Abstract
The properties of Na+-dependent L-alanine transport in human erythrocytes were investigated using K+ as the Na+ substitute. Initial rates of Na+-dependent L-alanine uptake (0.2 mM extracellular amino acid) for erythrocytes from 22 donors ranged from 40 to 180 mumol/litre of cells per h at 37 degrees C. Amino acid uptake over the concentration range 0.1-8 mM was consistent with a single saturable component of Na+-dependent L-alanine transport. Apparent Km and Vmax. values at 37 and 5 degrees C measured in erythrocytes from the same donor were 0.27 and 0.085 mM respectively, and 270 and 8.5 mumol/litre of cells per h respectively. The transporter responsible for this uptake was identified as system ASC on the basis of cross-inhibition studies with a series of 42 amino acids and amino acid analogues. Apparent Ki values for glycine, L-alpha-amino-n-butyrate, L-serine and L-leucine as inhibitors of Na+-dependent L-alanine uptake at 37 degrees C were 4.2, 0.12, 0.16 and 0.70 mM respectively. Reticulocytes from a patient with inherited pyruvate kinase deficiency were found to have a 10-fold elevated activity of Na+-dependent L-alanine uptake compared with erythrocytes from normal donors. Separation of erythrocytes according to cell density (cell age) established that even the oldest mature erythrocytes retained significant Na+-dependent L-alanine transport activity. Amino acid transport was, however, a more sensitive indicator of cell age than acetylcholinesterase activity. Erythrocytes were found to accumulate L-alanine against its concentration gradient (distribution ratio approx. 1.5 after 4 h incubation), an effect that was abolished in Na+-free media. Na+-dependent L-alanine uptake was shown to be associated with L-alanine-dependent Na+ influx, the measured coupling ratio being 1:1.
Full text
PDF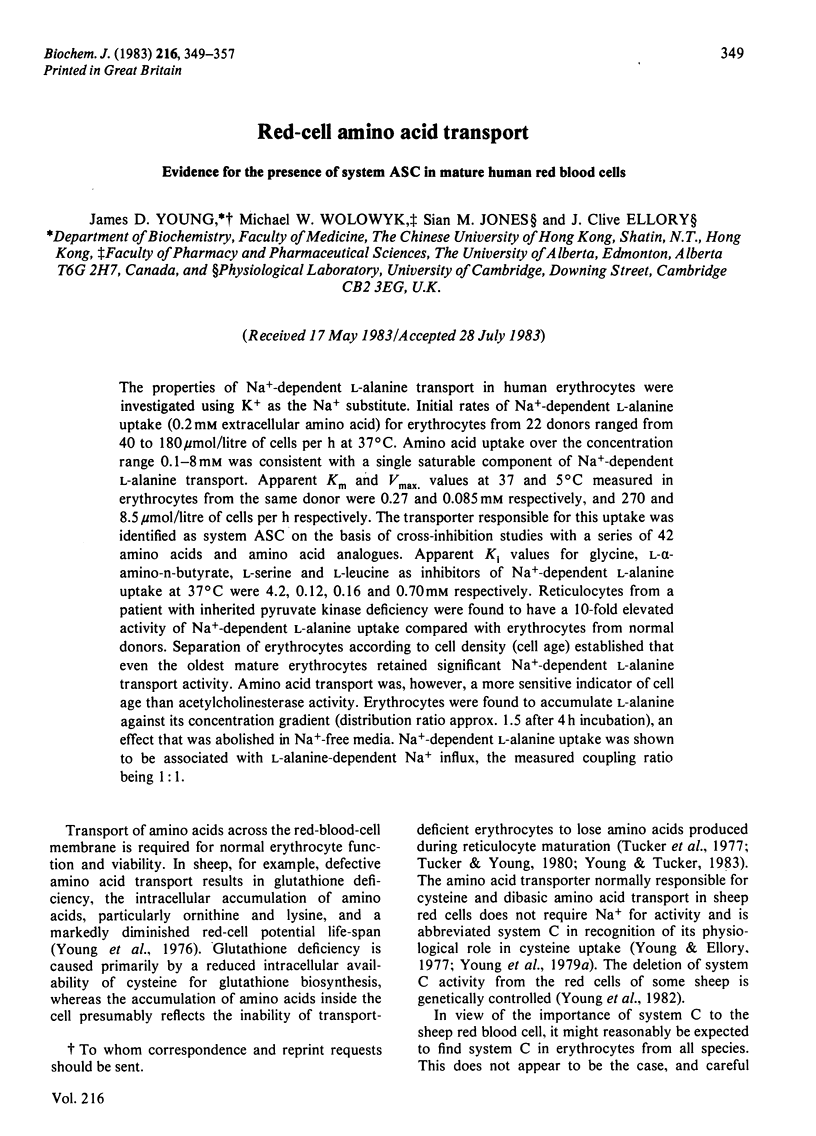
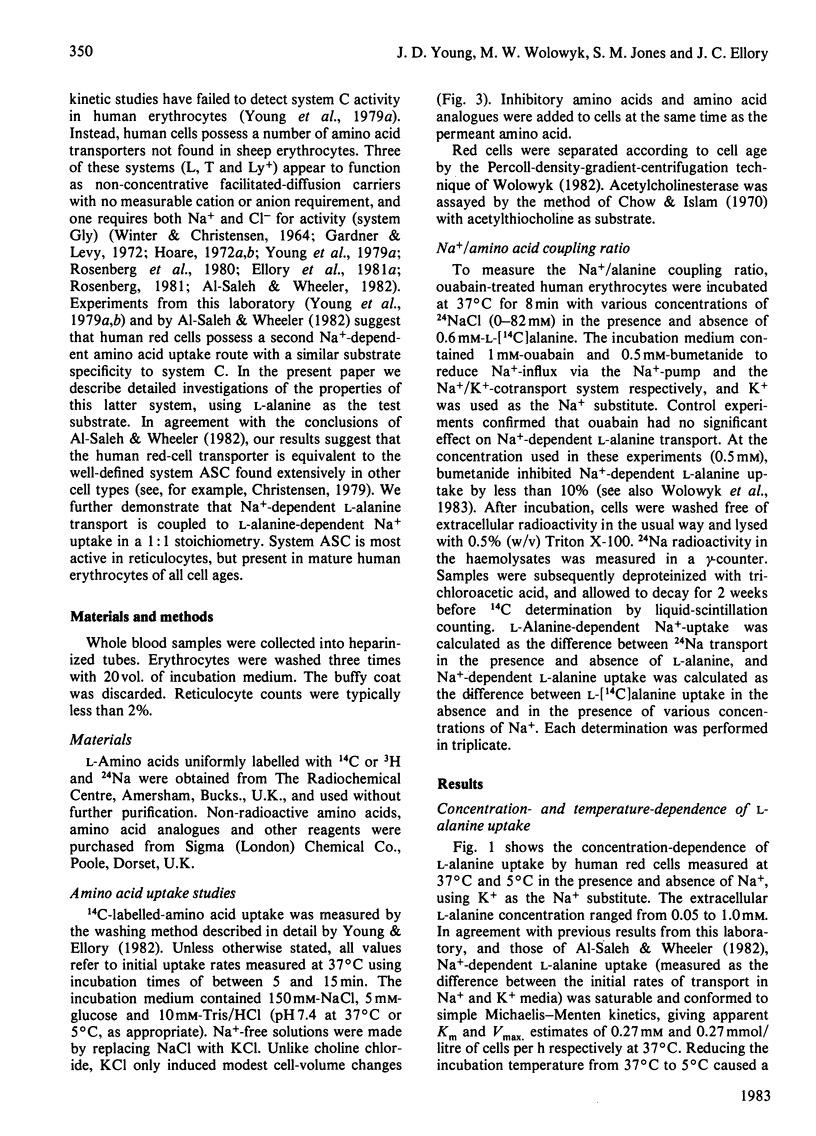
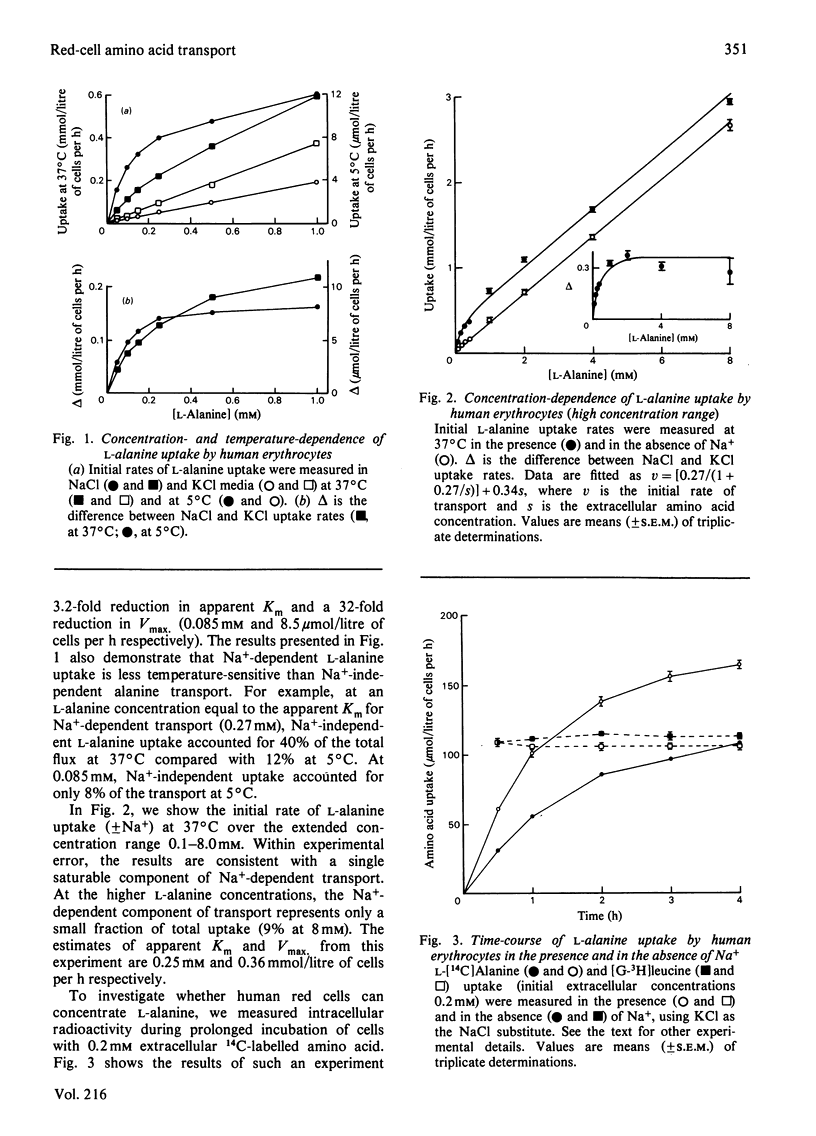
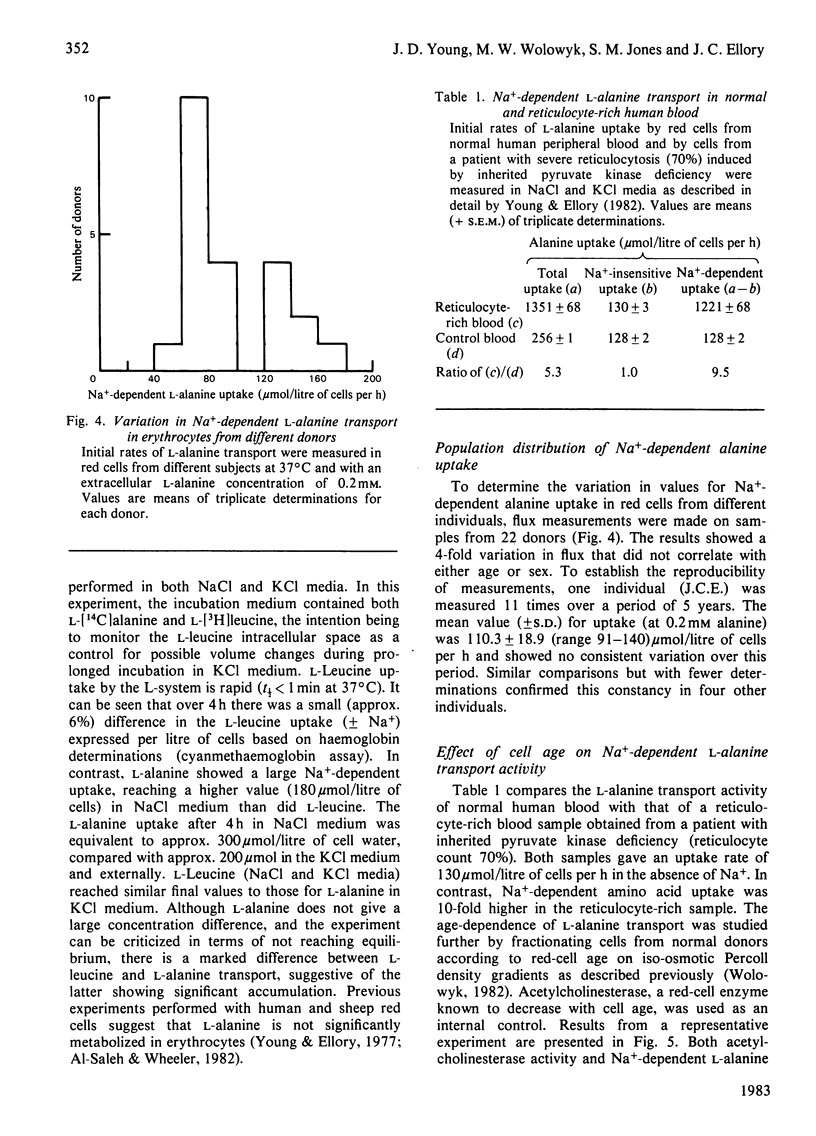
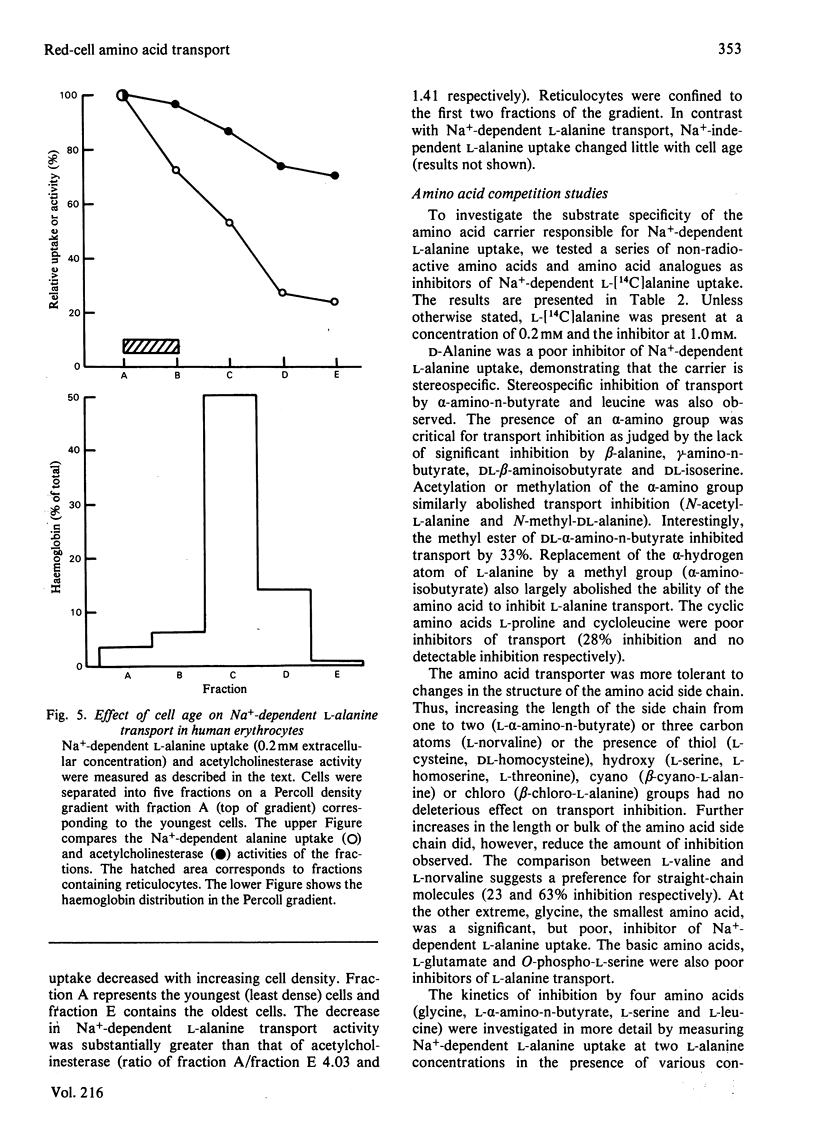
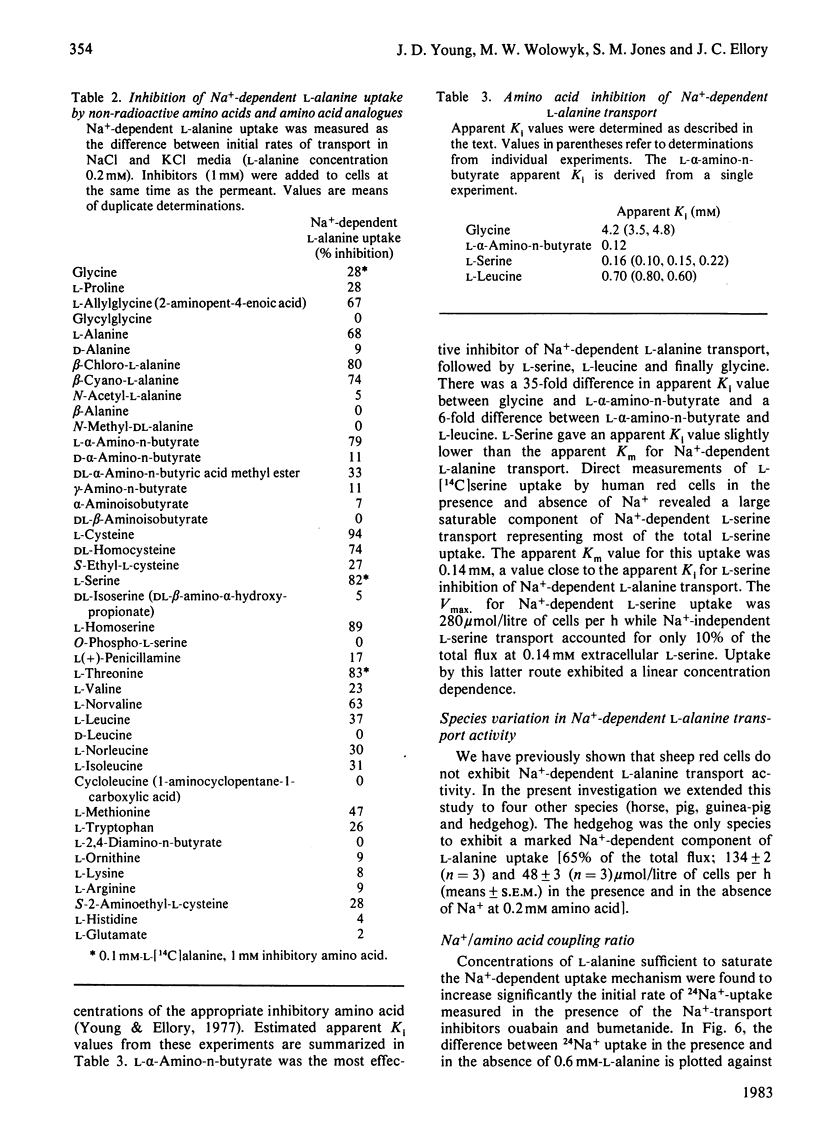
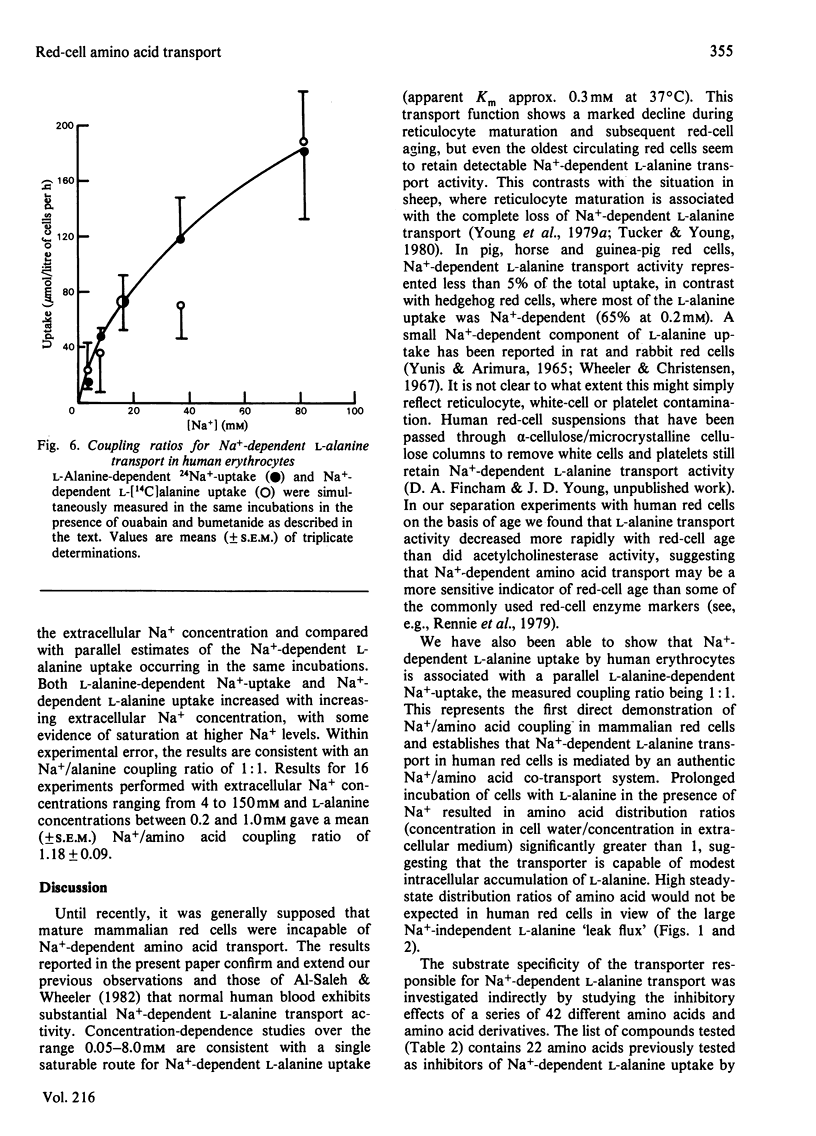
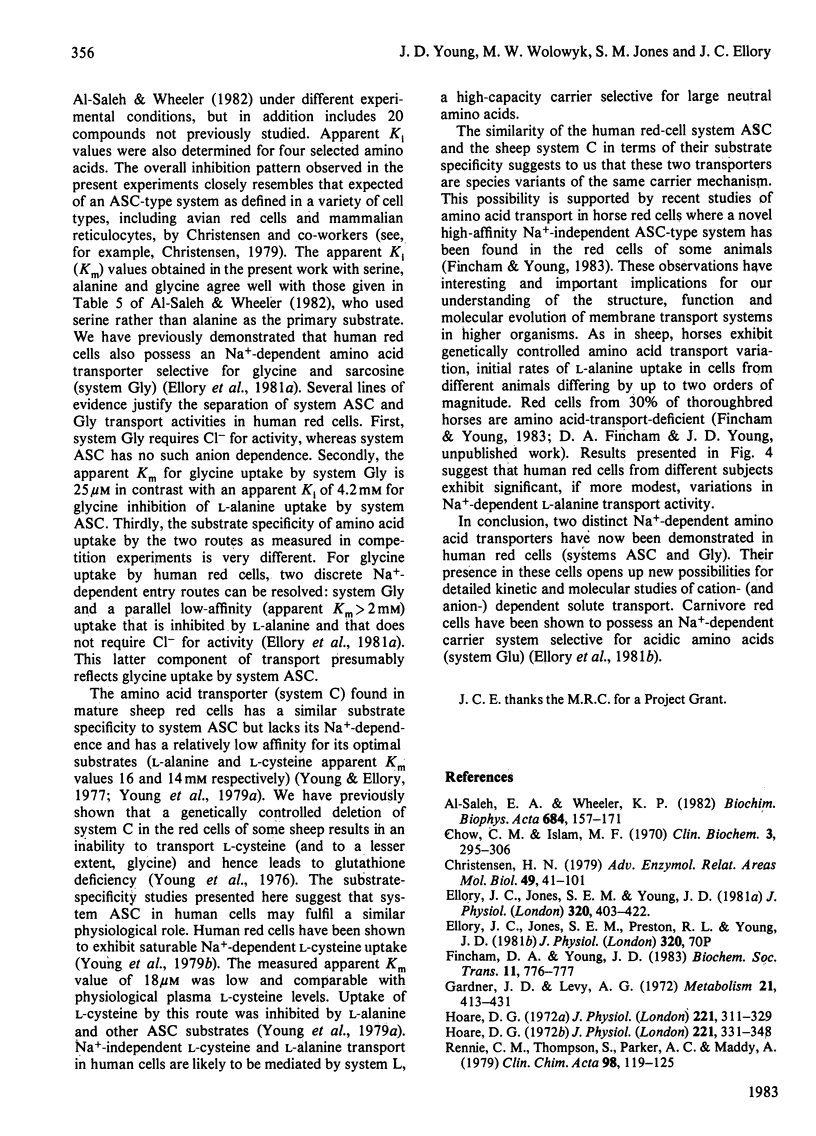
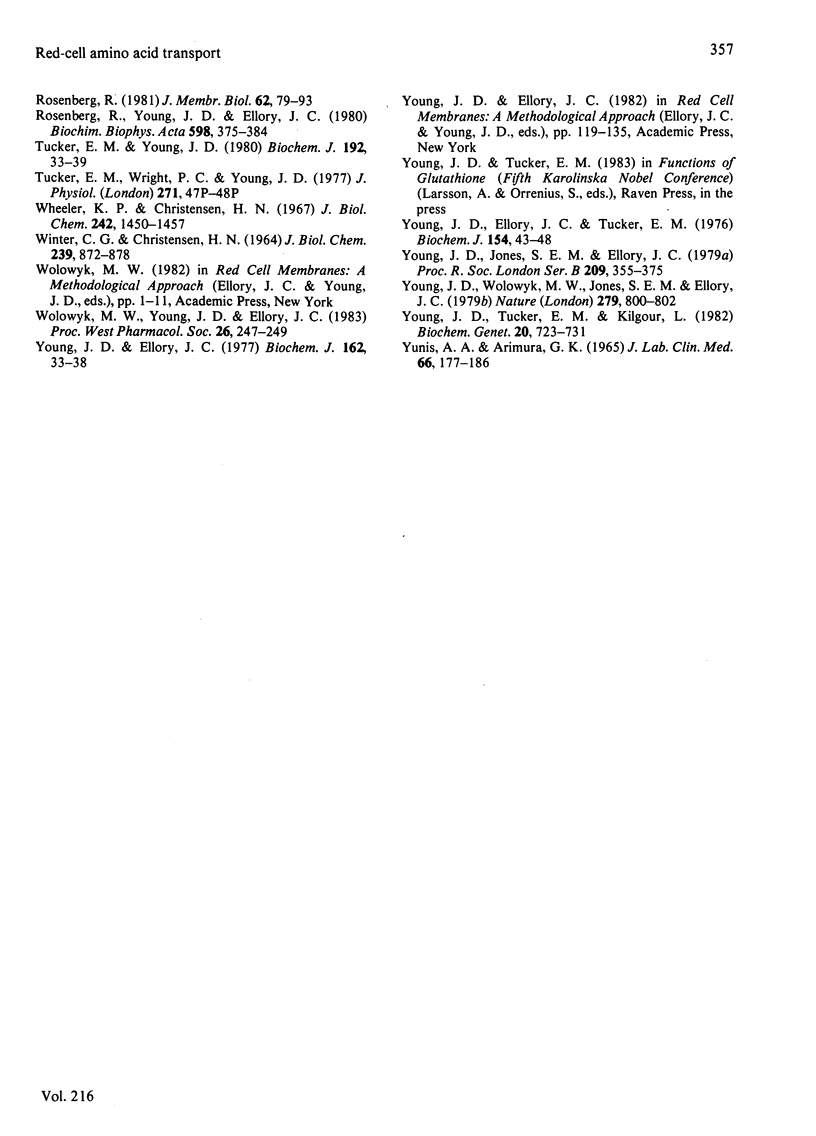
Selected References
These references are in PubMed. This may not be the complete list of references from this article.
- Al-Saleh E. A., Wheeler K. P. Transport of neutral amino acids by human erythrocytes. Biochim Biophys Acta. 1982 Jan 22;684(2):157–171. doi: 10.1016/0005-2736(82)90001-3. [DOI] [PubMed] [Google Scholar]
- Chow C. M., Islam M. F. Colorimetric determination of red blood cell acetylcholinesterase activity. Clin Biochem. 1970 Dec;3(4):295–306. [PubMed] [Google Scholar]
- Christensen H. N. Exploiting amino acid structure to learn about membrane transport. Adv Enzymol Relat Areas Mol Biol. 1979;49:41–101. doi: 10.1002/9780470122945.ch2. [DOI] [PubMed] [Google Scholar]
- Gardner J. D., Levy A. G. Transport of dibasic amino acids by human erythrocytes. Metabolism. 1972 May;21(5):413–431. doi: 10.1016/0026-0495(72)90054-6. [DOI] [PubMed] [Google Scholar]
- Hoare D. G. The temperature dependence of the transport of L-leucine in human erythrocytes. J Physiol. 1972 Mar;221(2):331–348. doi: 10.1113/jphysiol.1972.sp009754. [DOI] [PMC free article] [PubMed] [Google Scholar]
- Hoare D. G. The transport of L-leucine in human erythrocytes: a new kinetic analysis. J Physiol. 1972 Mar;221(2):311–329. doi: 10.1113/jphysiol.1972.sp009753. [DOI] [PMC free article] [PubMed] [Google Scholar]
- Rennie C. M., Thompson S., Parker A. C., Maddy A. Human erythrocyte fraction in "Percoll" density gradients. Clin Chim Acta. 1979 Oct 15;98(1-2):119–125. doi: 10.1016/0009-8981(79)90172-4. [DOI] [PubMed] [Google Scholar]
- Rosenberg R. L-Leucine transport in human red blood cells: a detailed kinetic analysis. J Membr Biol. 1981;62(1-2):79–93. doi: 10.1007/BF01870202. [DOI] [PubMed] [Google Scholar]
- Rosenberg R., Young J. D., Ellory J. C. L-Tryptophan transport in human red blood cells. Biochim Biophys Acta. 1980 May 23;598(2):375–384. doi: 10.1016/0005-2736(80)90015-2. [DOI] [PubMed] [Google Scholar]
- Tucker E. M., Young J. D. Biochemical changes during reticulocyte maturation in culture. A comparison of genetically different sheep erythrocytes. Biochem J. 1980 Oct 15;192(1):33–39. doi: 10.1042/bj1920033. [DOI] [PMC free article] [PubMed] [Google Scholar]
- WINTER C. G., CHRISTENSEN H. N. MIGRATION OF AMINO ACIDS ACROSS THE MEMBRANE OF THE HUMAN ERYTHROCYTE. J Biol Chem. 1964 Mar;239:872–878. [PubMed] [Google Scholar]
- Wheeler K. P., Christensen H. N. Role of na+ in the transport of amino acids in rabbit red cells. J Biol Chem. 1967 Apr 10;242(7):1450–1457. [PubMed] [Google Scholar]
- Wolowyk M. W., Young J. D., Ellory J. C. Inhibition of amino acid transport by loop diuretics. Proc West Pharmacol Soc. 1983;26:247–249. [PubMed] [Google Scholar]
- YUNIS A. A., ARIMURA G. K. AMINO ACID TRANSPORT IN BLOOD CELLS. II. PATTERNS OF TRANSPORT OF SOME AMINO ACIDS IN MAMMALIAN RETICULOCYTES AND MATURE RED BLOOD CELLS. J Lab Clin Med. 1965 Aug;66:177–186. [PubMed] [Google Scholar]
- Young J. D., Ellory J. C. Substrate specificity of amino acid transport in sheep erythrocytes. Biochem J. 1977 Jan 15;162(1):33–38. doi: 10.1042/bj1620033. [DOI] [PMC free article] [PubMed] [Google Scholar]
- Young J. D., Ellory J. C., Tucker E. M. Amino acid transport in normal and glutathione-deficient sheep erythrocytes. Biochem J. 1976 Jan 15;154(1):43–48. doi: 10.1042/bj1540043. [DOI] [PMC free article] [PubMed] [Google Scholar]
- Young J. D., Jones S. E., Ellory J. C. Amino acid transport in human and in sheep erythrocytes. Proc R Soc Lond B Biol Sci. 1980 Sep 26;209(1176):355–375. doi: 10.1098/rspb.1980.0100. [DOI] [PubMed] [Google Scholar]
- Young J. D., Tucker E. M., Kilgour L. Genetic control of amino acid transport in sheep erythrocytes. Biochem Genet. 1982 Aug;20(7-8):723–731. doi: 10.1007/BF00483969. [DOI] [PubMed] [Google Scholar]
- Young J. D., Wolowyk M. W., Jones S. E., Ellory J. C. Sodium-dependent cysteine transport in human red blood cells. Nature. 1979 Jun 28;279(5716):800–802. doi: 10.1038/279800a0. [DOI] [PubMed] [Google Scholar]