [Google Scholar]
- Wise W. C. Maturation of membrane function: transport of amino acid by rat erythroid cells. J Cell Physiol. 1975 Dec;87(2):199–201. doi: 10.1002/jcp.1040870208. [DOI] [PubMed] [Google Scholar]
- Wiser M. F., Plitt B. Plasmodium berghei, P. chabaudi, and P. falciparum: similarities in phosphoproteins and protein kinase activities and their stage specific expression. Exp Parasitol. 1987 Dec;64(3):328–335. doi: 10.1016/0014-4894(87)90043-9. [DOI] [PubMed] [Google Scholar]
- Wiser M. F., Wood P. A., Eaton J. W., Sheppard J. R. Membrane-associated phosphoproteins in Plasmodium berghei-infected murine erythrocytes. J Cell Biol. 1983 Jul;97(1):196–201. doi: 10.1083/jcb.97.1.196. [DOI] [PMC free article] [PubMed] [Google Scholar]
- Witters L. A., Vater C. A., Lienhard G. E. Phosphorylation of the glucose transporter in vitro and in vivo by protein kinase C. 1985 Jun 27-Jul 3Nature. 315(6022):777–778. doi: 10.1038/315777a0. [DOI] [PubMed] [Google Scholar]
- Zarchin S., Krugliak M., Ginsburg H. Digestion of the host erythrocyte by malaria parasites is the primary target for quinoline-containing antimalarials. Biochem Pharmacol. 1986 Jul 15;35(14):2435–2442. doi: 10.1016/0006-2952(86)90473-9. [DOI] [PubMed] [Google Scholar]