Abstract
The growth of one smooth and three individual striated muscles was studied from birth to old age (105 weeks), and where possible during the later stages of foetal life also. Developmental changes in protein turnover (measured in vivo) were related to the changing patterns of growth within each muscle, and the body as a whole. Developmental growth (i.e. protein accumulation) in all muscles involved an increasing proportion of protein per unit wet weight, as well as cellular hypertrophy. The contribution of the heart towards whole-body protein and nucleic acid contents progressively decreased from 18 days of gestation to senility. In contrast, post-natal changes in both slow-twitch (soleus) and fast-twitch (tibialis anterior) skeletal muscles remained reasonably constant with respect to whole-body values. Such age-related growth in all four muscle types was accompanied by a progressive decline in both the fractional rates of protein synthesis and breakdown, the changes in synthesis being more pronounced. Age for age, the fractional rates of synthesis were highest in the oesophageal smooth muscle, similar in both cardiac and the slow-twitch muscles, and lowest in the fast-twitch tibialis muscle. Despite these differences, the developmental fall in synthetic rates was remarkably similar in all four muscles, e.g. the rates at 105 weeks were 30-35% of their values at weaning. Such developmental changes in synthesis were largely related to diminishing ribosomal capacities within each muscle. When measured under near-steady-state conditions (i.e. 105 weeks of age), the half-lives of mixed muscle proteins were 5.1, 10.4, 12.1 and 18.3 days for the smooth, cardiac, soleus and tibialis muscles respectively. Old-age atrophy was evident in the senile animals, this being more marked in each of the four muscle types than in the animal as a whole. In each muscle of the senile rats the protein content and composition per unit wet weight, and both the fractional and total rates of synthesis, were significantly lower than in the muscles of younger, mature, animals (i.e. 44 weeks). In the soleus the decreased synthesis rate appeared to be related to a further fall in the ribosomal capacity. In contrast, the changes in synthesis in the three remaining muscles correlated with significant decreases in the synthetic rate per ribosome.(ABSTRACT TRUNCATED AT 400 WORDS)
Full text
PDF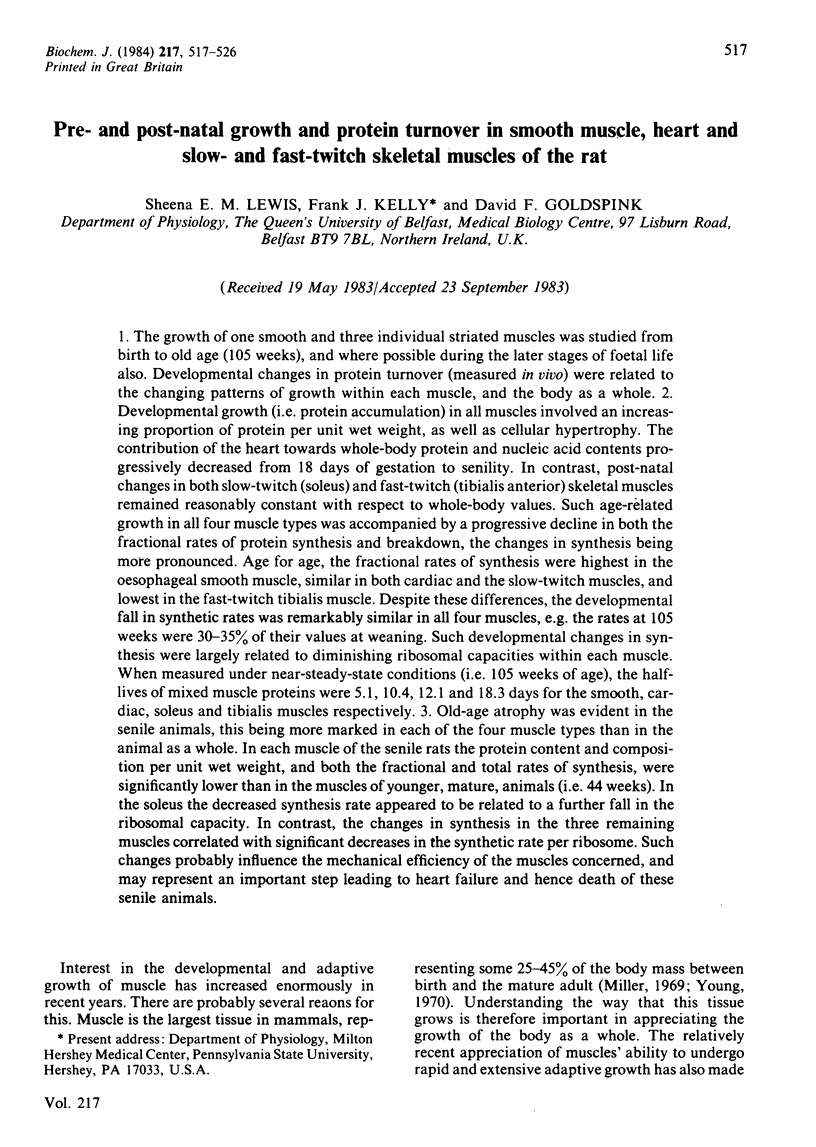
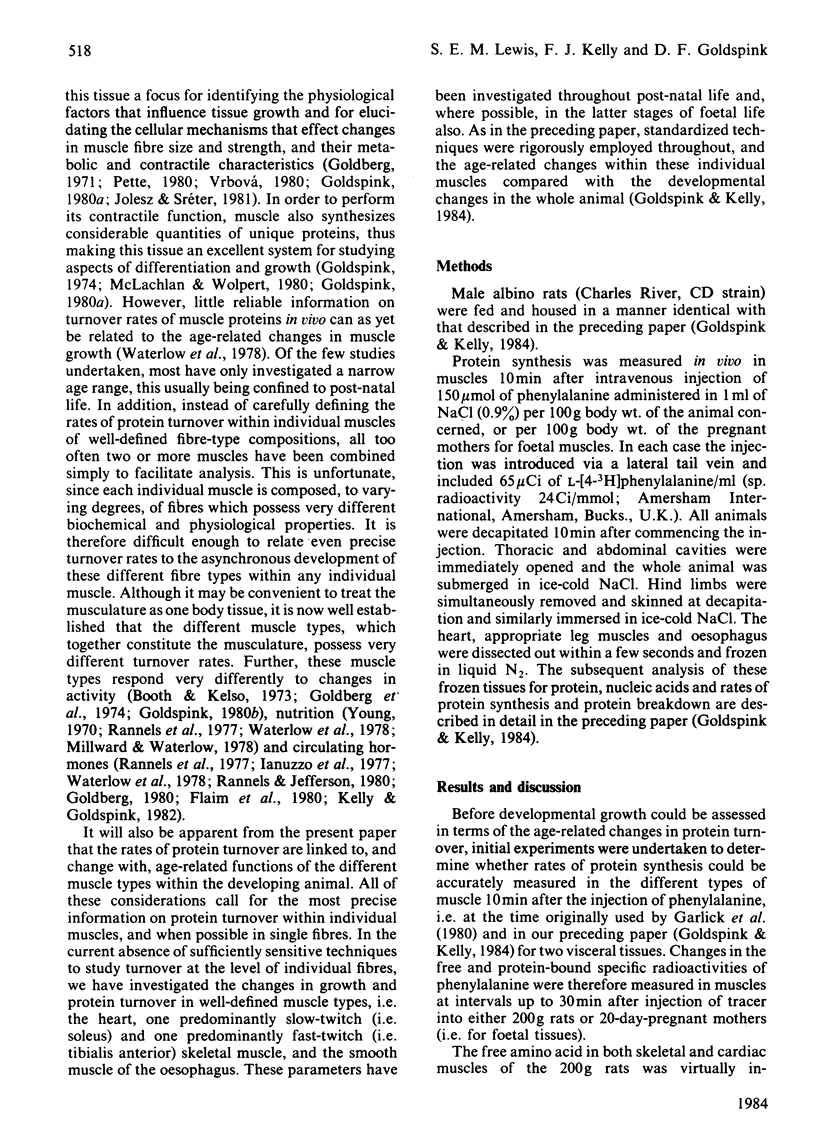
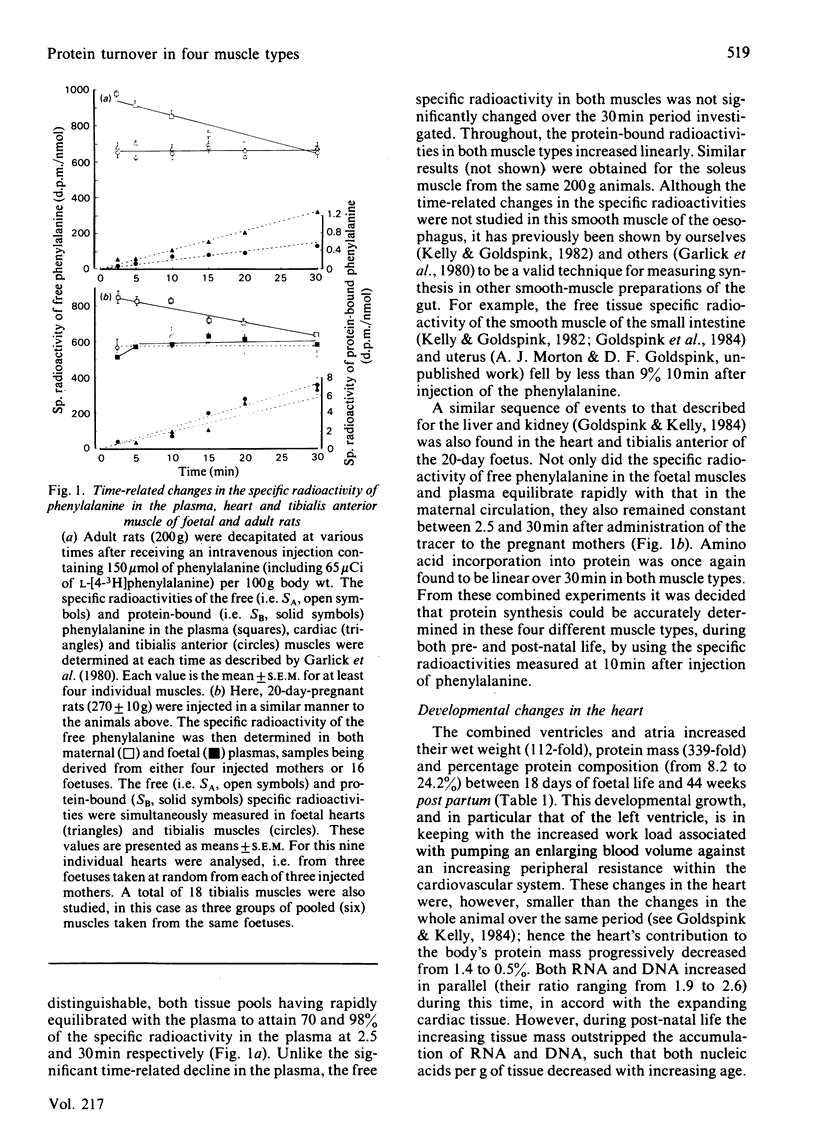
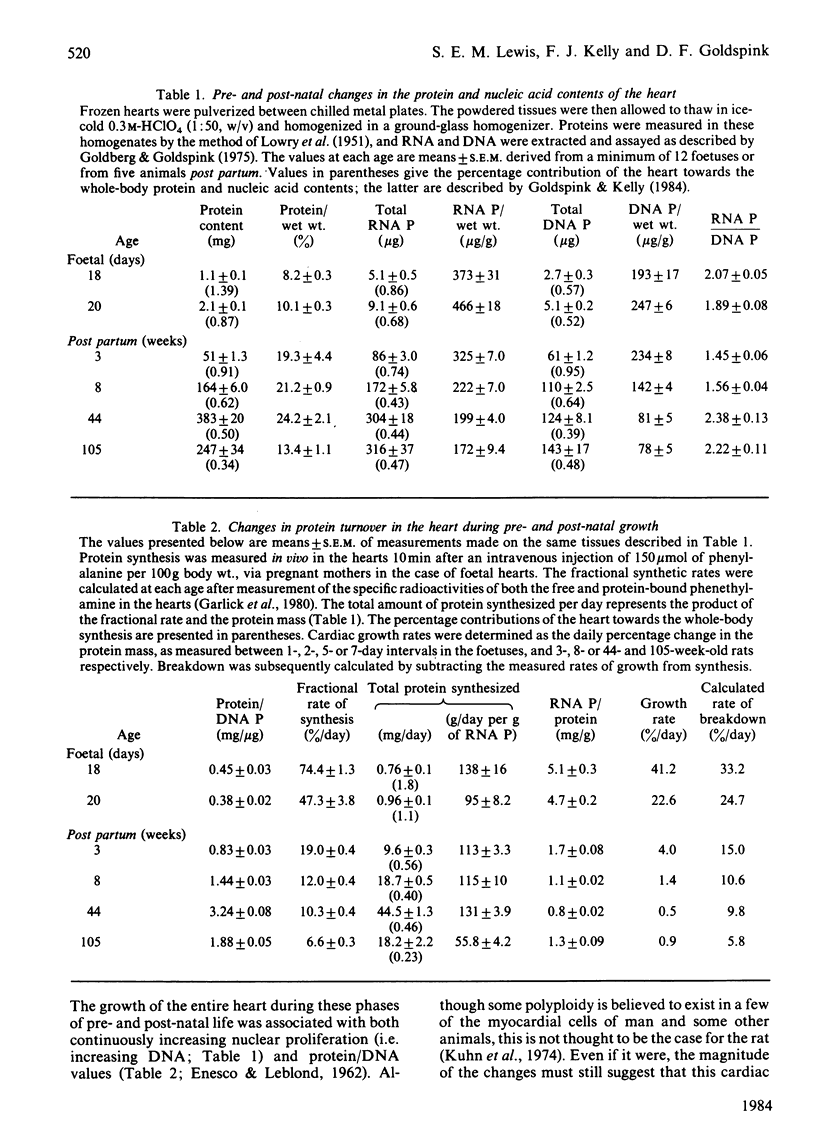
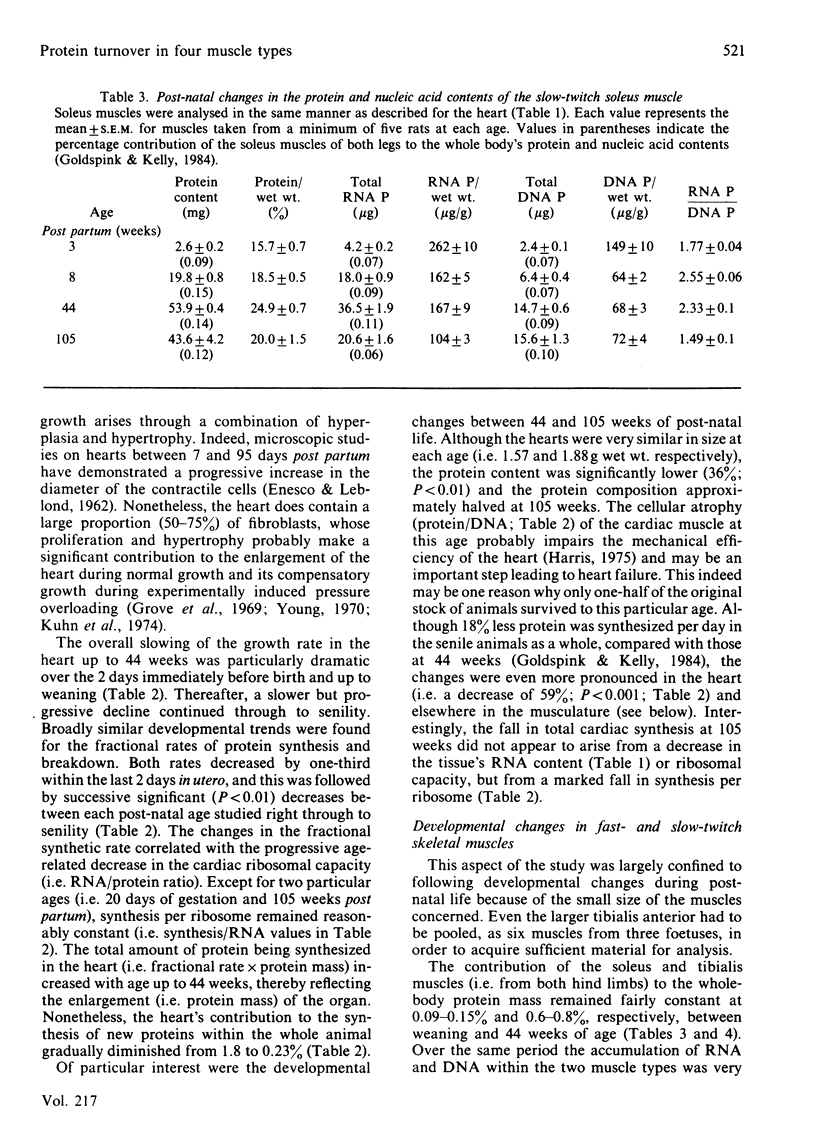
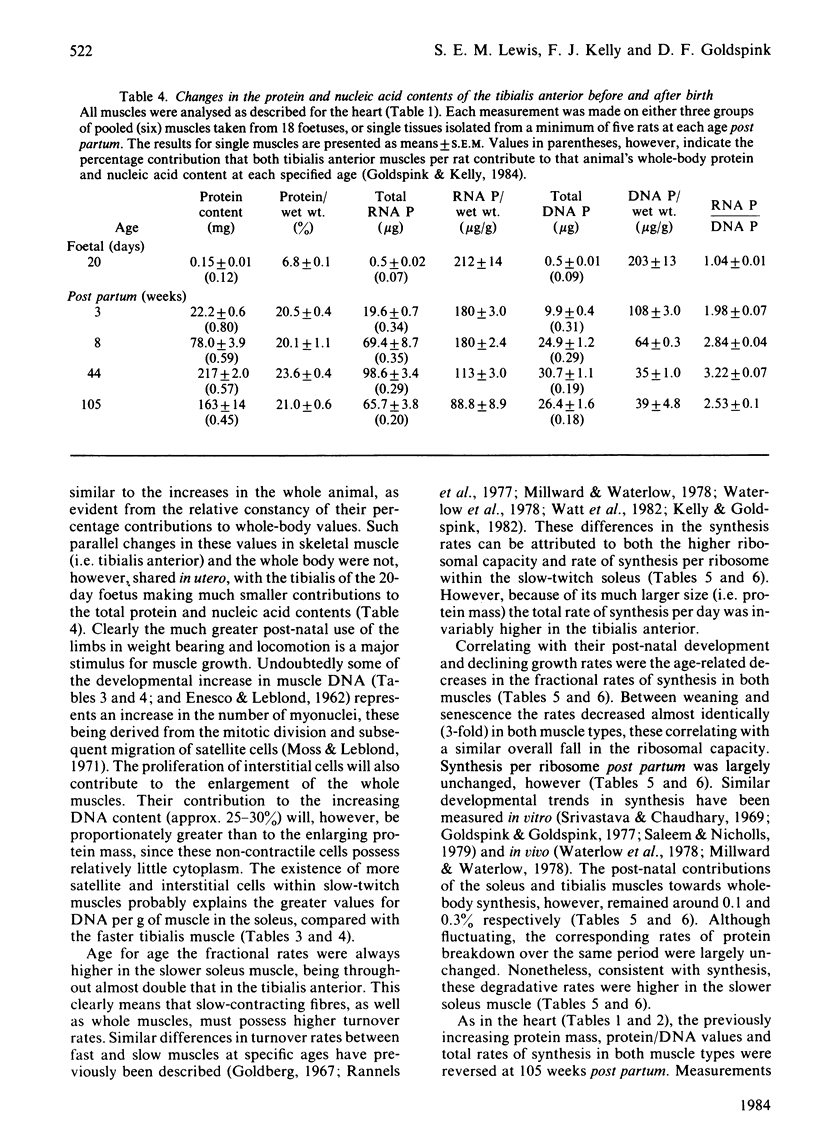
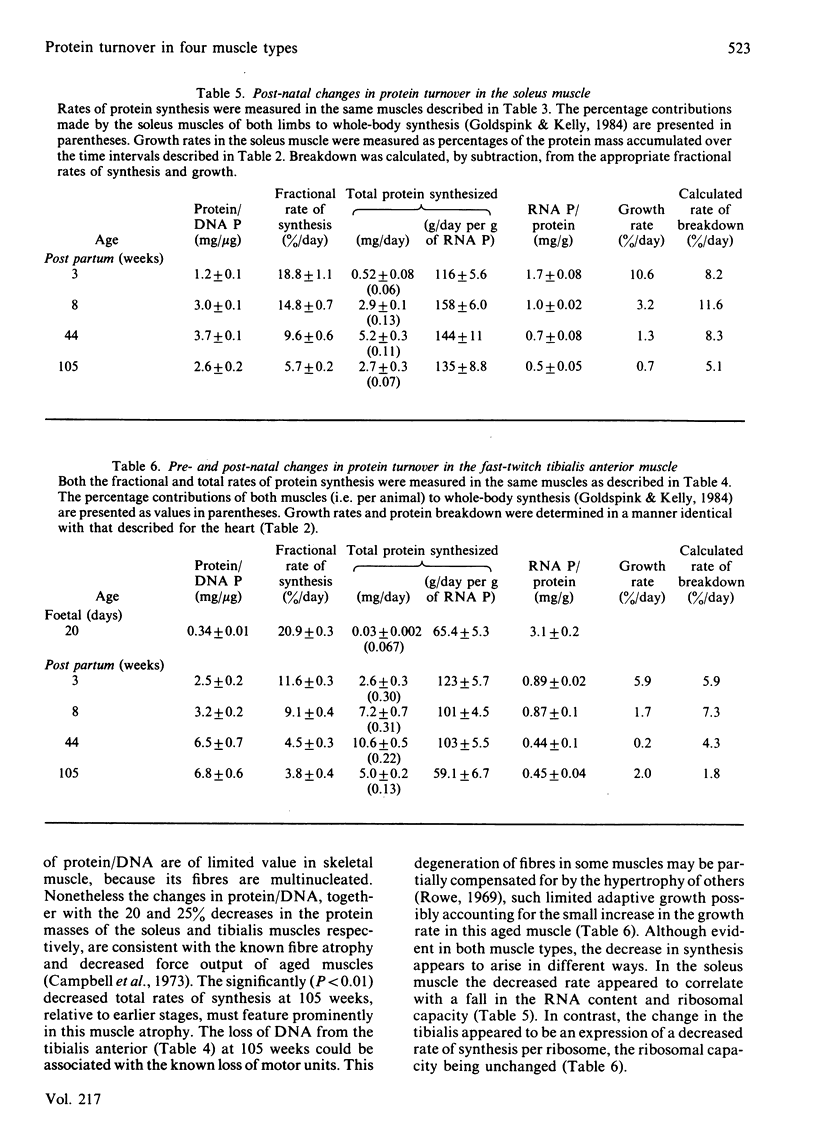
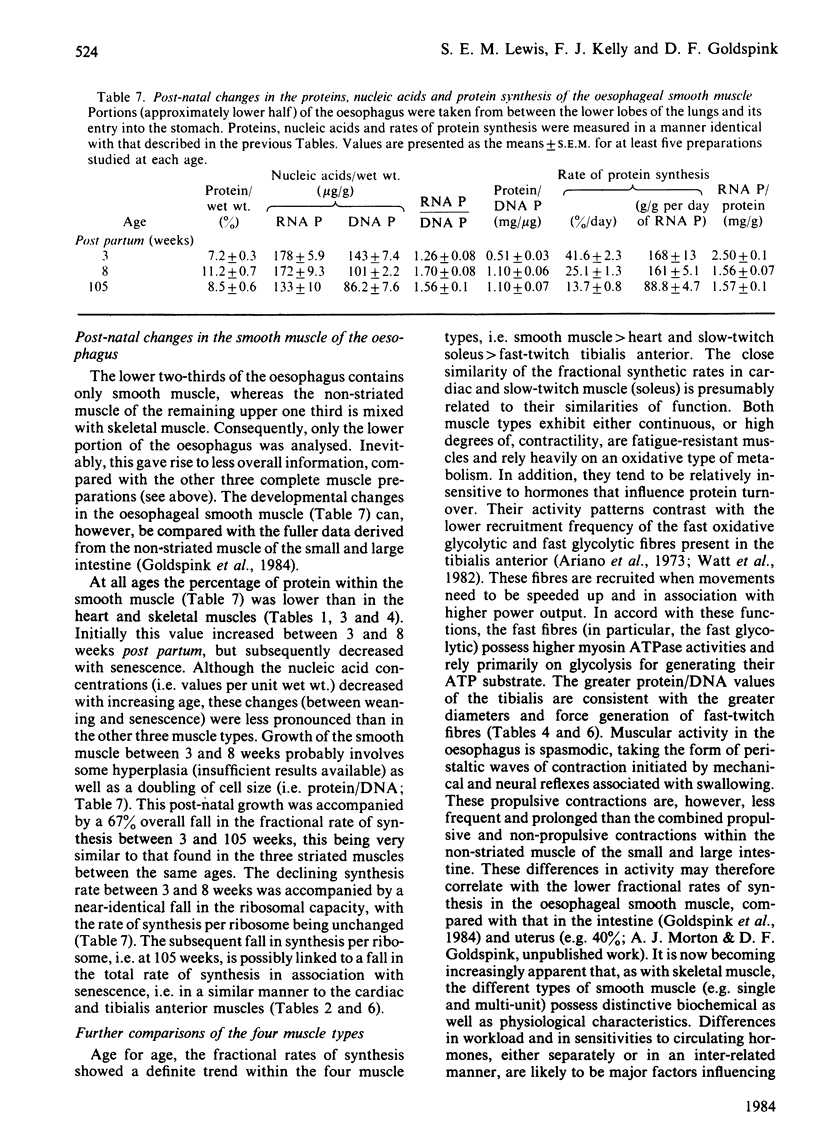
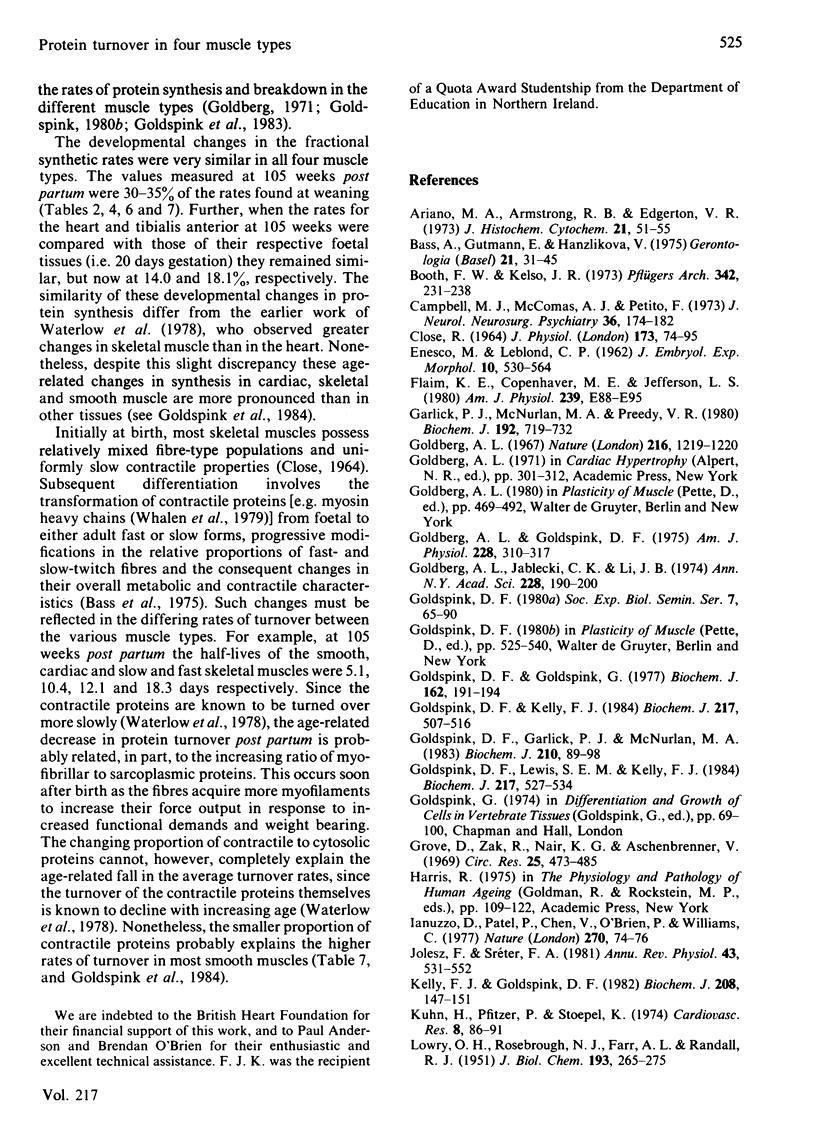
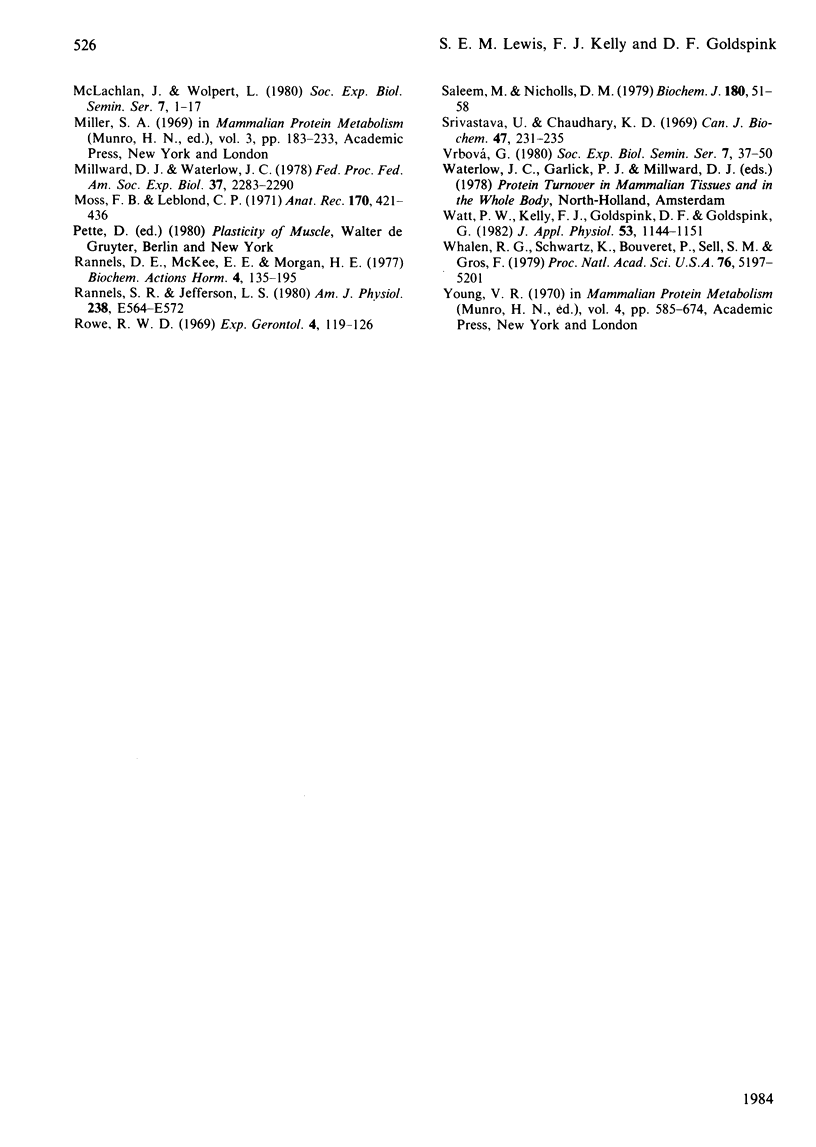
Selected References
These references are in PubMed. This may not be the complete list of references from this article.
- Ariano M. A., Armstrong R. B., Edgerton V. R. Hindlimb muscle fiber populations of five mammals. J Histochem Cytochem. 1973 Jan;21(1):51–55. doi: 10.1177/21.1.51. [DOI] [PubMed] [Google Scholar]
- Bass A., Gutmann E., Hanzlíková V. Biochemical and histochemical changes in energy supply enzyme pattern of muscles of the rat during old age. Gerontologia. 1975;21(1):31–45. doi: 10.1159/000212028. [DOI] [PubMed] [Google Scholar]
- Booth F. W., Kelso J. R. Effect of hind-limb immobilization on contractile and histochemical properties of skeletal muscle. Pflugers Arch. 1973 Aug 27;342(3):231–238. doi: 10.1007/BF00591371. [DOI] [PubMed] [Google Scholar]
- CLOSE R. DYNAMIC PROPERTIES OF FAST AND SLOW SKELETAL MUSCLES OF THE RAT DURING DEVELOPMENT. J Physiol. 1964 Sep;173:74–95. doi: 10.1113/jphysiol.1964.sp007444. [DOI] [PMC free article] [PubMed] [Google Scholar]
- Campbell M. J., McComas A. J., Petito F. Physiological changes in ageing muscles. J Neurol Neurosurg Psychiatry. 1973 Apr;36(2):174–182. doi: 10.1136/jnnp.36.2.174. [DOI] [PMC free article] [PubMed] [Google Scholar]
- Flaim K. E., Copenhaver M. E., Jefferson L. S. Effects of diabetes on protein synthesis in fast- and slow-twitch rat skeletal muscle. Am J Physiol. 1980 Jul;239(1):E88–E95. doi: 10.1152/ajpendo.1980.239.1.E88. [DOI] [PubMed] [Google Scholar]
- Garlick P. J., McNurlan M. A., Preedy V. R. A rapid and convenient technique for measuring the rate of protein synthesis in tissues by injection of [3H]phenylalanine. Biochem J. 1980 Nov 15;192(2):719–723. doi: 10.1042/bj1920719. [DOI] [PMC free article] [PubMed] [Google Scholar]
- Goldberg A. L., Goldspink D. F. Influence of food deprivation and adrenal steroids on DNA synthesis in various mammalian tissues. Am J Physiol. 1975 Jan;228(1):310–317. doi: 10.1152/ajplegacy.1975.228.1.310. [DOI] [PubMed] [Google Scholar]
- Goldberg A. L., Jablecki C., Li J. B. Trophic functions of the neuron. 3. Mechanisms of neurotrophic interactions. Effects of use and disuse on amino acid transport and protein turnover in muscle. Ann N Y Acad Sci. 1974 Mar 22;228(0):190–201. doi: 10.1111/j.1749-6632.1974.tb20510.x. [DOI] [PubMed] [Google Scholar]
- Goldberg A. L. Protein synthesis in tonic and phasic skeletal muscles. Nature. 1967 Dec 23;216(5121):1219–1220. doi: 10.1038/2161219a0. [DOI] [PubMed] [Google Scholar]
- Goldspink D. F., Garlick P. J., McNurlan M. A. Protein turnover measured in vivo and in vitro in muscles undergoing compensatory growth and subsequent denervation atrophy. Biochem J. 1983 Jan 15;210(1):89–98. doi: 10.1042/bj2100089. [DOI] [PMC free article] [PubMed] [Google Scholar]
- Goldspink D. F., Goldspink G. Age-related changes in protein turnover and ribonucleic acid of the diaphragm muscle of normal and dystrophic hamsters. Biochem J. 1977 Jan 15;162(1):191–194. doi: 10.1042/bj1620191. [DOI] [PMC free article] [PubMed] [Google Scholar]
- Goldspink D. F., Kelly F. J. Protein turnover and growth in the whole body, liver and kidney of the rat from the foetus to senility. Biochem J. 1984 Jan 15;217(2):507–516. doi: 10.1042/bj2170507. [DOI] [PMC free article] [PubMed] [Google Scholar]
- Goldspink D. F., Lewis S. E., Kelly F. J. Protein synthesis during the developmental growth of the small and large intestine of the rat. Biochem J. 1984 Jan 15;217(2):527–534. doi: 10.1042/bj2170527. [DOI] [PMC free article] [PubMed] [Google Scholar]
- Grove D., Zak R., Nair K. G., Aschenbrenner V. Biochemical correlates of cardiac hypertrophy. IV. Observations on the cellular organization of growth during myocardial hypertrophy in the rat. Circ Res. 1969 Oct;25(4):473–485. doi: 10.1161/01.res.25.4.473. [DOI] [PubMed] [Google Scholar]
- Ianuzzo D., Patel P., Chen V., O'Brien P., Williams C. Thyroidal trophic influence on skeletal muscle myosin. Nature. 1977 Nov 3;270(5632):74–76. doi: 10.1038/270074a0. [DOI] [PubMed] [Google Scholar]
- Jolesz F., Sreter F. A. Development, innervation, and activity-pattern induced changes in skeletal muscle. Annu Rev Physiol. 1981;43:531–552. doi: 10.1146/annurev.ph.43.030181.002531. [DOI] [PubMed] [Google Scholar]
- Kelly F. J., Goldspink D. F. The differing responses of four muscle types to dexamethasone treatment in the rat. Biochem J. 1982 Oct 15;208(1):147–151. doi: 10.1042/bj2080147. [DOI] [PMC free article] [PubMed] [Google Scholar]
- Kuhn H., Pfitzer P., Stoepel K. DNA content and DNA synthesis in the myocardium of rats after induced renal hypertension. Cardiovasc Res. 1974 Jan;8(1):86–91. doi: 10.1093/cvr/8.1.86. [DOI] [PubMed] [Google Scholar]
- LOWRY O. H., ROSEBROUGH N. J., FARR A. L., RANDALL R. J. Protein measurement with the Folin phenol reagent. J Biol Chem. 1951 Nov;193(1):265–275. [PubMed] [Google Scholar]
- Millward D. J., Waterlow J. C. Effect of nutrition on protein turnover in skeletal muscle. Fed Proc. 1978 Jul;37(9):2283–2290. [PubMed] [Google Scholar]
- Moss F. P., Leblond C. P. Satellite cells as the source of nuclei in muscles of growing rats. Anat Rec. 1971 Aug;170(4):421–435. doi: 10.1002/ar.1091700405. [DOI] [PubMed] [Google Scholar]
- Rannels S. R., Jefferson L. S. Effects of glucocorticoids on muscle protein turnover in perfused rat hemicorpus. Am J Physiol. 1980 Jun;238(6):E564–E572. doi: 10.1152/ajpendo.1980.238.6.E564. [DOI] [PubMed] [Google Scholar]
- Rowe R. W. The effect of senility on skeletal muscles in the mouse. Exp Gerontol. 1969 Jul;4(2):119–126. doi: 10.1016/0531-5565(69)90034-5. [DOI] [PubMed] [Google Scholar]
- Saleem M., Nicholls D. M. Protein synthesis in muscles from normal and dystrophic hamsters. Biochem J. 1979 Apr 15;180(1):51–58. doi: 10.1042/bj1800051. [DOI] [PMC free article] [PubMed] [Google Scholar]
- Srivastava U., Chaudhary K. D. Effect of age on protein and ribonucleic acid metabolism in mouse skeletal muscle. Can J Biochem. 1969 Mar;47(3):231–235. doi: 10.1139/o69-037. [DOI] [PubMed] [Google Scholar]
- Watt P. W., Kelly F. J., Goldspink D. F., Goldspink G. Exercise-induced morphological and biochemical changes in skeletal muscles of the rat. J Appl Physiol Respir Environ Exerc Physiol. 1982 Nov;53(5):1144–1151. doi: 10.1152/jappl.1982.53.5.1144. [DOI] [PubMed] [Google Scholar]
- Whalen R. G., Schwartz K., Bouveret P., Sell S. M., Gros F. Contractile protein isozymes in muscle development: identification of an embryonic form of myosin heavy chain. Proc Natl Acad Sci U S A. 1979 Oct;76(10):5197–5201. doi: 10.1073/pnas.76.10.5197. [DOI] [PMC free article] [PubMed] [Google Scholar]