Abstract
The specificity of two genetically related cell-envelope serine proteinases (PI-type and PIII-type) of Lactococcus lactis subsp. cremoris towards the alpha s1-casein-(1-23)-fragment, an important intermediate product of primary chymosin-directed proteolysis in cheese, has been established. Both enzymes showed, at pH 6.5 and under relatively low-ionic-strength conditions, a characteristic, mutually different, cleavage pattern that seems, in the first instance, to be determined by the charge N-terminal to the cleaved bond. With Pi, three cleavage sites were found in the N-terminal positively charged part of the peptide and, with PIII, three sites were found in the C-terminal negatively charged part. Comparison of the specific cleavage sites in this peptide and those in beta-casein revealed similarities with respect to the different residues which can occur N-terminally to the cleaved bond. The properties of these substrate residues match with the structural and various interactive features of the respective binding regions of the enzymes predicted on the basis of a close sequence similarity of the lactococcal proteinases with the subtilisin family. A hydrophobic interaction and/or hydrogen-bridge formation seems to govern the binding of the first amino acid residue N-terminal to the scissile bond. The more distantly N-terminally positioned sequence of residues apparently is attracted electrostatically by a negative charge in the binding region of PI and by a positive charge in that of PIII, provided that the opposite charge is is present at the appropriate position in this sequence. Hence a specific electrostatic binding may occur; additionally, hydrophobic interaction and/or hydrogen-bond formation is important.
Full text
PDF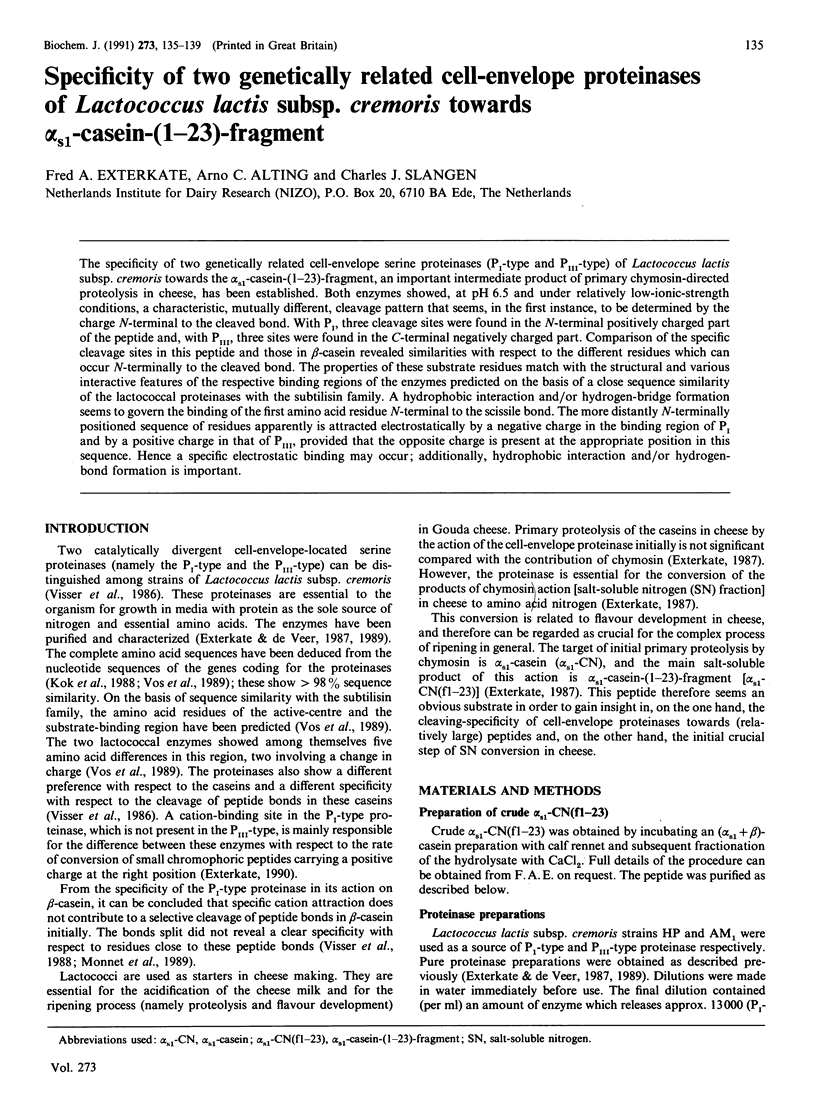
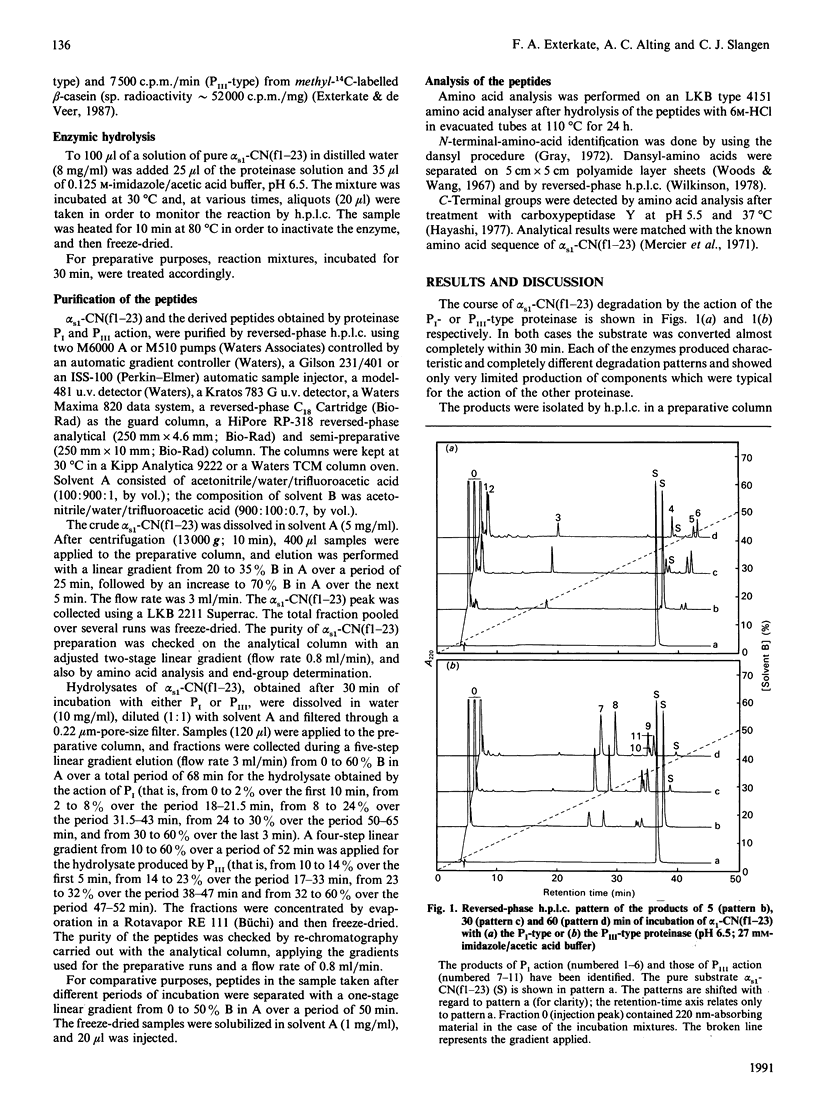
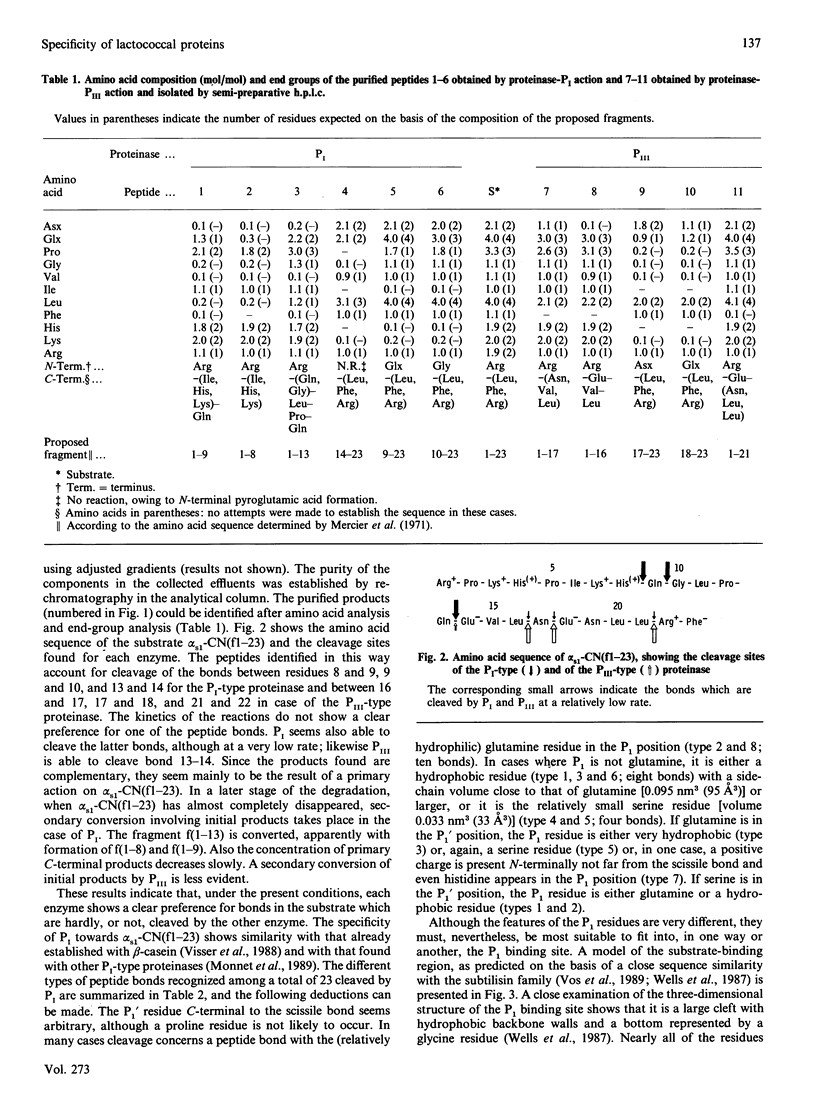
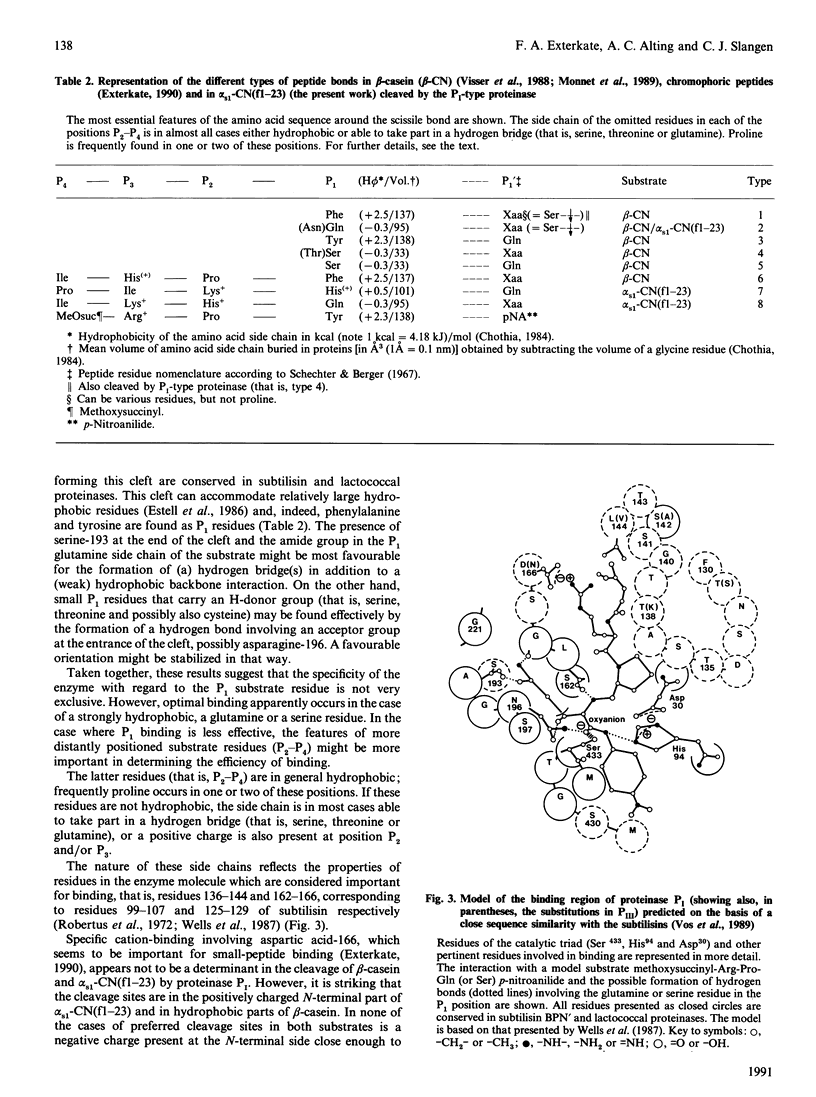
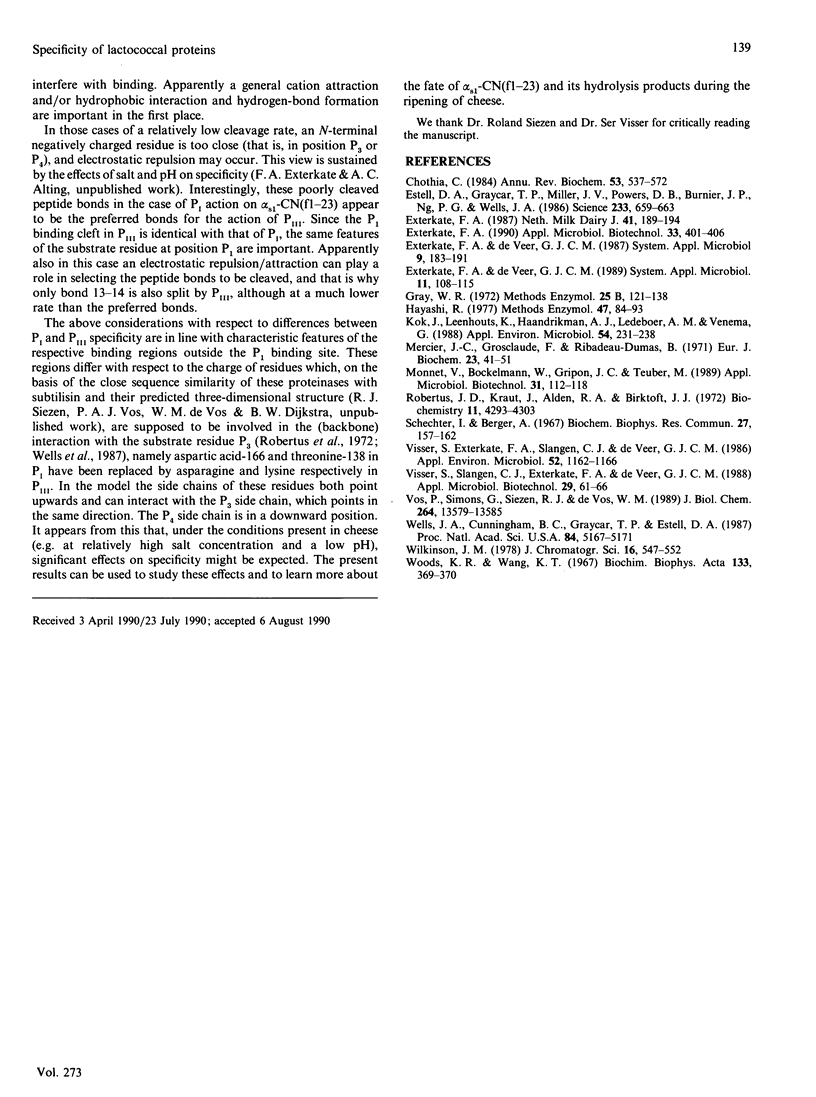
Selected References
These references are in PubMed. This may not be the complete list of references from this article.
- Chothia C. Principles that determine the structure of proteins. Annu Rev Biochem. 1984;53:537–572. doi: 10.1146/annurev.bi.53.070184.002541. [DOI] [PubMed] [Google Scholar]
- Estell D. A., Graycar T. P., Miller J. V., Powers D. B., Wells J. A., Burnier J. P., Ng P. G. Probing steric and hydrophobic effects on enzyme-substrate interactions by protein engineering. Science. 1986 Aug 8;233(4764):659–663. doi: 10.1126/science.233.4764.659. [DOI] [PubMed] [Google Scholar]
- Exterkate F. A. Differences in short peptide-substrate cleavage by two cell-envelope-located serine proteinases of Lactococcus lactis subsp. cremoris are related to secondary binding specificity. Appl Microbiol Biotechnol. 1990 Jul;33(4):401–406. doi: 10.1007/BF00176654. [DOI] [PubMed] [Google Scholar]
- Hayashi R. Carboxypeptidase Y in sequence determination of peptides. Methods Enzymol. 1977;47:84–93. doi: 10.1016/0076-6879(77)47010-1. [DOI] [PubMed] [Google Scholar]
- Kok J., Leenhouts K. J., Haandrikman A. J., Ledeboer A. M., Venema G. Nucleotide sequence of the cell wall proteinase gene of Streptococcus cremoris Wg2. Appl Environ Microbiol. 1988 Jan;54(1):231–238. doi: 10.1128/aem.54.1.231-238.1988. [DOI] [PMC free article] [PubMed] [Google Scholar]
- Mercier J. C., Grosclaude F., Ribadeau-Dumas B. Structure primaire de la caséine s1 -bovine. Séquence complète. Eur J Biochem. 1971 Nov 11;23(1):41–51. doi: 10.1111/j.1432-1033.1971.tb01590.x. [DOI] [PubMed] [Google Scholar]
- Robertus J. D., Kraut J., Alden R. A., Birktoft J. J. Subtilisin; a stereochemical mechanism involving transition-state stabilization. Biochemistry. 1972 Nov 7;11(23):4293–4303. doi: 10.1021/bi00773a016. [DOI] [PubMed] [Google Scholar]
- Schechter I., Berger A. On the size of the active site in proteases. I. Papain. Biochem Biophys Res Commun. 1967 Apr 20;27(2):157–162. doi: 10.1016/s0006-291x(67)80055-x. [DOI] [PubMed] [Google Scholar]
- Visser S., Exterkate F. A., Slangen C. J., de Veer G. J. Comparative Study of Action of Cell Wall Proteinases from Various Strains of Streptococcus cremoris on Bovine alpha(s1)-, beta-, and kappa-Casein. Appl Environ Microbiol. 1986 Nov;52(5):1162–1166. doi: 10.1128/aem.52.5.1162-1166.1986. [DOI] [PMC free article] [PubMed] [Google Scholar]
- Vos P., Simons G., Siezen R. J., de Vos W. M. Primary structure and organization of the gene for a procaryotic, cell envelope-located serine proteinase. J Biol Chem. 1989 Aug 15;264(23):13579–13585. [PubMed] [Google Scholar]
- Wells J. A., Cunningham B. C., Graycar T. P., Estell D. A. Recruitment of substrate-specificity properties from one enzyme into a related one by protein engineering. Proc Natl Acad Sci U S A. 1987 Aug;84(15):5167–5171. doi: 10.1073/pnas.84.15.5167. [DOI] [PMC free article] [PubMed] [Google Scholar]
- Woods K. R., Wang K. T. Separation of dansyl-amino acids by polyamide layer chromatography. Biochim Biophys Acta. 1967 Feb 21;133(2):369–370. doi: 10.1016/0005-2795(67)90078-5. [DOI] [PubMed] [Google Scholar]