Abstract
Rats were fed on a diet containing 1% beta-guanidinopropionic acid (GPA), a creatine substrate analogue, for 6-10 weeks to deplete their muscle of creatine. This manipulation was previously shown to give a 90% decrease in [phosphocreatine] in skeletal and cardiac muscle and a 50% decrease in [ATP] in skeletal muscle only. Maximal activities of creatine kinase and of representative enzymes of aerobic and anaerobic energy metabolism were measured in the superficial white, medial and deep red portions of the gastrocnemius muscle, in the soleus and plantaris muscle and in the heart. Fast-twitch muscles were smaller in GPA-fed animals than in controls, but the size of the soleus muscle was unchanged. The activities of aerobic enzymes increased by 30-40% in all fast-twitch muscle regions except the superficial gastrocnemius, but were unchanged in the soleus muscle. The activities of creatine kinase and phosphofructokinase decreased by 20-50% in all skeletal-muscle regions except the deep gastrocnemius, and the activity of glycogen phosphorylase generally paralleled these changes. There were no significant changes in the activities of any of the enzymes measured in the heart. The glycogen content of the gastrocnemius-plantaris complex was increased by 185% in GPA-fed rats. The proportion of Type I fibres in the soleus muscle increased from 81% in control rats to 100% in GPA-fed rats, consistent with a previous report of altered isometric twitch characteristics and a decrease in the maximum velocity of shortening in this muscle [Petrofsky & Fitch (1980) Pflugers Arch. 384, 123-129]. We conclude that fast-twitch muscles adapt by a combination of decreasing diffusion distances, increasing aerobic capacity and decreasing glycolytic potential. Slow-twitch muscles decrease glycolytic potential and become slower, thus decreasing energy demand. These results suggest that persistent changes in the [phosphocreatine] and [ATP] are alone sufficient to alter the expression of enzyme proteins and proteins of the contractile apparatus, and that fibre-type-specific thresholds exist for the transformation response.
Full text
PDF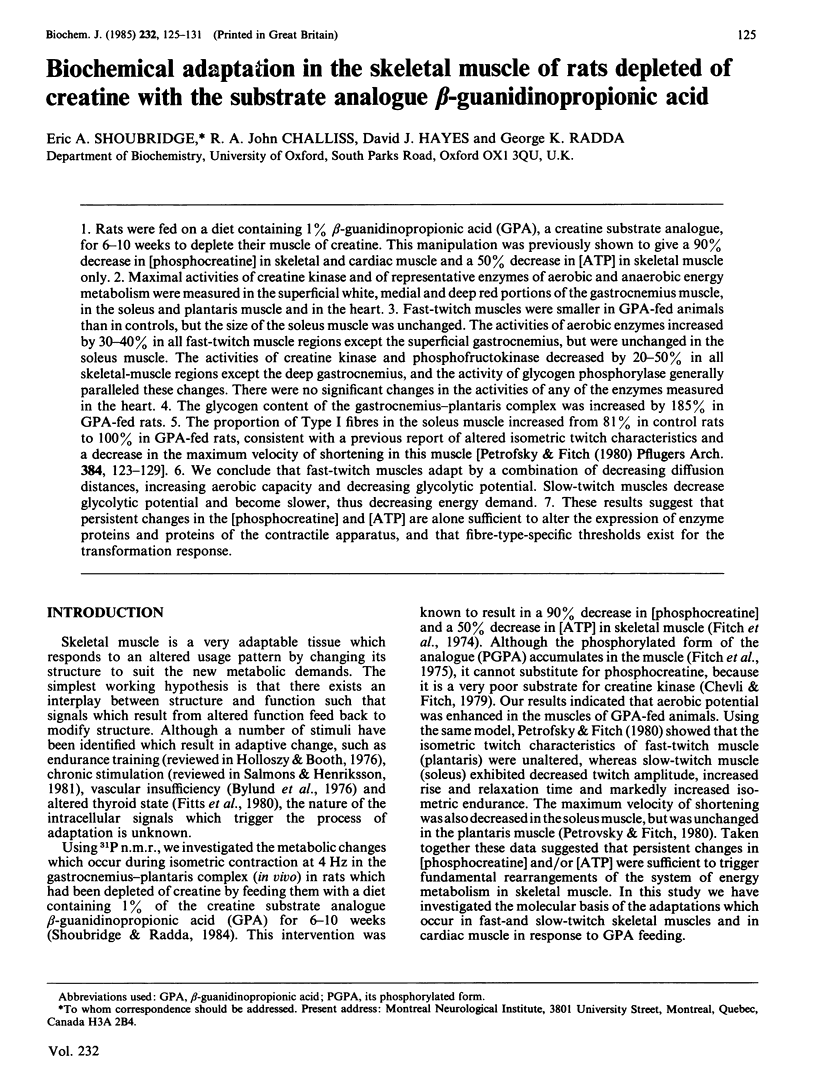
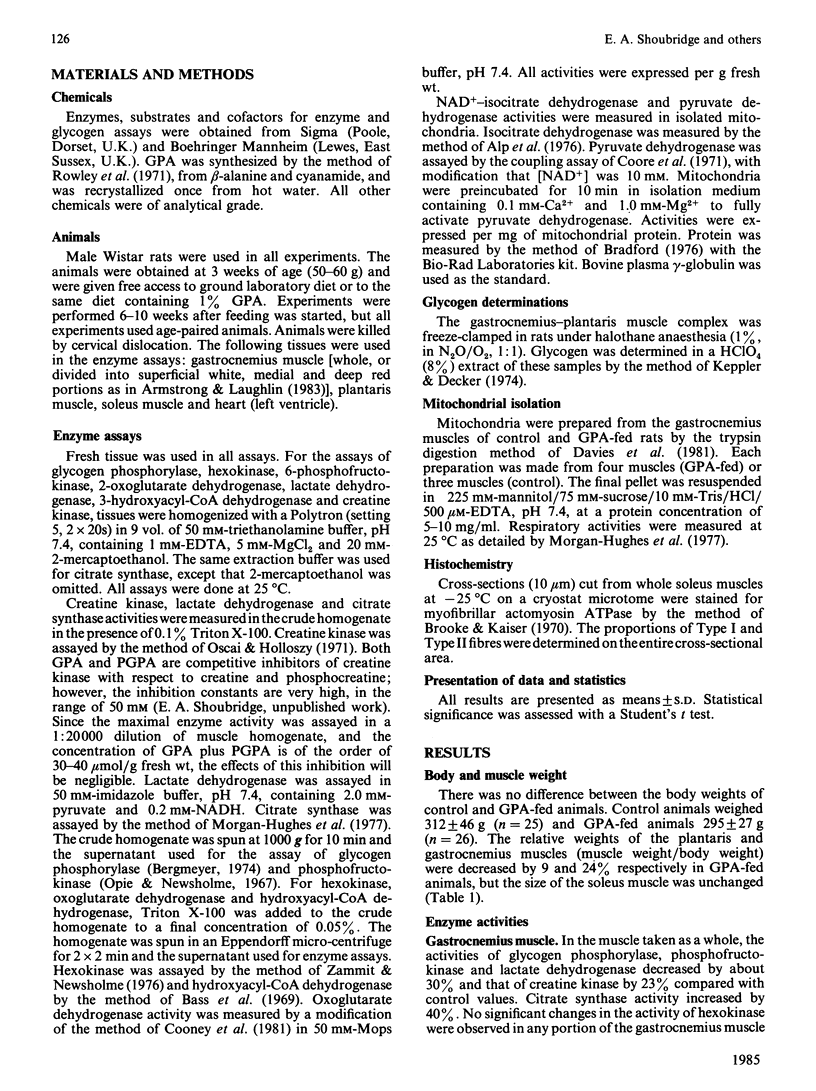
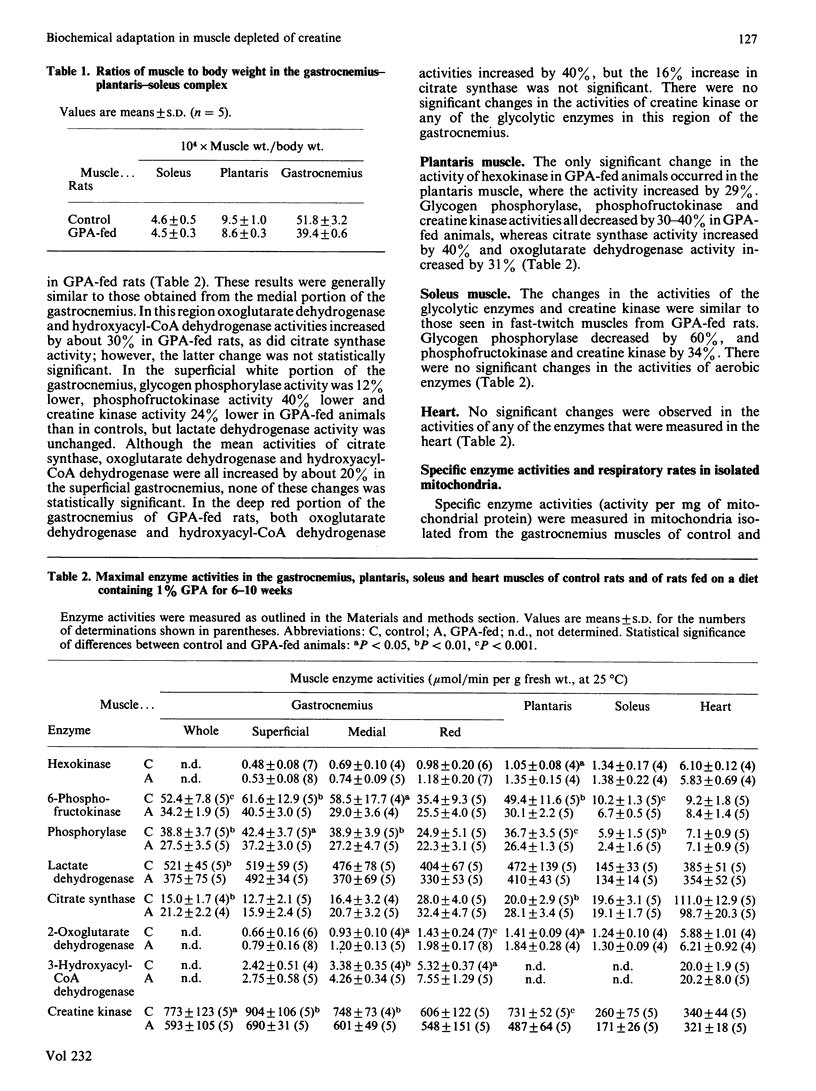
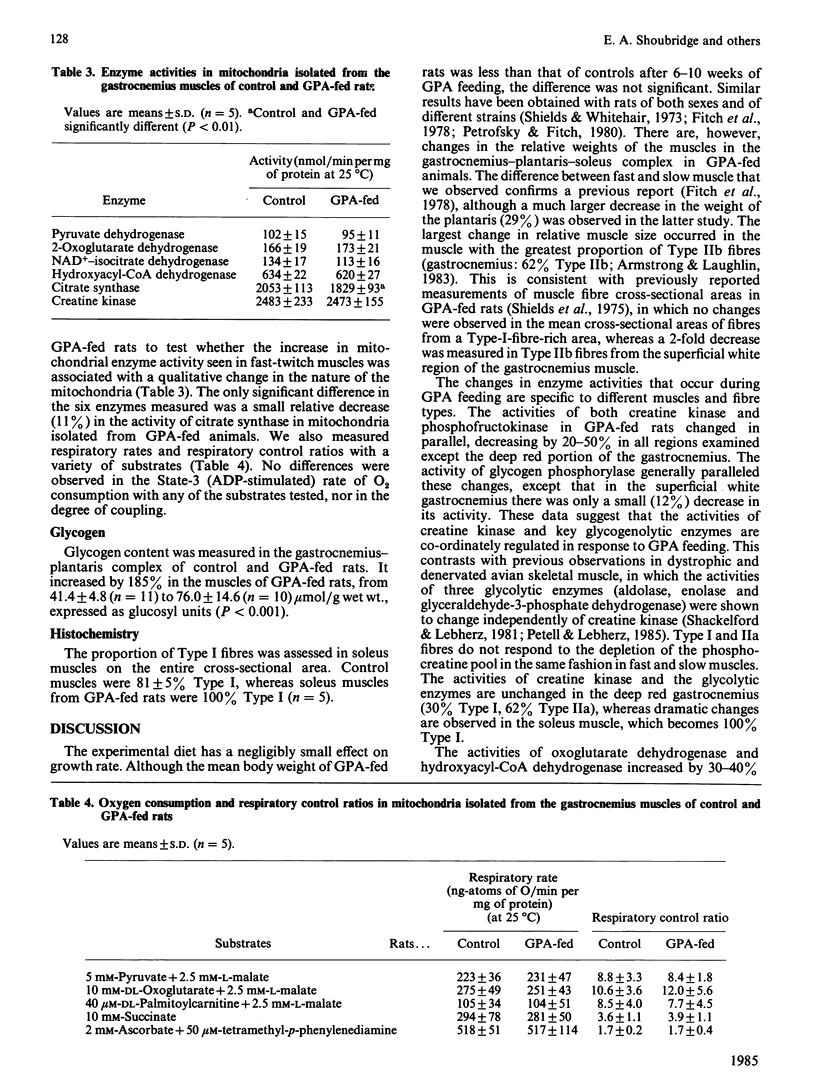
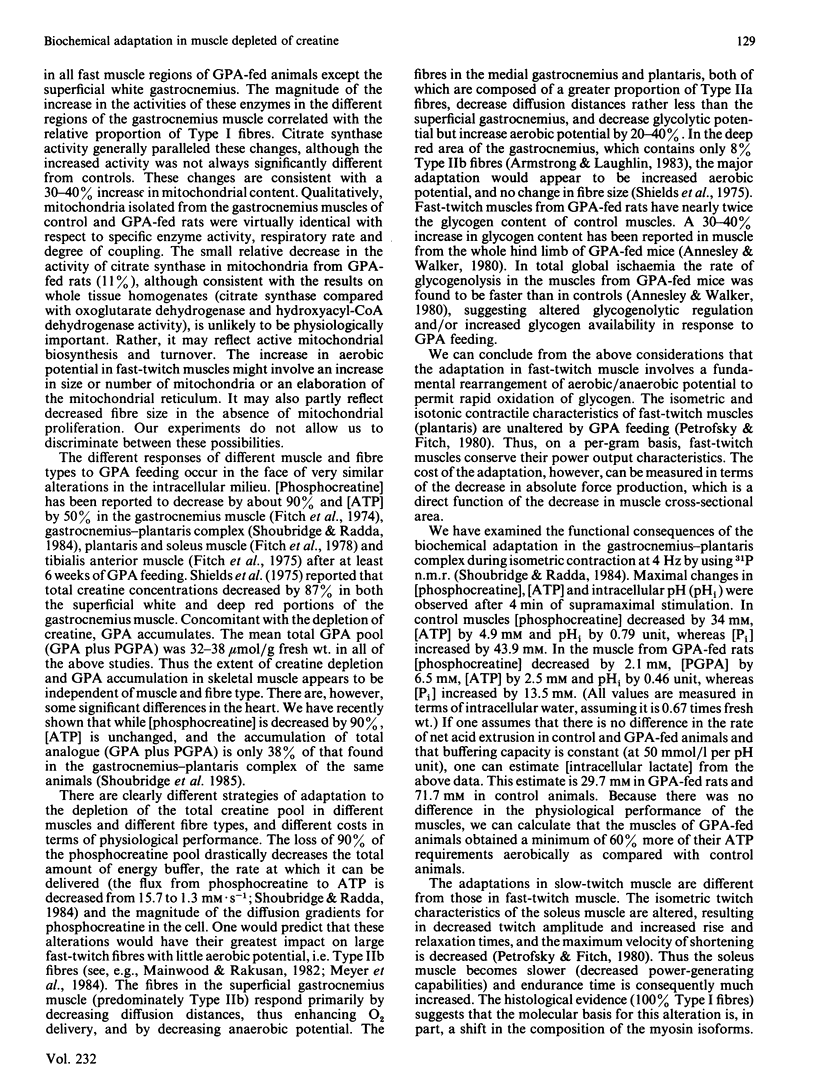
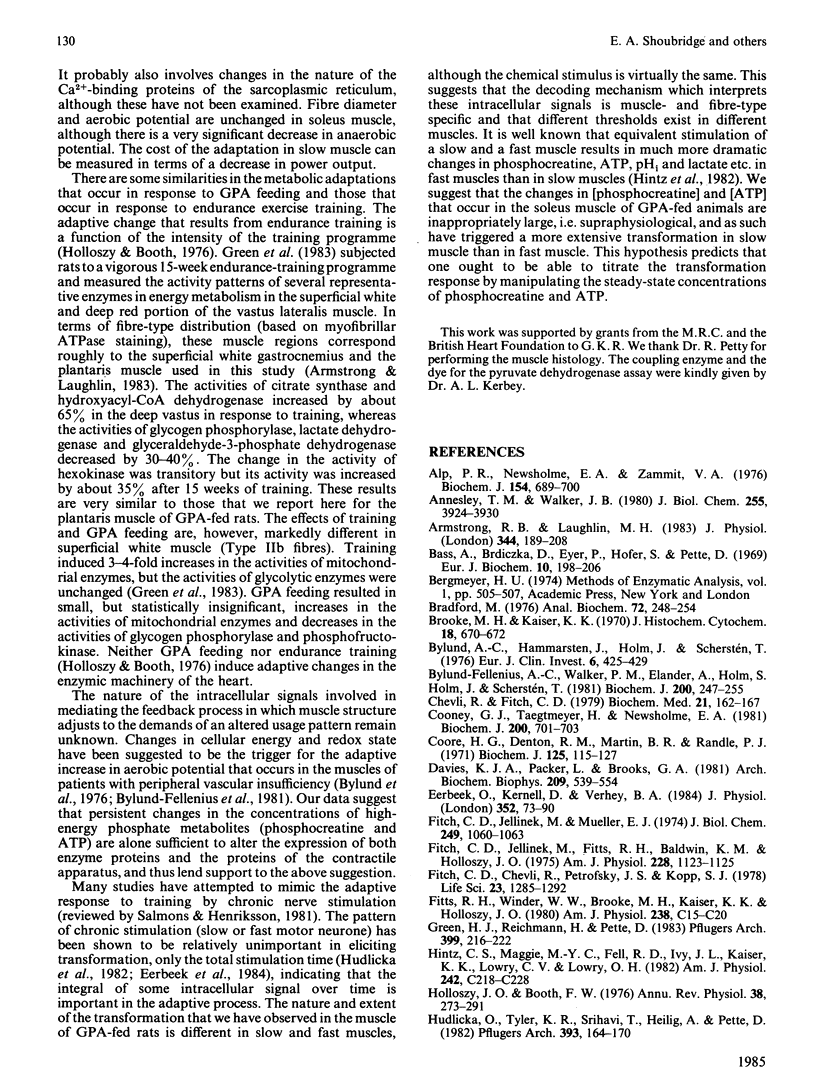
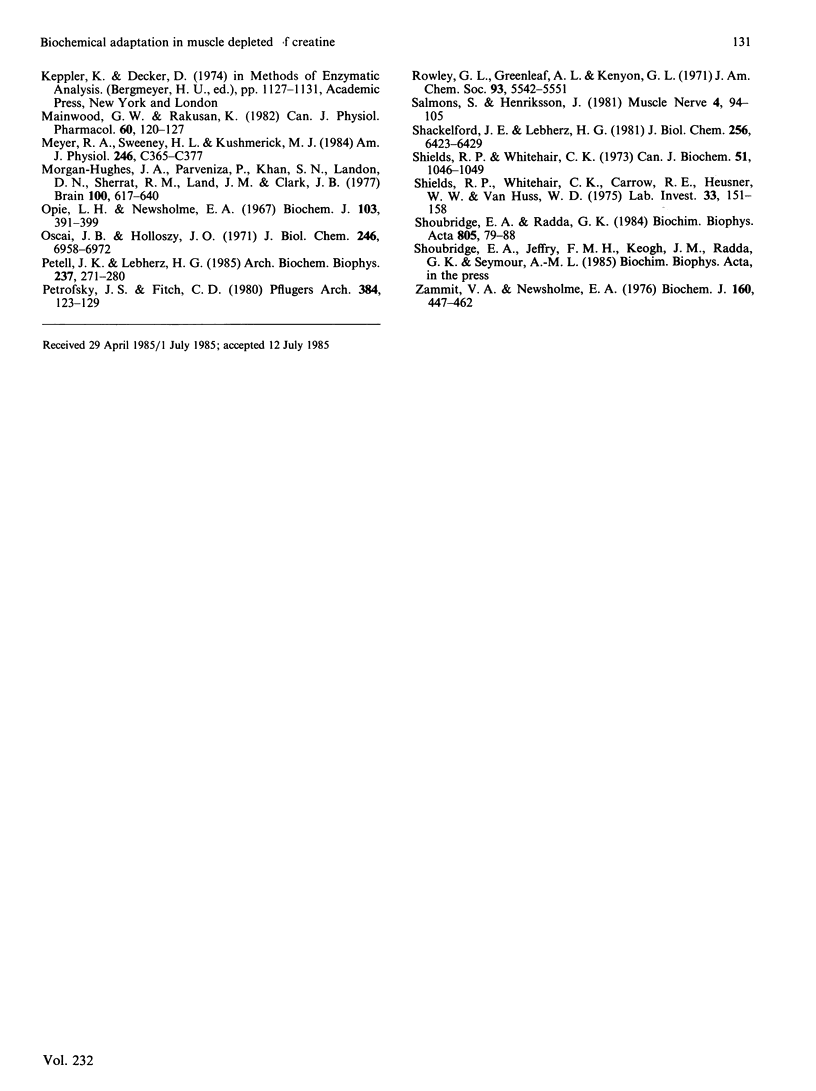
Selected References
These references are in PubMed. This may not be the complete list of references from this article.
- Alp P. R., Newsholme E. A., Zammit V. A. Activities of citrate synthase and NAD+-linked and NADP+-linked isocitrate dehydrogenase in muscle from vertebrates and invertebrates. Biochem J. 1976 Mar 15;154(3):689–700. doi: 10.1042/bj1540689. [DOI] [PMC free article] [PubMed] [Google Scholar]
- Annesley T. M., Walker J. B. Energy metabolism of skeletal muscle containing cyclocreatine phosphate. Delay in onset of rigor mortis and decreased glycogenolysis in response to ischemia or epinephrine. J Biol Chem. 1980 May 10;255(9):3924–3930. [PubMed] [Google Scholar]
- Armstrong R. B., Laughlin M. H. Blood flows within and among rat muscles as a function of time during high speed treadmill exercise. J Physiol. 1983 Nov;344:189–208. doi: 10.1113/jphysiol.1983.sp014933. [DOI] [PMC free article] [PubMed] [Google Scholar]
- Bass A., Brdiczka D., Eyer P., Hofer S., Pette D. Metabolic differentiation of distinct muscle types at the level of enzymatic organization. Eur J Biochem. 1969 Sep;10(2):198–206. doi: 10.1111/j.1432-1033.1969.tb00674.x. [DOI] [PubMed] [Google Scholar]
- Bradford M. M. A rapid and sensitive method for the quantitation of microgram quantities of protein utilizing the principle of protein-dye binding. Anal Biochem. 1976 May 7;72:248–254. doi: 10.1016/0003-2697(76)90527-3. [DOI] [PubMed] [Google Scholar]
- Brooke M. H., Kaiser K. K. Three "myosin adenosine triphosphatase" systems: the nature of their pH lability and sulfhydryl dependence. J Histochem Cytochem. 1970 Sep;18(9):670–672. doi: 10.1177/18.9.670. [DOI] [PubMed] [Google Scholar]
- Bylund-Fellenius A. C., Walker P. M., Elander A., Holm S., Holm J., Scherstén T. Energy metabolism in relation to oxygen partial pressure in human skeletal muscle during exercise. Biochem J. 1981 Nov 15;200(2):247–255. doi: 10.1042/bj2000247. [DOI] [PMC free article] [PubMed] [Google Scholar]
- Bylund A. C., Hammarsten J., Holm J., Scherstén T. Enzyme activities in skeletal muscles from patients with peripheral arterial insufficiency. Eur J Clin Invest. 1976 Nov 30;6(6):425–429. doi: 10.1111/j.1365-2362.1976.tb00538.x. [DOI] [PubMed] [Google Scholar]
- Chevli R., Fitch C. D. beta-Guanidinopropionate and phosphorylated beta-guanidinopropionate as substrates for creatine kinase. Biochem Med. 1979 Apr;21(2):162–167. doi: 10.1016/0006-2944(79)90068-1. [DOI] [PubMed] [Google Scholar]
- Cooney G. J., Taegtmeyer H., Newsholme E. A. Tricarboxylic acid cycle flux and enzyme activities in the isolated working rat heart. Biochem J. 1981 Dec 15;200(3):701–703. doi: 10.1042/bj2000701. [DOI] [PMC free article] [PubMed] [Google Scholar]
- Coore H. G., Denton R. M., Martin B. R., Randle P. J. Regulation of adipose tissue pyruvate dehydrogenase by insulin and other hormones. Biochem J. 1971 Nov;125(1):115–127. doi: 10.1042/bj1250115. [DOI] [PMC free article] [PubMed] [Google Scholar]
- Davies K. J., Packer L., Brooks G. A. Biochemical adaptation of mitochondria, muscle, and whole-animal respiration to endurance training. Arch Biochem Biophys. 1981 Jul;209(2):539–554. doi: 10.1016/0003-9861(81)90312-x. [DOI] [PubMed] [Google Scholar]
- Eerbeek O., Kernell D., Verhey B. A. Effects of fast and slow patterns of tonic long-term stimulation on contractile properties of fast muscle in the cat. J Physiol. 1984 Jul;352:73–90. doi: 10.1113/jphysiol.1984.sp015278. [DOI] [PMC free article] [PubMed] [Google Scholar]
- Fitch C. D., Chevli R., Petrofsky J. S., Kopp S. J. Sustained isometric contraction of skeletal muscle depleted of phosphocreatine. Life Sci. 1978 Sep 25;23(12):1285–1291. doi: 10.1016/0024-3205(78)90507-6. [DOI] [PubMed] [Google Scholar]
- Fitch C. D., Jellinek M., Fitts R. H., Baldwin K. M., Holloszy J. O. Phosphorylated beta-guanidinopropionate as a substitute for phosphocreatine in rat muscle. Am J Physiol. 1975 Apr;228(4):1123–1125. doi: 10.1152/ajplegacy.1975.228.4.1123. [DOI] [PubMed] [Google Scholar]
- Fitch C. D., Jellinek M., Mueller E. J. Experimental depletion of creatine and phosphocreatine from skeletal muscle. J Biol Chem. 1974 Feb 25;249(4):1060–1063. [PubMed] [Google Scholar]
- Fitts R. H., Winder W. W., Brooke M. H., Kaiser K. K., Holloszy J. O. Contractile, biochemical, and histochemical properties of thyrotoxic rat soleus muscle. Am J Physiol. 1980 Jan;238(1):C14–C20. doi: 10.1152/ajpcell.1980.238.1.C15. [DOI] [PubMed] [Google Scholar]
- Green H. J., Reichmann H., Pette D. Fibre type specific transformations in the enzyme activity pattern of rat vastus lateralis muscle by prolonged endurance training. Pflugers Arch. 1983 Nov;399(3):216–222. doi: 10.1007/BF00656718. [DOI] [PubMed] [Google Scholar]
- Hintz C. S., Chi M. M., Fell R. D., Ivy J. L., Kaiser K. K., Lowry C. V., Lowry O. H. Metabolite changes in individual rat muscle fibers during stimulation. Am J Physiol. 1982 Mar;242(3):C218–C228. doi: 10.1152/ajpcell.1982.242.3.C218. [DOI] [PubMed] [Google Scholar]
- Holloszy J. O., Booth F. W. Biochemical adaptations to endurance exercise in muscle. Annu Rev Physiol. 1976;38:273–291. doi: 10.1146/annurev.ph.38.030176.001421. [DOI] [PubMed] [Google Scholar]
- Hudlická O., Tyler K. R., Srihari T., Heilig A., Pette D. The effect of different patterns of long-term stimulation on contractile properties and myosin light chains in rabbit fast muscles. Pflugers Arch. 1982 Apr;393(2):164–170. doi: 10.1007/BF00582940. [DOI] [PubMed] [Google Scholar]
- Mainwood G. W., Alward M., Eiselt B. Contractile characteristics of creatine-depleted rat diaphragm. Can J Physiol Pharmacol. 1982 Feb;60(2):120–127. doi: 10.1139/y82-020. [DOI] [PubMed] [Google Scholar]
- Meyer R. A., Sweeney H. L., Kushmerick M. J. A simple analysis of the "phosphocreatine shuttle". Am J Physiol. 1984 May;246(5 Pt 1):C365–C377. doi: 10.1152/ajpcell.1984.246.5.C365. [DOI] [PubMed] [Google Scholar]
- Morgan-Hughes J. A., Darveniza P., Kahn S. N., Landon D. N., Sherratt R. M., Land J. M., Clark J. B. A mitochondrial myopathy characterized by a deficiency in reducible cytochrome b. Brain. 1977 Dec;100(4):617–640. doi: 10.1093/brain/100.4.617. [DOI] [PubMed] [Google Scholar]
- Opie L. H., Newsholme E. A. The activities of fructose 1,6-diphosphatase, phosphofructokinase and phosphoenolpyruvate carboxykinase in white muscle and red muscle. Biochem J. 1967 May;103(2):391–399. doi: 10.1042/bj1030391. [DOI] [PMC free article] [PubMed] [Google Scholar]
- Oscai L. B., Holloszy J. O. Biochemical adaptations in muscle. II. Response of mitochondrial adenosine triphosphatase, creatine phosphokinase, and adenylate kinase activities in skeletal muscle to exercise. J Biol Chem. 1971 Nov 25;246(22):6968–6972. [PubMed] [Google Scholar]
- Petell J. K., Lebherz H. G. Content and synthesis of glycolytic enzymes and creatine kinase in skeletal muscles and normal and dystrophic chickens. Arch Biochem Biophys. 1985 Feb 15;237(1):271–280. doi: 10.1016/0003-9861(85)90278-4. [DOI] [PubMed] [Google Scholar]
- Petrofsky J. S., Fitch C. D. Contractile characteristics of skeletal muscles depleted of phosphocreatine. Pflugers Arch. 1980 Mar;384(2):123–129. doi: 10.1007/BF00584427. [DOI] [PubMed] [Google Scholar]
- Rowley G. L., Greenleaf A. L., Kenyon G. L. On the specificity of creatine kinase. New glycocyamines and glycocyamine analogs related to creatine. J Am Chem Soc. 1971 Oct 20;93(12):5542–5551. doi: 10.1021/ja00750a038. [DOI] [PubMed] [Google Scholar]
- Salmons S., Henriksson J. The adaptive response of skeletal muscle to increased use. Muscle Nerve. 1981 Mar-Apr;4(2):94–105. doi: 10.1002/mus.880040204. [DOI] [PubMed] [Google Scholar]
- Shackelford J. E., Lebherz H. G. Effect of denervation on the levels and rates of synthesis of specific enzymes in "fast-twitch" (breast) muscle fibers of the chicken. J Biol Chem. 1981 Jun 25;256(12):6423–6429. [PubMed] [Google Scholar]
- Shields R. P., Whitehair C. K., Carrow R. E., Heusner W. W., Van Huss W. D. Skeletal muscle function and structure after depletion of creatine. Lab Invest. 1975 Aug;33(2):151–158. [PubMed] [Google Scholar]
- Shields R. P., Whitehair C. K. Muscle creatine: in vivo depletion by feeding beta-guanidinopropionic acid. Can J Biochem. 1973 Jul;51(7):1046–1049. doi: 10.1139/o73-136. [DOI] [PubMed] [Google Scholar]
- Shoubridge E. A., Radda G. K. A 31P-nuclear magnetic resonance study of skeletal muscle metabolism in rats depleted of creatine with the analogue beta-guanidinopropionic acid. Biochim Biophys Acta. 1984 Sep 14;805(1):79–88. doi: 10.1016/0167-4889(84)90039-9. [DOI] [PubMed] [Google Scholar]
- Zammit V. A., Newsholme E. A. The maximum activities of hexokinase, phosphorylase, phosphofructokinase, glycerol phosphate dehydrogenases, lactate dehydrogenase, octopine dehydrogenase, phosphoenolpyruvate carboxykinase, nucleoside diphosphatekinase, glutamate-oxaloacetate transaminase and arginine kinase in relation to carbohydrate utilization in muscles from marine invertebrates. Biochem J. 1976 Dec 15;160(3):447–462. doi: 10.1042/bj1600447. [DOI] [PMC free article] [PubMed] [Google Scholar]