Abstract
Glucagon (10 nM) caused a transient elevation of intracellular cyclic AMP concentrations, which reached a peak in around 5 min, and slowly returned to basal values in around 30 min. When 1 mM-3-isobutyl-1-methylxanthine (IBMX) was present, this process yielded a Ka of 1 nM for glucagon. The addition of insulin (10 nM) after 5 min exposure to glucagon (10 nM) caused intracellular cyclic AMP concentrations to fall dramatically, attaining basal values within 10 min. The regulation of this process was dose-dependent, exhibiting a Ka of 0.4 nM for insulin. If insulin and glucagon were added together to hepatocytes, then insulin decreased the magnitude of the cyclic AMP response to glucagon. IBMX (1 mM) prevented insulin antagonizing the action of glucagon in both of these instances. A gentle homogenization procedure followed by a rapid subcellular fractionation of hepatocytes on a Percoll gradient was developed. This was used to resolve subcellular membrane fractions and to identify cyclic AMP phosphodiesterase activity in both membrane and cytosol fractions. Glucagon and insulin only affected the activity of two distinct membrane-bound species, a plasma-membrane enzyme and a 'dense vesicle' enzyme. Glucagon (10 nM), insulin (10 nM), IBMX (1 mM), dibutyryl cyclic AMP (10 microM) and cholera toxin (1 microgram/ml) all elicited the activation of the 'dense vesicle' enzyme. The plasma-membrane enzyme was not activated by glucagon, IBMX or dibutyryl cyclic AMP, although insulin and cholera toxin both led to its activation. The degree of activation of the plasma-membrane enzyme produced by insulin was increased in the presence of IBMX or dibutyryl cyclic AMP. Glucagon pretreatment (5 min) of hepatocytes blocked the ability of insulin to activate the plasma-membrane enzyme. The activity state of these phosphodiesterases is discussed in relation to the observed changes in intracellular cyclic AMP concentrations. It is suggested that insulin exerts its action on the plasma-membrane phosphodiesterase through a mechanism involving a guanine nucleotide-regulatory protein.
Full text
PDF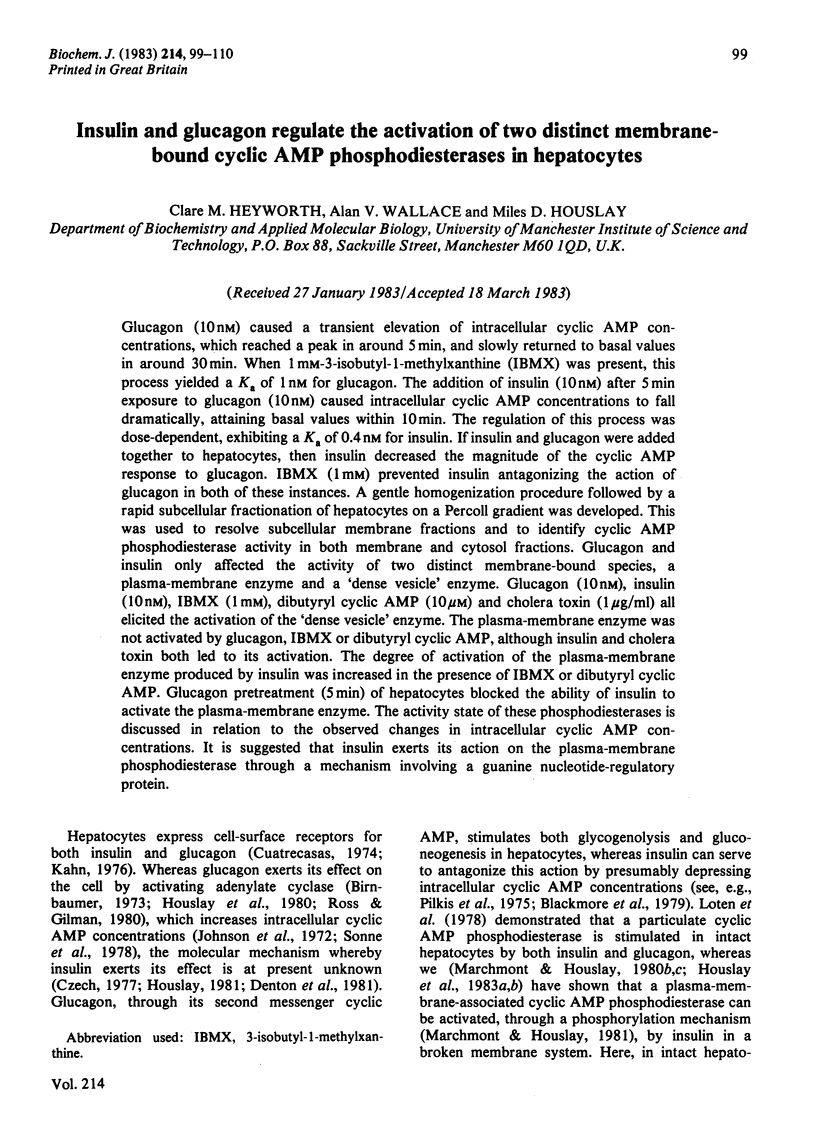
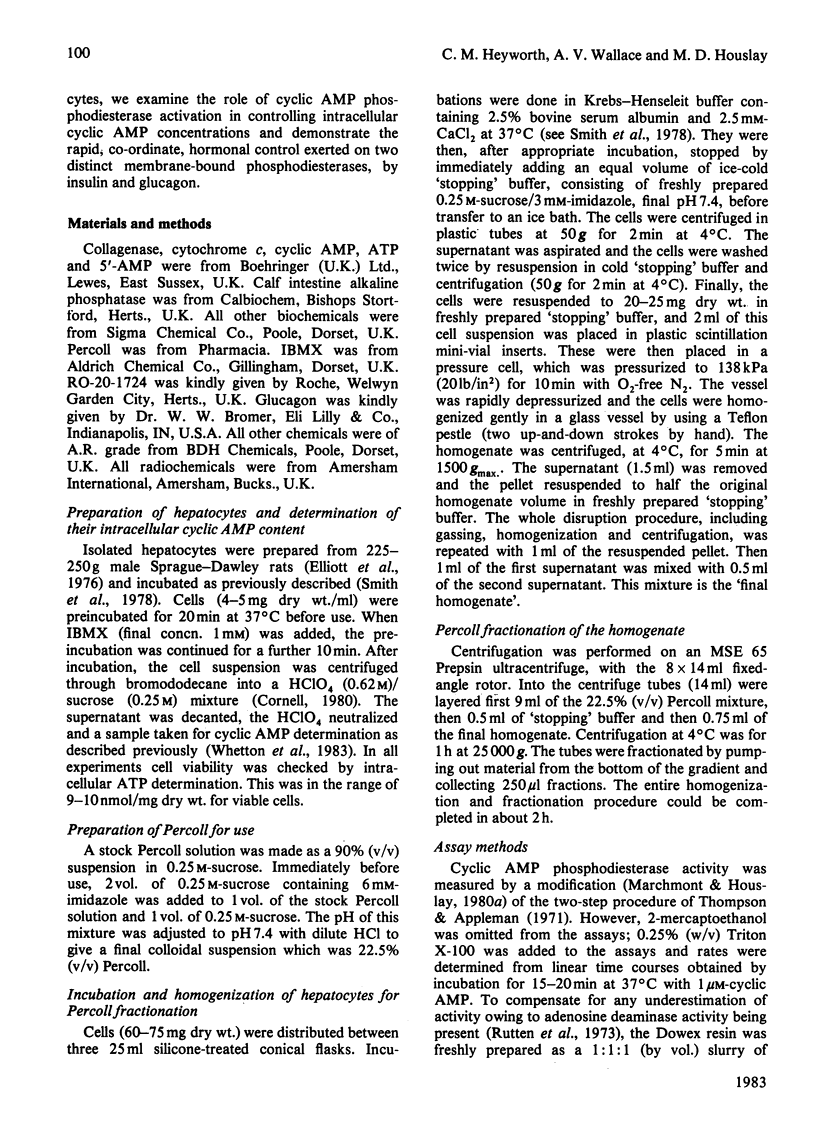
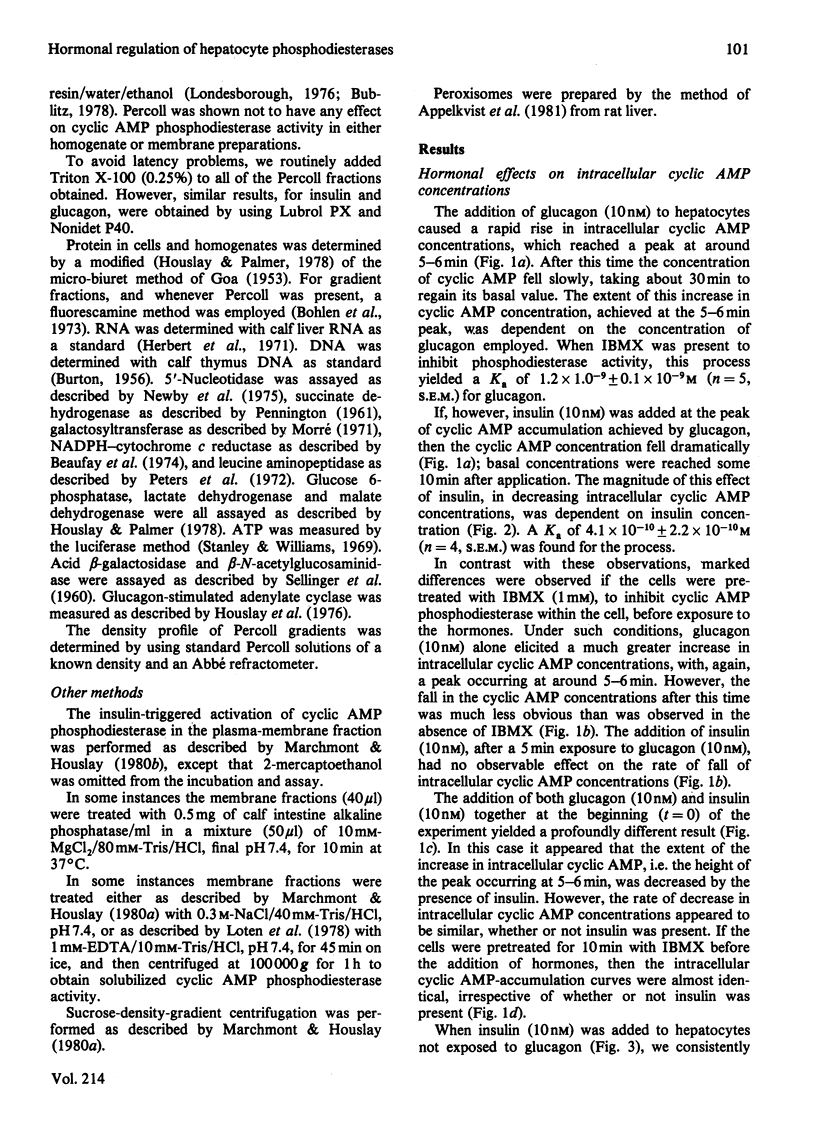
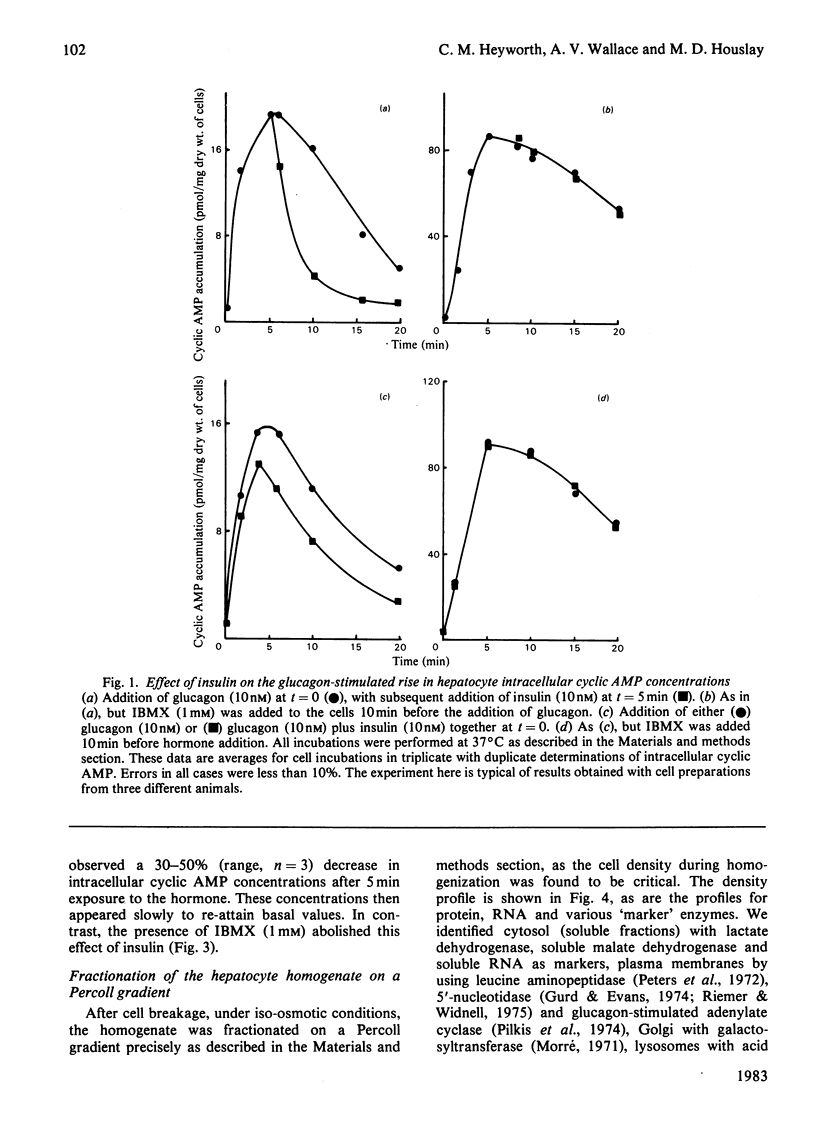
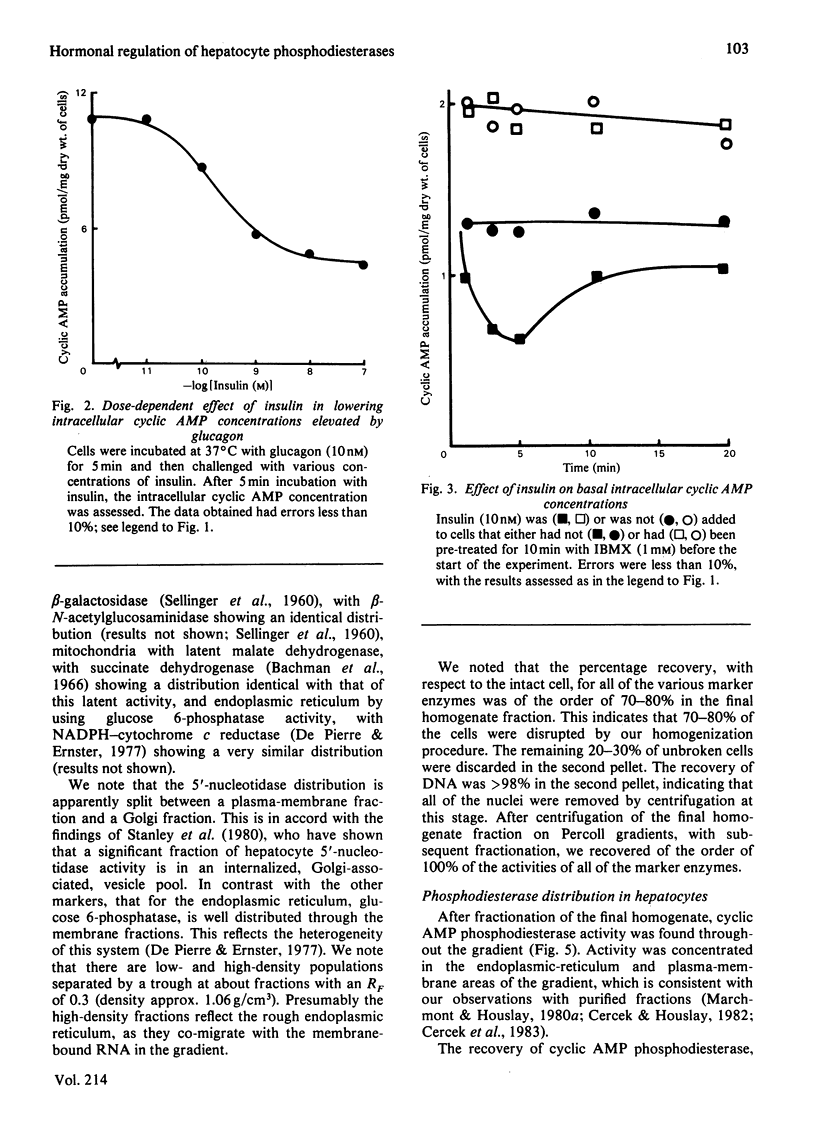
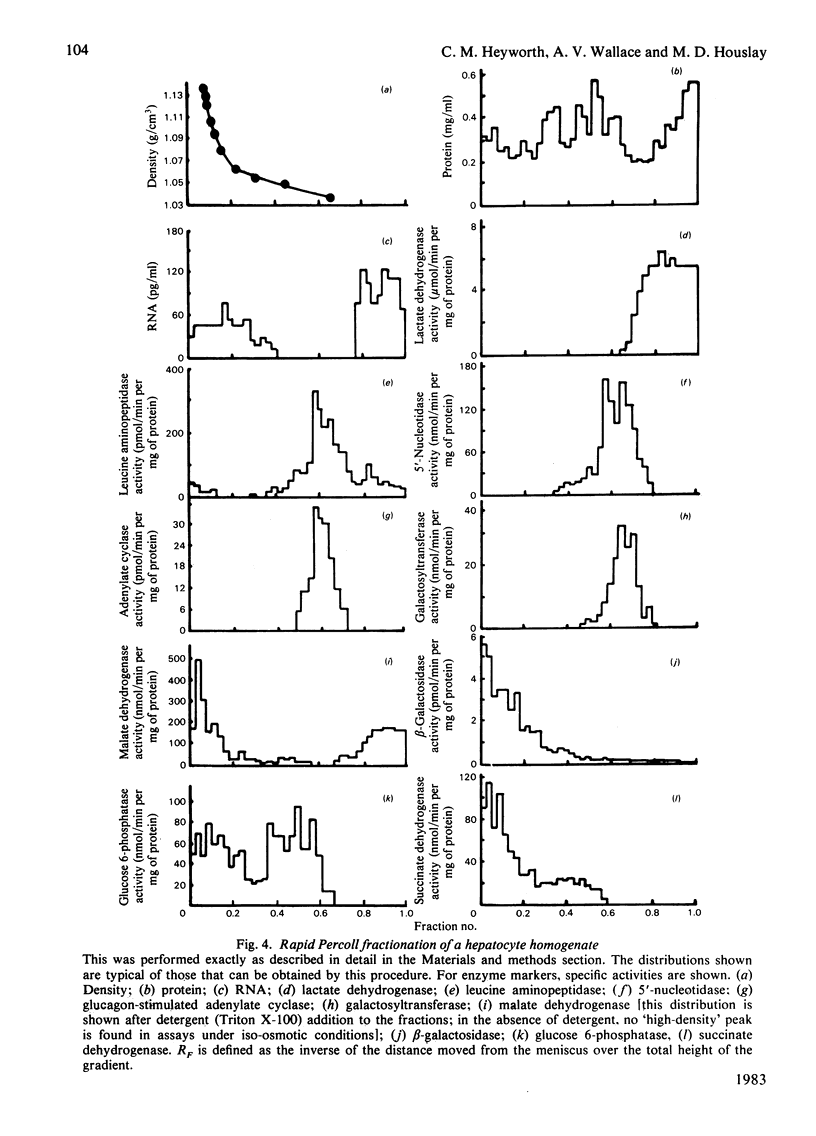
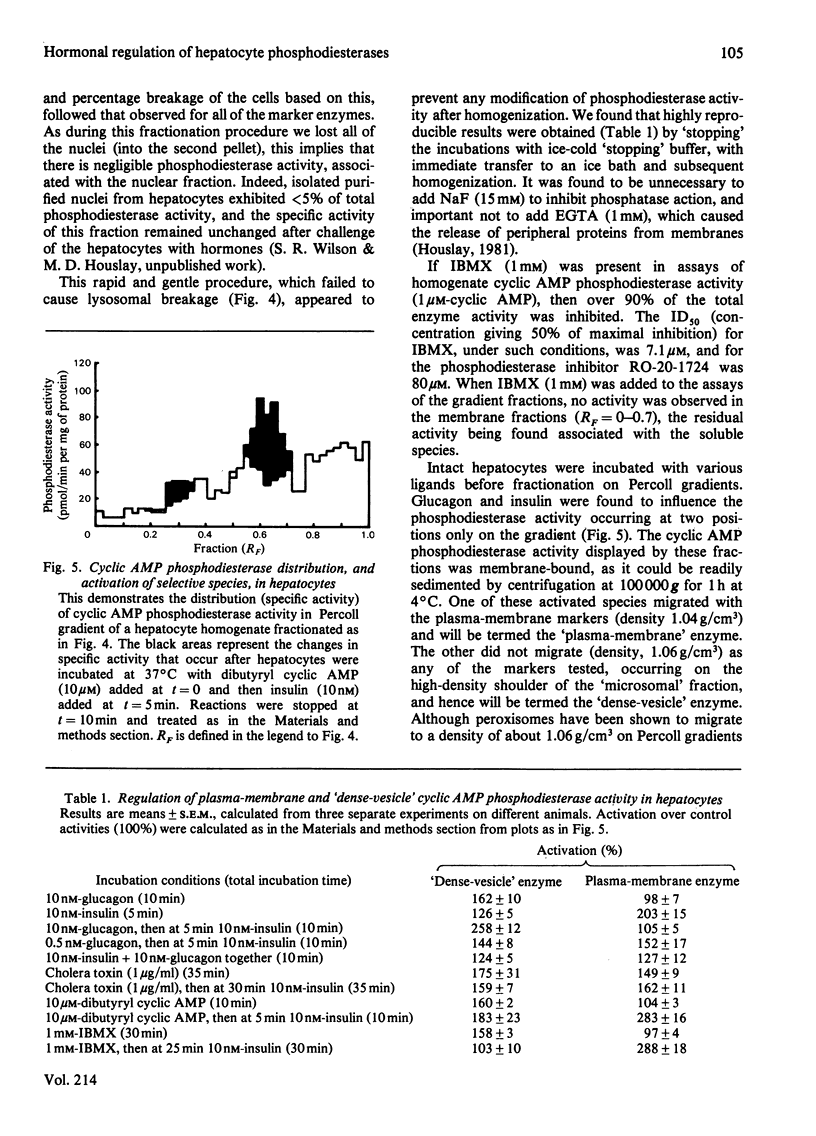
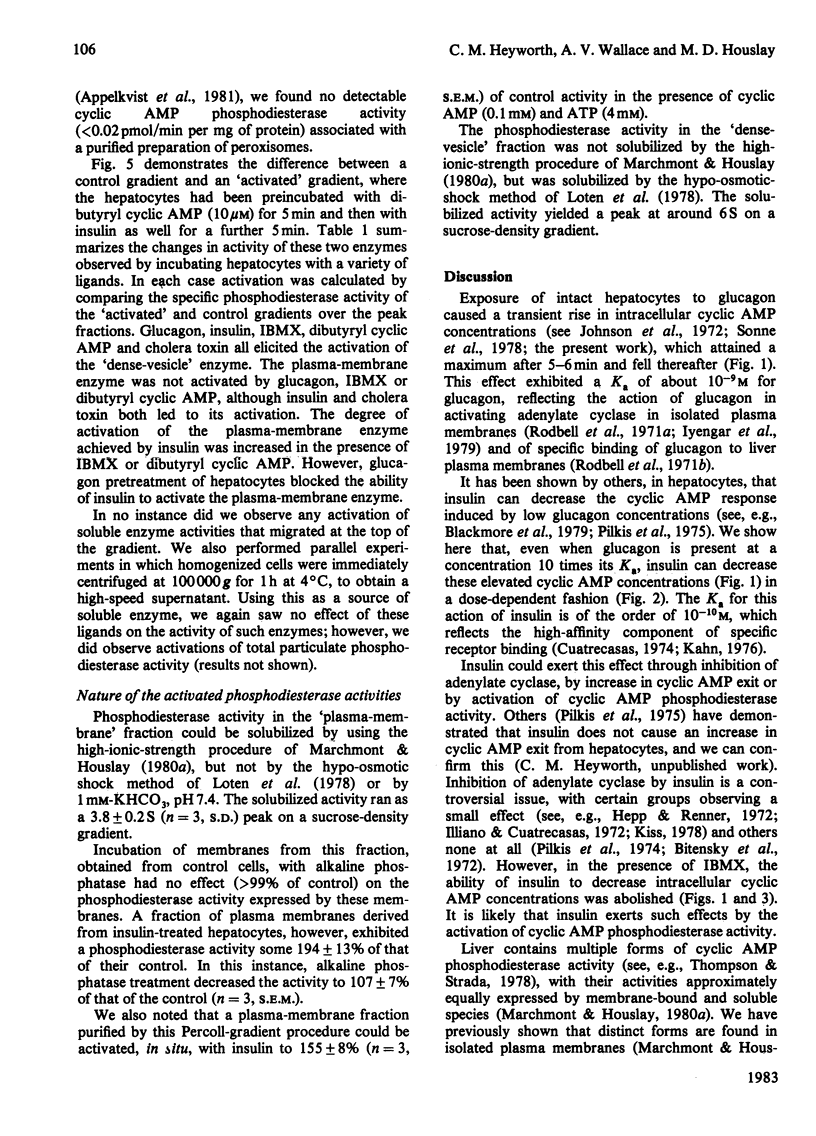
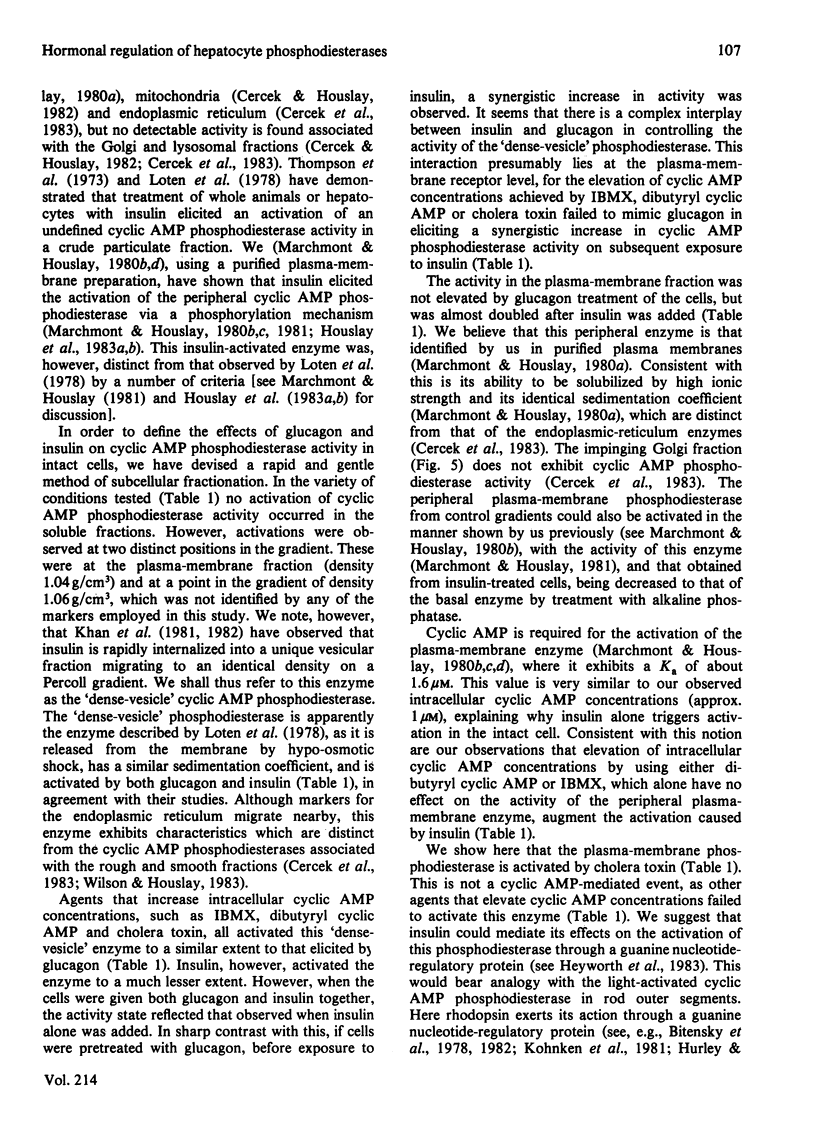
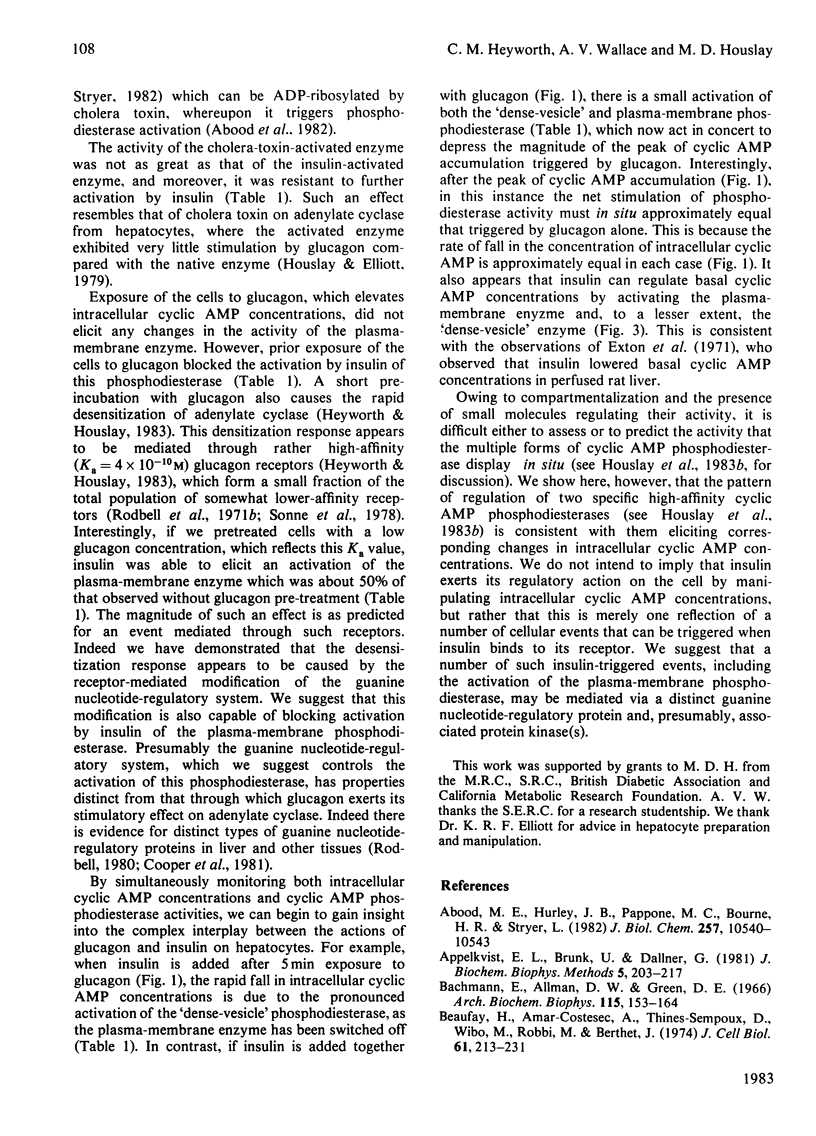
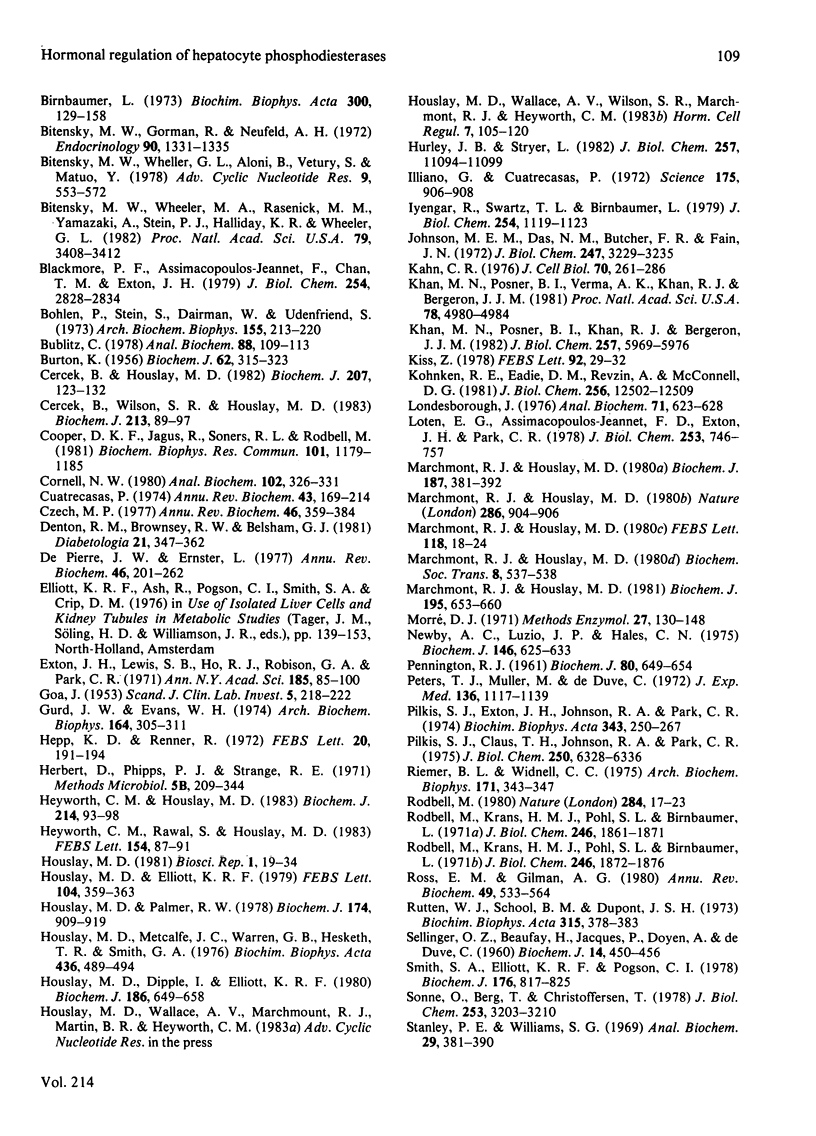
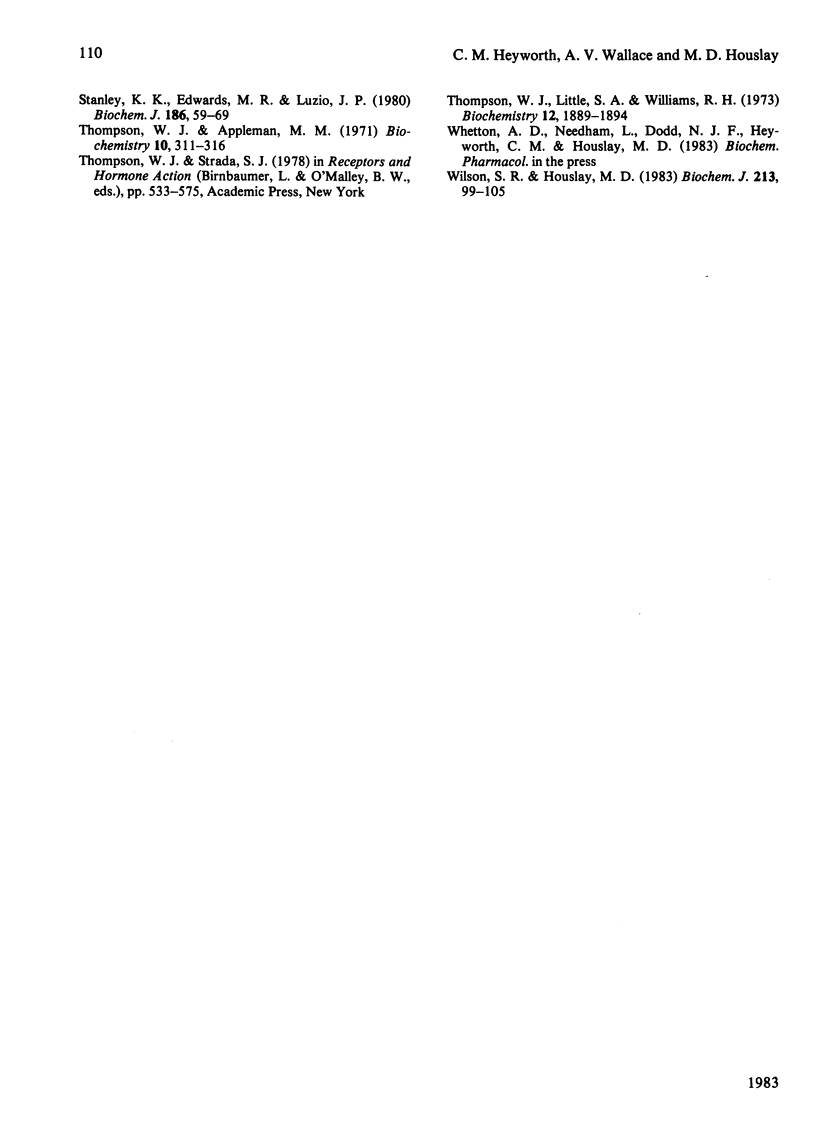
Selected References
These references are in PubMed. This may not be the complete list of references from this article.
- Abood M. E., Hurley J. B., Pappone M. C., Bourne H. R., Stryer L. Functional homology between signal-coupling proteins. Cholera toxin inactivates the GTPase activity of transducin. J Biol Chem. 1982 Sep 25;257(18):10540–10543. [PubMed] [Google Scholar]
- Appelkvist E. L., Brunk U., Dallner G. Isolation of peroxisomes from rat liver using sucrose and Percoll gradients. J Biochem Biophys Methods. 1981 Oct;5(4):203–217. doi: 10.1016/0165-022x(81)90045-2. [DOI] [PubMed] [Google Scholar]
- BURTON K. A study of the conditions and mechanism of the diphenylamine reaction for the colorimetric estimation of deoxyribonucleic acid. Biochem J. 1956 Feb;62(2):315–323. doi: 10.1042/bj0620315. [DOI] [PMC free article] [PubMed] [Google Scholar]
- Bachmann E., Allmann D. W., Green D. E. The membrane systems of the mitochondrion. I. The S fraction of the outer membrane of beef heart mitochondria. Arch Biochem Biophys. 1966 Jul;115(1):153–164. doi: 10.1016/s0003-9861(66)81051-2. [DOI] [PubMed] [Google Scholar]
- Beaufay H., Amar-Costesec A., Thinès-Sempoux D., Wibo M., Robbi M., Berthet J. Analytical study of microsomes and isolated subcellular membranes from rat liver. 3. Subfractionation of the microsomal fraction by isopycnic and differential centrifugation in density gradients. J Cell Biol. 1974 Apr;61(1):213–231. doi: 10.1083/jcb.61.1.213. [DOI] [PMC free article] [PubMed] [Google Scholar]
- Birnbaumer L. Hormone-sensitive adenylyl cyclases. Useful models for studying hormone receptor functions in cell-free systems. Biochim Biophys Acta. 1973 Sep 10;300(2):129–158. doi: 10.1016/0304-4157(73)90002-6. [DOI] [PubMed] [Google Scholar]
- Bitensky M. W., Wheeler G. L., Aloni B., Vetury S., Matuo Y. Light- and GTP-activated photoreceptor phosphodiesterase: regulation by a light-activated GTPase and identification of rhodopsin as the phosphodiesterase binding site. Adv Cyclic Nucleotide Res. 1978;9:553–572. [PubMed] [Google Scholar]
- Bitensky M. W., Wheeler M. A., Rasenick M. M., Yamazaki A., Stein P. J., Halliday K. R., Wheeler G. L. Functional exchange of components between light-activated photoreceptor phosphodiesterase and hormone-activated adenylate cyclase systems. Proc Natl Acad Sci U S A. 1982 Jun;79(11):3408–3412. doi: 10.1073/pnas.79.11.3408. [DOI] [PMC free article] [PubMed] [Google Scholar]
- Blackmore P. F., Assimacopoulos-Jeannet F., Chan T. M., Exton J. H. Studies on alpha-adrenergic activation of hepatic glucose output. Insulin inhibition of alpha-adrenergic and glucagon actions in normal and calcium-depleted hepatocytes. J Biol Chem. 1979 Apr 25;254(8):2828–2834. [PubMed] [Google Scholar]
- Bublitz C. A batch procedure for the assay of cyclic nucleotide phosphodiesterase. Anal Biochem. 1978 Jul 15;88(1):109–113. doi: 10.1016/0003-2697(78)90402-5. [DOI] [PubMed] [Google Scholar]
- Böhlen P., Stein S., Dairman W., Udenfriend S. Fluorometric assay of proteins in the nanogram range. Arch Biochem Biophys. 1973 Mar;155(1):213–220. doi: 10.1016/s0003-9861(73)80023-2. [DOI] [PubMed] [Google Scholar]
- Cercek B., Houslay M. D. Submitochondrial localization and asymmetric disposition of two peripheral cyclic nucleotide phosphodiesterases. Biochem J. 1982 Oct 1;207(1):123–132. doi: 10.1042/bj2070123. [DOI] [PMC free article] [PubMed] [Google Scholar]
- Cercek B., Wilson S. R., Houslay M. D. Heterogeneity of cyclic nucleotide phosphodiesterases in liver endoplasmic reticulum. Biochem J. 1983 Jul 1;213(1):89–97. doi: 10.1042/bj2130089. [DOI] [PMC free article] [PubMed] [Google Scholar]
- Cooper D. M., Jagus R., Somers R. L., Rodbell M. Cholera toxin modifies diverse GTP-modulated regulatory proteins. Biochem Biophys Res Commun. 1981 Aug 31;101(4):1179–1185. doi: 10.1016/0006-291x(81)91572-2. [DOI] [PubMed] [Google Scholar]
- Cornell N. W. Rapid fractionation of cell suspensions with the use of brominated hydrocarbons. Anal Biochem. 1980 Mar 1;102(2):326–331. doi: 10.1016/0003-2697(80)90162-1. [DOI] [PubMed] [Google Scholar]
- Cuatrecasas P. Membrane receptors. Annu Rev Biochem. 1974;43(0):169–214. doi: 10.1146/annurev.bi.43.070174.001125. [DOI] [PubMed] [Google Scholar]
- Czech M. P. Molecular basis of insulin action. Annu Rev Biochem. 1977;46:359–384. doi: 10.1146/annurev.bi.46.070177.002043. [DOI] [PubMed] [Google Scholar]
- DePierre J. W., Ernster L. Enzyme topology of intracellular membranes. Annu Rev Biochem. 1977;46:201–262. doi: 10.1146/annurev.bi.46.070177.001221. [DOI] [PubMed] [Google Scholar]
- Denton R. M., Brownsey R. W., Belsham G. J. A partial view of the mechanism of insulin action. Diabetologia. 1981 Oct;21(4):347–362. doi: 10.1007/BF00252681. [DOI] [PubMed] [Google Scholar]
- Exton J. H., Lewis S. B., Ho R. J., Robison G. A., Park C. R. The role of cyclic AMP in the interaction of glucagon and insulin in the control of liver metabolism. Ann N Y Acad Sci. 1971 Dec 30;185:85–100. doi: 10.1111/j.1749-6632.1971.tb45239.x. [DOI] [PubMed] [Google Scholar]
- GOA J. A micro biuret method for protein determination; determination of total protein in cerebrospinal fluid. Scand J Clin Lab Invest. 1953;5(3):218–222. doi: 10.3109/00365515309094189. [DOI] [PubMed] [Google Scholar]
- Gurd J. W., Evans W. H. Distribution of liver plasma membrane 5' nucleotidase as indicated by its reaction with anti-plasma membrane serum. Arch Biochem Biophys. 1974 Sep;164(1):305–311. doi: 10.1016/0003-9861(74)90035-6. [DOI] [PubMed] [Google Scholar]
- Hepp K. D., Renner R. Insulin action on the adenyl cyclase system: Antagonism to activation by lipolytic hormones. FEBS Lett. 1972 Feb 1;20(2):191–194. doi: 10.1016/0014-5793(72)80791-9. [DOI] [PubMed] [Google Scholar]
- Heyworth C. M., Houslay M. D. Challenge of hepatocytes by glucagon triggers a rapid modulation of adenylate cyclase activity in isolated membranes. Biochem J. 1983 Jul 15;214(1):93–98. doi: 10.1042/bj2140093. [DOI] [PMC free article] [PubMed] [Google Scholar]
- Heyworth C. M., Rawal S., Houslay M. D. Guanine nucleotides can activate the insulin-stimulated phosphodiesterase in liver plasma membranes. FEBS Lett. 1983 Apr 5;154(1):87–91. doi: 10.1016/0014-5793(83)80880-1. [DOI] [PubMed] [Google Scholar]
- Houslay M. D., Dipple I., Elliott K. R. Guanosine 5'-triphosphate and guanosine 5'-[beta gamma-imido]triphosphate effect a collision coupling mechanism between the glucagon receptor and catalytic unit of adenylate cyclase. Biochem J. 1980 Mar 15;186(3):649–658. doi: 10.1042/bj1860649. [DOI] [PMC free article] [PubMed] [Google Scholar]
- Houslay M. D., Elliott K. R. Cholera toxin mediated activation of adenylate cyclase in intact rat hepatocytes. FEBS Lett. 1979 Aug 15;104(2):359–363. doi: 10.1016/0014-5793(79)80852-2. [DOI] [PubMed] [Google Scholar]
- Houslay M. D., Metcalfe J. C., Warren G. B., Hesketh T. R., Smith G. A. The glucagon receptor of rat liver plasma membrane can couple to adenylate cyclase without activating it. Biochim Biophys Acta. 1976 Jun 17;436(2):489–494. doi: 10.1016/0005-2736(76)90210-8. [DOI] [PubMed] [Google Scholar]
- Houslay M. D., Palmer R. W. Changes in the form of Arrhenius plots of the activity of glucagon-stimulated adenylate cyclase and other hamster liver plasma-membrane enzymes occurring on hibernation. Biochem J. 1978 Sep 15;174(3):909–919. doi: 10.1042/bj1740909. [DOI] [PMC free article] [PubMed] [Google Scholar]
- Hurley J. B., Stryer L. Purification and characterization of the gamma regulatory subunit of the cyclic GMP phosphodiesterase from retinal rod outer segments. J Biol Chem. 1982 Sep 25;257(18):11094–11099. [PubMed] [Google Scholar]
- Illiano G., Cuatrecasas P. Modulation of adenylate cyclase activity in liver and fat cell membranes by insulin. Science. 1972 Feb 25;175(4024):906–908. doi: 10.1126/science.175.4024.906. [DOI] [PubMed] [Google Scholar]
- Iyengar R., Swartz T. L., Birnbaumer L. Coupling of glucagon receptor to adenylyl cyclase. Requirement of a receptor-related guanyl nucleotide binding site for coupling of receptor to the enzyme. J Biol Chem. 1979 Feb 25;254(4):1119–1123. [PubMed] [Google Scholar]
- Johnson M. E., Das N. M., Butcher F. R., Fain J. N. The regulation of gluconeogenesis in isolated rat liver cells by glucagon, insulin, dibutyryl cyclic adenosine monophosphate, and fatty acids. J Biol Chem. 1972 May 25;247(10):3229–3235. [PubMed] [Google Scholar]
- Kahn C. R. Membrane receptors for hormones and neurotransmitters. J Cell Biol. 1976 Aug;70(2 Pt 1):261–286. doi: 10.1083/jcb.70.2.261. [DOI] [PMC free article] [PubMed] [Google Scholar]
- Khan M. N., Posner B. I., Khan R. J., Bergeron J. J. Internalization of insulin into rat liver Golgi elements. Evidence for vesicle heterogeneity and the path of intracellular processing. J Biol Chem. 1982 May 25;257(10):5969–5976. [PubMed] [Google Scholar]
- Khan M. N., Posner B. I., Verma A. K., Khan R. J., Bergeron J. J. Intracellular hormone receptors: evidence for insulin and lactogen receptors in a unique vesicle sedimenting in lysosome fractions of rat liver. Proc Natl Acad Sci U S A. 1981 Aug;78(8):4980–4984. doi: 10.1073/pnas.78.8.4980. [DOI] [PMC free article] [PubMed] [Google Scholar]
- Kiss Z. Inhibition of the glucagon stimulated adenylate cyclase activity by insulin. FEBS Lett. 1978 Aug 1;92(1):29–32. doi: 10.1016/0014-5793(78)80714-5. [DOI] [PubMed] [Google Scholar]
- Londesborough J. Quantitative estimation of 3'5' cyclic AMP phosphodiesterase using anion exchange resin in a batch process. Anal Biochem. 1976 Apr;71(2):623–628. doi: 10.1016/s0003-2697(76)80037-1. [DOI] [PubMed] [Google Scholar]
- Loten E. G., Assimacopoulos-Jeannet F. D., Exton J. H., Park C. R. Stimulation of a low Km phosphodiesterase from liver by insulin and glucagon. J Biol Chem. 1978 Feb 10;253(3):746–757. [PubMed] [Google Scholar]
- Marchmont R. J., Houslay M. D. A peripheral and an intrinsic enzyme constitute the cyclic AMP phosphodiesterase activity of rat liver plasma membranes. Biochem J. 1980 May 1;187(2):381–392. doi: 10.1042/bj1870381. [DOI] [PMC free article] [PubMed] [Google Scholar]
- Marchmont R. J., Houslay M. D. Characterization of the phosphorylated form of the insulin-stimulated cyclic AMP phosphodiesterase from rat liver plasma membranes. Biochem J. 1981 Jun 1;195(3):653–660. doi: 10.1042/bj1950653. [DOI] [PMC free article] [PubMed] [Google Scholar]
- Marchmont R. J., Houslay M. D. Insulin controls the cyclic AMP-dependent phosphorylation of integral and peripheral proteins associated with the rat liver plasma membrane. FEBS Lett. 1980 Aug 25;118(1):18–24. doi: 10.1016/0014-5793(80)81209-9. [DOI] [PubMed] [Google Scholar]
- Marchmont R. J., Houslay M. D. Insulin trigger, cyclic AMP-dependent activation and phosphorylation of a plasma membrane cyclic AMP phosphodiesterase. Nature. 1980 Aug 28;286(5776):904–906. doi: 10.1038/286904a0. [DOI] [PubMed] [Google Scholar]
- Newby A. C., Luzio J. P., Hales C. N. The properties and extracellular location of 5'-nucleotidase of the rat fat-cell plasma membrane. Biochem J. 1975 Mar;146(3):625–633. doi: 10.1042/bj1460625. [DOI] [PMC free article] [PubMed] [Google Scholar]
- PENNINGTON R. J. Biochemistry of dystrophic muscle. Mitochondrial succinate-tetrazolium reductase and adenosine triphosphatase. Biochem J. 1961 Sep;80:649–654. doi: 10.1042/bj0800649. [DOI] [PMC free article] [PubMed] [Google Scholar]
- Peters T. J., Müller M., De Duve C. Lysosomes of the arterial wall. I. Isolation and subcellular fractionation of cells from normal rabbit aorta. J Exp Med. 1972 Nov 1;136(5):1117–1139. doi: 10.1084/jem.136.5.1117. [DOI] [PMC free article] [PubMed] [Google Scholar]
- Pilkis S. J., Claus T. H., Johnson R. A., Park C. R. Hormonal control of cyclic 3':5'-AMP levels and gluconeogenesis in isolated hepatocytes from fed rats. J Biol Chem. 1975 Aug 25;250(16):6328–6336. [PubMed] [Google Scholar]
- Pilkis S. J., Exton J. H., Johnson R. A., Park C. R. Effects of glucagon on cyclic AMP and carbohydrate metabolism in livers from diabetic rats. Biochim Biophys Acta. 1974 Mar 20;343(1):250–267. doi: 10.1016/0304-4165(74)90258-x. [DOI] [PubMed] [Google Scholar]
- Riemer B. L., Widnell C. C. The demonstration of a specific 5'-nucleotidase activity in rat tissues. Arch Biochem Biophys. 1975 Nov;171(1):343–347. doi: 10.1016/0003-9861(75)90041-7. [DOI] [PubMed] [Google Scholar]
- Rodbell M., Krans H. M., Pohl S. L., Birnbaumer L. The glucagon-sensitive adenyl cyclase system in plasma membranes of rat liver. 3. Binding of glucagon: method of assay and specificity. J Biol Chem. 1971 Mar 25;246(6):1861–1871. [PubMed] [Google Scholar]
- Rodbell M., Krans H. M., Pohl S. L., Birnbaumer L. The glucagon-sensitive adenyl cyclase system in plasma membranes of rat liver. IV. Effects of guanylnucleotides on binding of 125I-glucagon. J Biol Chem. 1971 Mar 25;246(6):1872–1876. [PubMed] [Google Scholar]
- Rodbell M. The role of hormone receptors and GTP-regulatory proteins in membrane transduction. Nature. 1980 Mar 6;284(5751):17–22. doi: 10.1038/284017a0. [DOI] [PubMed] [Google Scholar]
- Ross E. M., Gilman A. G. Biochemical properties of hormone-sensitive adenylate cyclase. Annu Rev Biochem. 1980;49:533–564. doi: 10.1146/annurev.bi.49.070180.002533. [DOI] [PubMed] [Google Scholar]
- SELLINGER O. Z., BEAUFAY H., JACQUES P., DOYEN A., DE DUVE C. Tissue fractionation studies. 15. Intracellular distribution and properties of beta-N-acetylglucosaminidase and beta-galactosidase in rat liver. Biochem J. 1960 Mar;74:450–456. doi: 10.1042/bj0740450. [DOI] [PMC free article] [PubMed] [Google Scholar]
- Smith S. A., Elliott K. R., Pogson C. I. Differential effects of tryptophan on glucose synthesis in rats and guinea pigs. Biochem J. 1978 Dec 15;176(3):817–825. doi: 10.1042/bj1760817. [DOI] [PMC free article] [PubMed] [Google Scholar]
- Sonne O., Berg T., Christoffersen T. Binding of 125I-labeled glucagon and glucagon-stimulated accumulation of adenosine 3':5'-monophosphate in isolated intact rat hepatocytes. Evidence for receptor heterogeneity. J Biol Chem. 1978 May 10;253(9):3203–3210. [PubMed] [Google Scholar]
- Stanley K. K., Edwards M. R., Luzio J. P. Subcellular distribution and movement of 5'-nucleotidase in rat cells. Biochem J. 1980 Jan 15;186(1):59–69. doi: 10.1042/bj1860059. [DOI] [PMC free article] [PubMed] [Google Scholar]
- Stanley P. E., Williams S. G. Use of the liquid scintillation spectrometer for determining adenosine triphosphate by the luciferase enzyme. Anal Biochem. 1969 Jun;29(3):381–392. doi: 10.1016/0003-2697(69)90323-6. [DOI] [PubMed] [Google Scholar]
- Thompson W. J., Appleman M. M. Multiple cyclic nucleotide phosphodiesterase activities from rat brain. Biochemistry. 1971 Jan 19;10(2):311–316. [PubMed] [Google Scholar]
- Thompson W. J., Little S. A., Williams R. H. Effect of insulin and growth hormone on rat liver cyclic nucleotide phosphodiesterase. Biochemistry. 1973 May 8;12(10):1889–1894. doi: 10.1021/bi00734a008. [DOI] [PubMed] [Google Scholar]
- Wilson S. R., Houslay M. D. Proteolytic activation and solubilization of endoplasmic-reticulum cyclic AMP phosphodiesterase activity. Biochem J. 1983 Jul 1;213(1):99–105. doi: 10.1042/bj2130099. [DOI] [PMC free article] [PubMed] [Google Scholar]