Abstract
The absorption coefficient of butyryl-CoA dehydrogenase from Megasphaera elsdenii at 450 nm is determined as 14.4 mM-1 X cm-1 in the CoA-free form and 14.2 mM-1 X cm-1 in the CoA-liganded form (both yellow). The latter value is considerably higher than the earlier published estimate. Phenazine ethosulphate offers great advantages over phenazine methosulphate as a coupling dye in the catalytic assay despite giving lower Vmax. values (506 min-1 as compared with 1250 min-1 under the conditions used). The phenazine ethosulphate assay is used to establish a pH optimum of 8.05 for oxidation of 100 microM-butyryl-CoA. The rates of oxidation of a range of straight-chain, branched-chain and alicyclic acyl thioesters are used to provide the following information. Only straight-chain acyl groups containing 4-6 carbon atoms are easily accommodated by the postulated hydrophobic pocket of the enzyme. C-3-substituted acyl-CoA thioesters are not oxidized at a significant rate, suggesting that the C-3 pro-S-hydrogen atom of straight-chain substrates is partially exposed to the solvent. Acyl-CoA thioesters with substitutions at C-2 are oxidized, though at a lower rate than their straight-chain counterparts. This implies that the C-2 pro-S-hydrogen atom of straight-chain substrates is partially exposed to the solvent. Saturated alicyclic carboxylic acyl-CoA thioesters with 4-7 carbon atoms in the ring are oxidized, with maximal activity for the cyclohexane derivative. This implies that optimal oxidation requires a true trans orientation of the two departing hydrogen atoms. The strain imposed by bound unsaturated alicyclic acyl thioesters strikingly perturbs the flavin visible-absorption spectrum, with the exception of the cyclohex-2-ene derivative, which forms a complex with similar spectral properties to those of the crotonyl-CoA complex. In the thiol moiety of thioester substrates the amide bond of N-acetylcysteamine is essential for both binding and catalysis. The adenosine structure contributes substantially to strong binding, but is less important in determining the catalytic rate.
Full text
PDF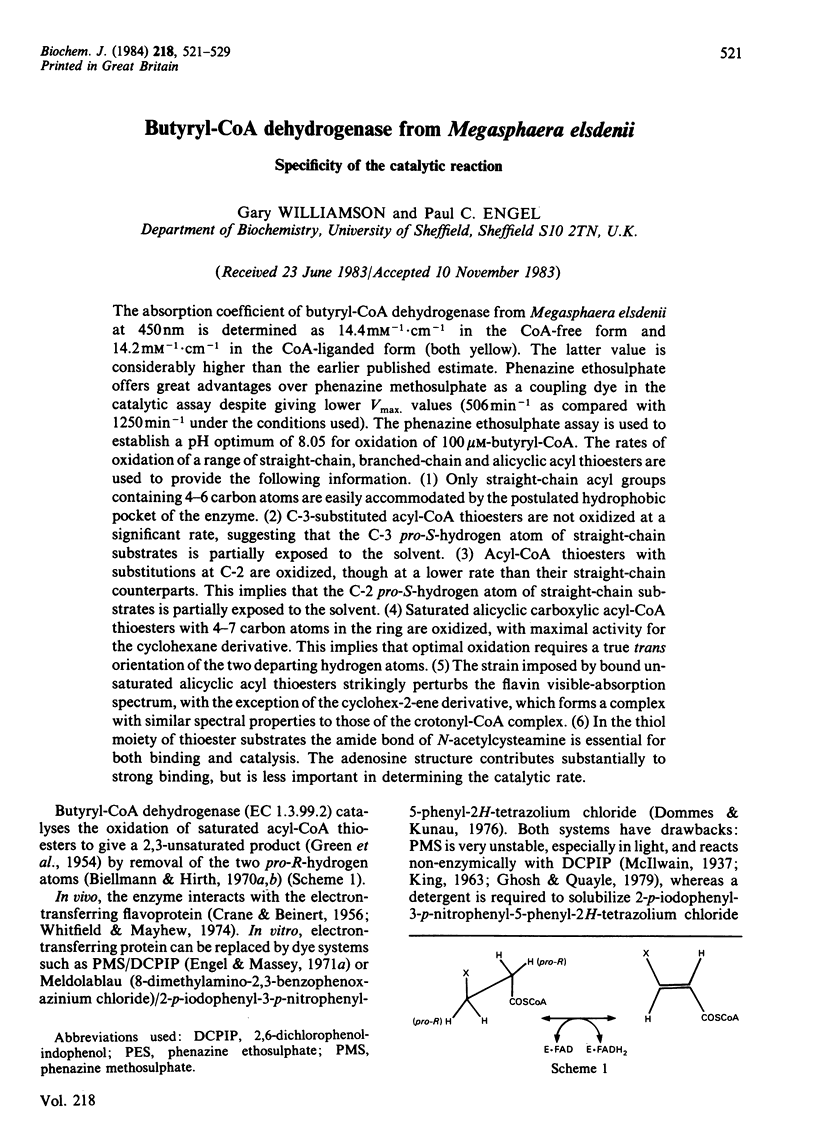
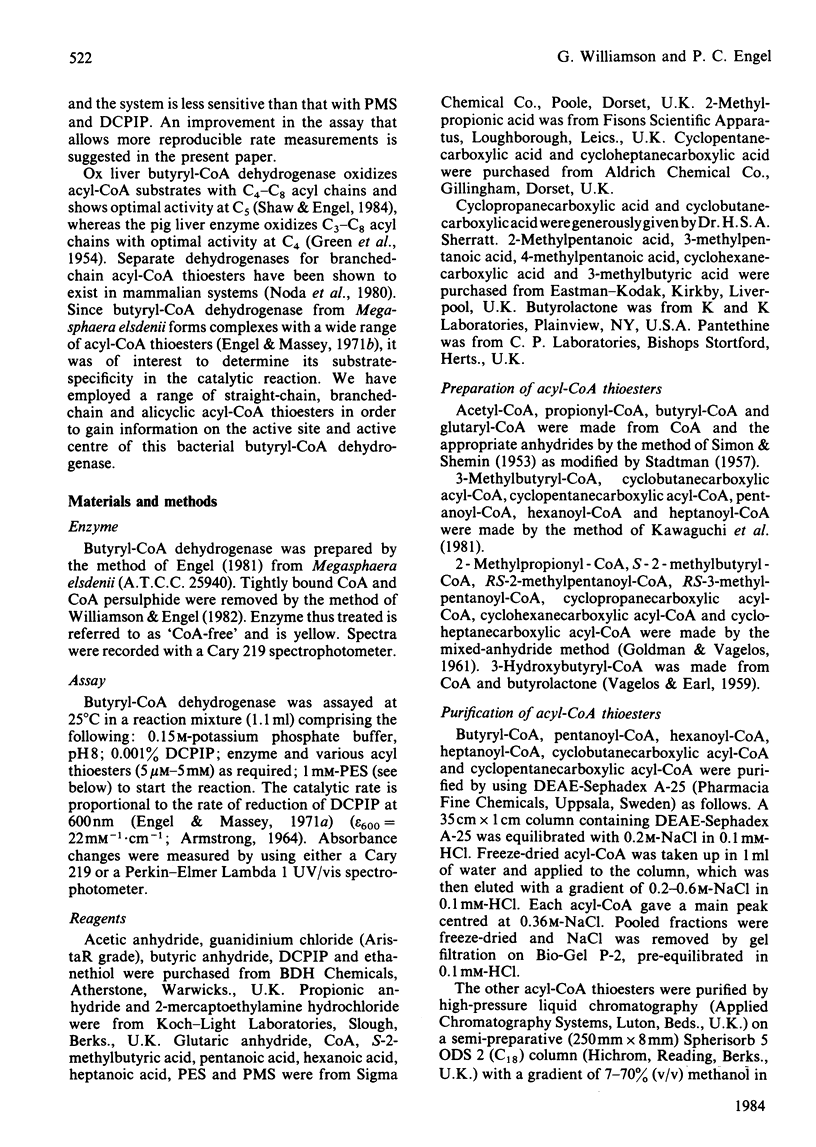
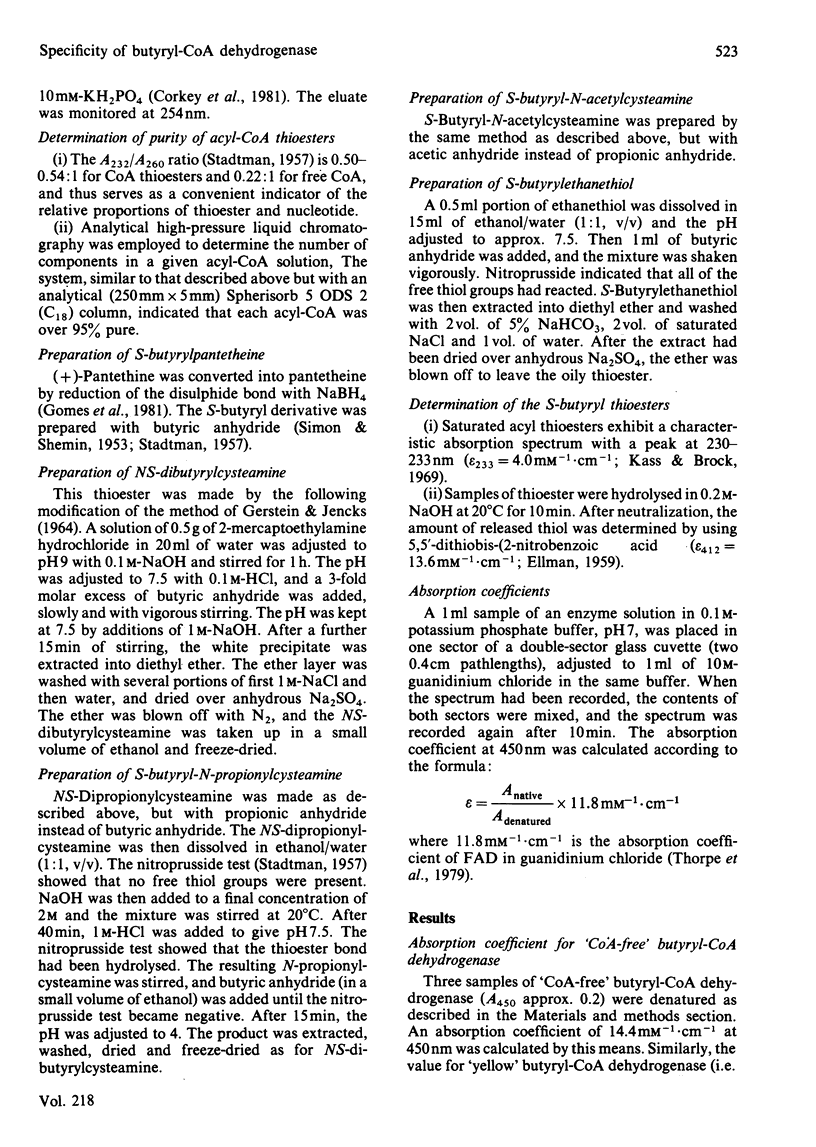
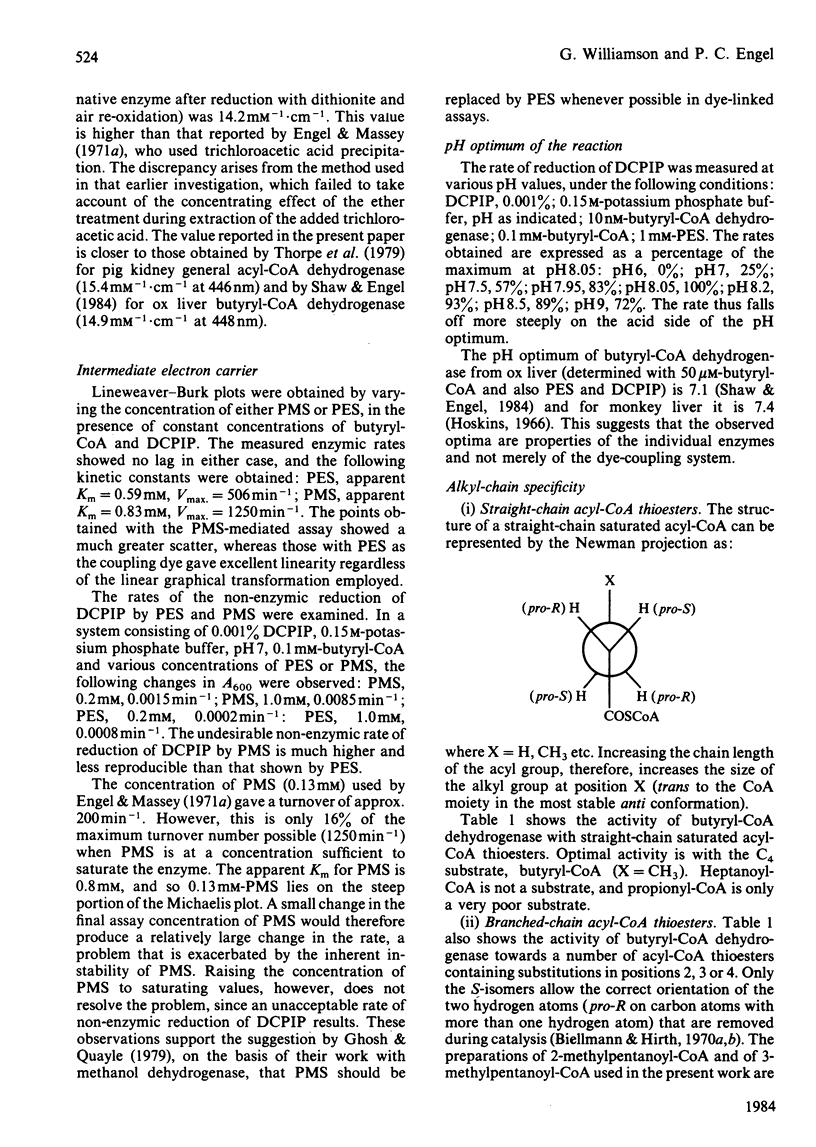
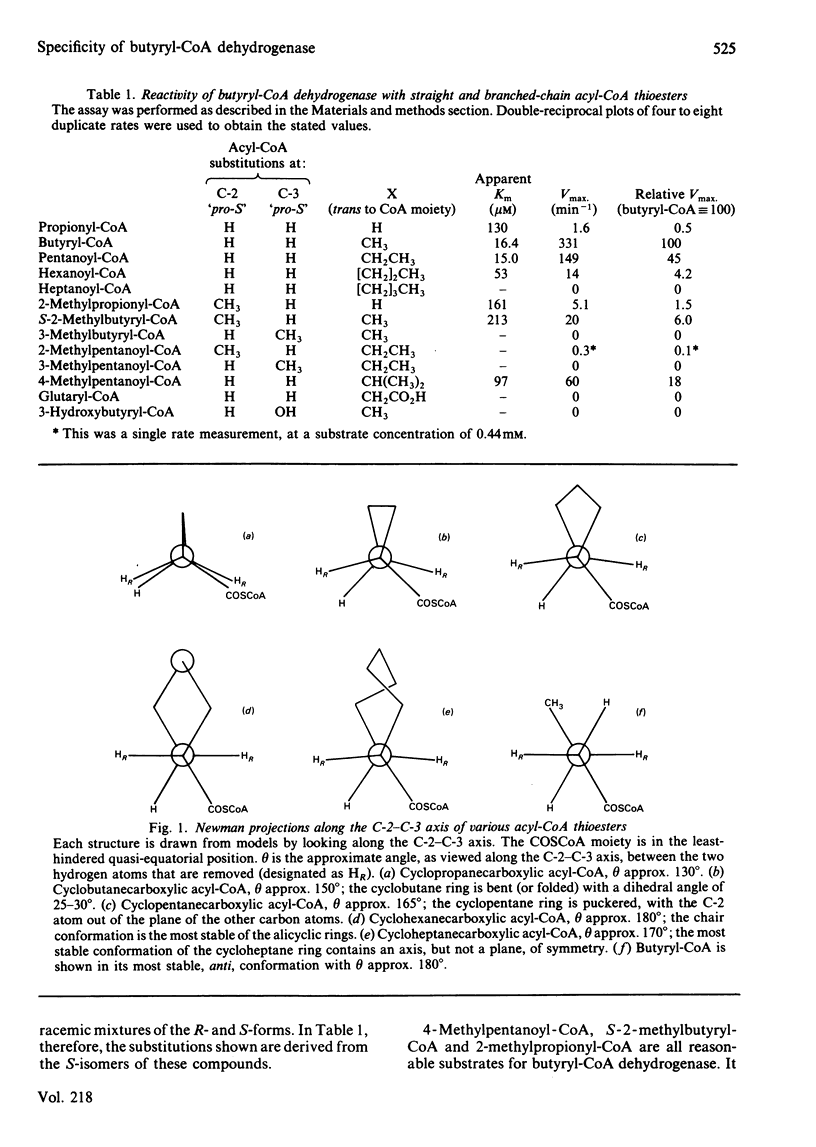
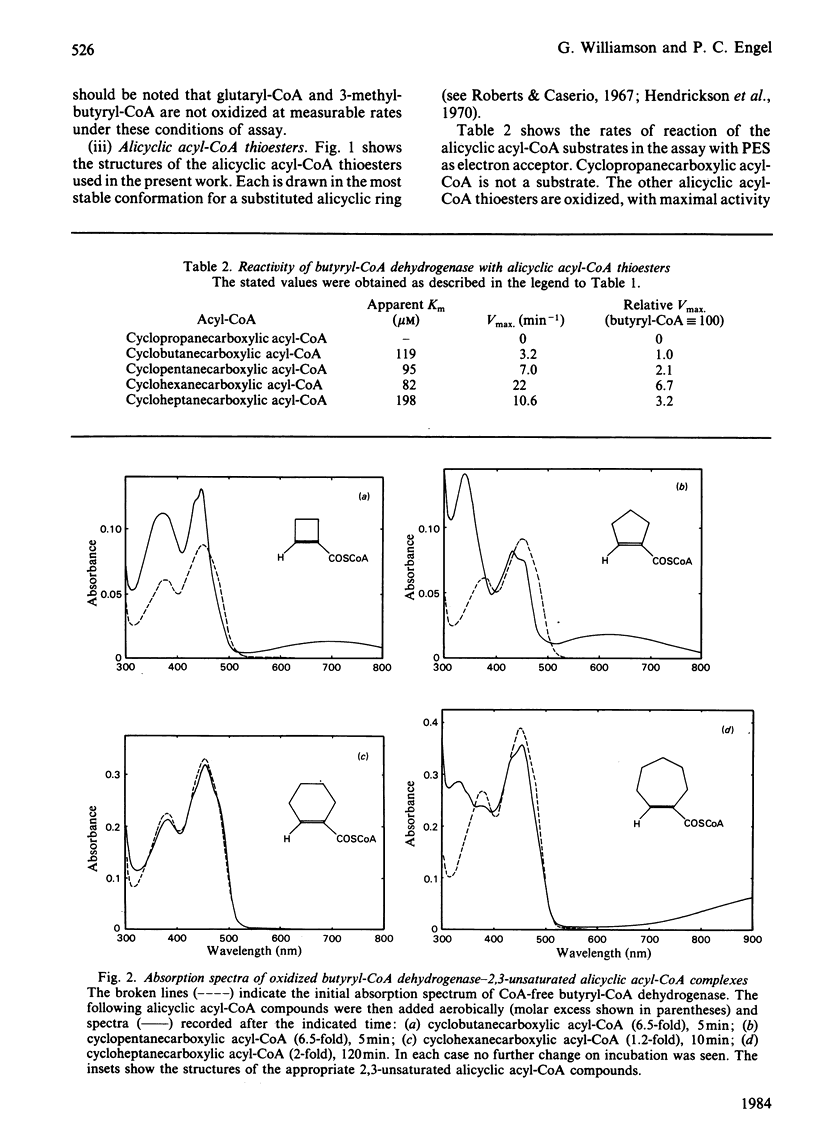
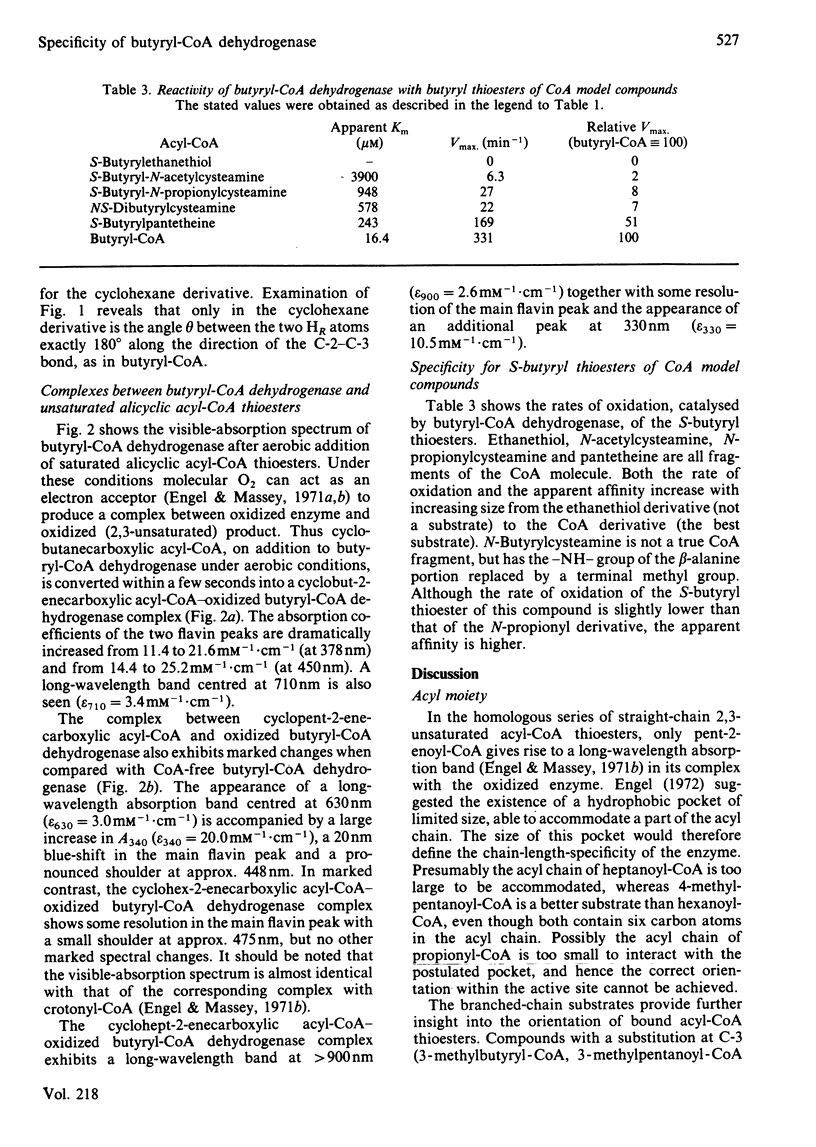
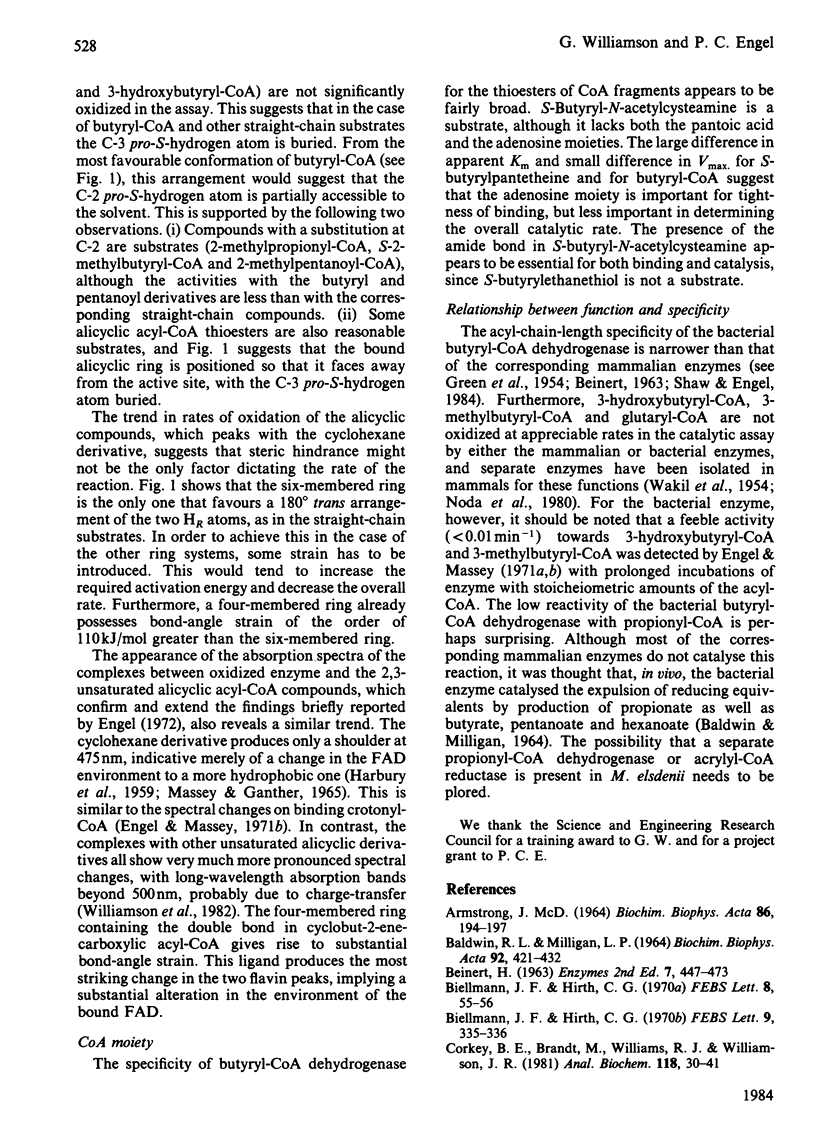
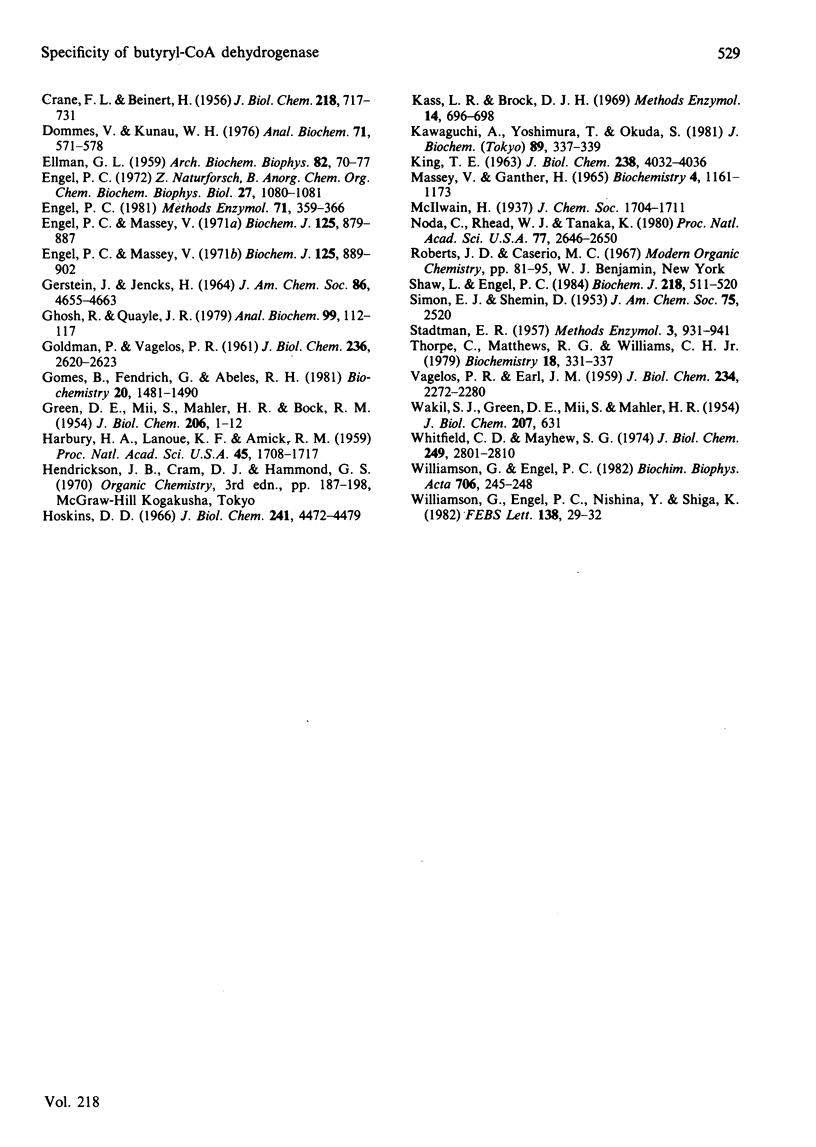
Selected References
These references are in PubMed. This may not be the complete list of references from this article.
- ARMSTRONG J. M. THE MOLAR EXTINCTION COEFFICIENT OF 2,6-DICHLOROPHENOL INDOPHENOL. Biochim Biophys Acta. 1964 Apr 4;86:194–197. doi: 10.1016/0304-4165(64)90180-1. [DOI] [PubMed] [Google Scholar]
- BALDWIN R. L., MILLIGAN L. P. ELECTRON TRANSPORT IN PEPTOSTREPTOCOCCUS ELSDENII. Biochim Biophys Acta. 1964 Dec 23;92:421–432. doi: 10.1016/0926-6569(64)90001-x. [DOI] [PubMed] [Google Scholar]
- Biellmann J. F., Hirth C. G. Stereochemistry of the oxidation at the alpha carbon of butyryl-CoA and of the enzymic hydrogen exchange. FEBS Lett. 1970 Sep 7;9(6):335–336. doi: 10.1016/0014-5793(70)80393-3. [DOI] [PubMed] [Google Scholar]
- Biellmann J. F., Hirth C. G. Stereochemistry of the oxidation at the beta-carbon of butyryl-SCoA. FEBS Lett. 1970 May 11;8(1):55–56. doi: 10.1016/0014-5793(70)80224-1. [DOI] [PubMed] [Google Scholar]
- CRANE F. L., BEINERT H. On the mechanism of dehydrogenation of fatty acyl derivatives of coenzyme A. II. The electron-transferring flavoprotein. J Biol Chem. 1956 Feb;218(2):717–731. [PubMed] [Google Scholar]
- Corkey B. E., Brandt M., Williams R. J., Williamson J. R. Assay of short-chain acyl coenzyme A intermediates in tissue extracts by high-pressure liquid chromatography. Anal Biochem. 1981 Nov 15;118(1):30–41. doi: 10.1016/0003-2697(81)90152-4. [DOI] [PubMed] [Google Scholar]
- Dommes V., Kunau W. H. A convenient assay for acyl-CoA-dehydrogenases. Anal Biochem. 1976 Apr;71(2):571–578. doi: 10.1016/s0003-2697(76)80026-7. [DOI] [PubMed] [Google Scholar]
- ELLMAN G. L. Tissue sulfhydryl groups. Arch Biochem Biophys. 1959 May;82(1):70–77. doi: 10.1016/0003-9861(59)90090-6. [DOI] [PubMed] [Google Scholar]
- Engel P. C. Butyryl coenzyme A dehydrogenase: studies on the specificity of the formation of acyl coenzyme A complexes with long-wavelength absorption. Z Naturforsch B. 1972 Sep;27(9):1080–1081. doi: 10.1515/znb-1972-0926. [DOI] [PubMed] [Google Scholar]
- GOLDMAN P., VAGELOS P. R. The specificity of triglyceride synthesis from diglycerides in chicken adipose tissue. J Biol Chem. 1961 Oct;236:2620–2623. [PubMed] [Google Scholar]
- GREEN D. E., MII S., MAHLER H. R., BOCK R. M. Studies on the fatty acid oxidizing system of animal tissues. III. Butyryl coenzyme A dehydrogenase. J Biol Chem. 1954 Jan;206(1):1–12. [PubMed] [Google Scholar]
- Ghosh R., Quayle J. R. Phenazine ethosulfate as a preferred electron acceptor to phenazine methosulfate in dye-linked enzyme assays. Anal Biochem. 1979 Oct 15;99(1):112–117. doi: 10.1016/0003-2697(79)90050-2. [DOI] [PubMed] [Google Scholar]
- Gomes B., Fendrich G., Abeles R. H. Mechanism of action of glutaryl-CoA and butyryl-CoA dehydrogenases. Purification of glutaryl-CoA dehydrogenase. Biochemistry. 1981 Mar 17;20(6):1481–1490. doi: 10.1021/bi00509a012. [DOI] [PubMed] [Google Scholar]
- Harbury H. A., Lanoue K. F., Loach P. A., Amick R. M. MOLECULAR INTERACTION OF ISOALLOXAZINE DERIVATIVES. II. Proc Natl Acad Sci U S A. 1959 Dec;45(12):1708–1717. doi: 10.1073/pnas.45.12.1708. [DOI] [PMC free article] [PubMed] [Google Scholar]
- Hoskins D. D. The electron-transferring flavoprotein as a common intermediate in the mitochondrial oxidation of butyryl coenzyme A and sarcosine. J Biol Chem. 1966 Oct 10;241(19):4472–4479. [PubMed] [Google Scholar]
- KING T. E. RECONSTITUTION OF RESPIRATORY CHAIN ENZYME SYSTEMS. XI. USE OF ARTIFICIAL ELECTRON ACCEPTORS IN THE ASSAY OF SUCCINATE-DEHYDROGENATING ENZYMES. J Biol Chem. 1963 Dec;238:4032–4036. [PubMed] [Google Scholar]
- Kawaguchi A., Yoshimura T., Okuda S. A new method for the preparation of acyl-CoA thioesters. J Biochem. 1981 Feb;89(2):337–339. doi: 10.1093/oxfordjournals.jbchem.a133207. [DOI] [PubMed] [Google Scholar]
- Massey V., Ganther H. On the interpretation of the absorption spectra of flavoproteins with special reference to D-amino acid oxidase. Biochemistry. 1965 Jun;4(6):1161–1173. doi: 10.1021/bi00882a027. [DOI] [PubMed] [Google Scholar]
- Noda C., Rhead W. J., Tanaka K. Isovaleryl-CoA dehydrogenase: demonstration in rat liver mitochondria by ion exchange chromatography and isoelectric focusing. Proc Natl Acad Sci U S A. 1980 May;77(5):2646–2650. doi: 10.1073/pnas.77.5.2646. [DOI] [PMC free article] [PubMed] [Google Scholar]
- Shaw L., Engel P. C. The purification and properties of ox liver short-chain acyl-CoA dehydrogenase. Biochem J. 1984 Mar 1;218(2):511–520. doi: 10.1042/bj2180511. [DOI] [PMC free article] [PubMed] [Google Scholar]
- Thorpe C., Matthews R. G., Williams C. H., Jr Acyl-coenzyme A dehydrogenase from pig kidney. Purification and properties. Biochemistry. 1979 Jan 23;18(2):331–337. doi: 10.1021/bi00569a016. [DOI] [PubMed] [Google Scholar]
- VAGELOS P. R., EARL J. M. Propionic acid metabolism. III. beta-Hydroxypropionyl coenzyme A and malonyl semialdehyde coenzyme A, intermediates in propionate oxidation by Clostridium kluyveri. J Biol Chem. 1959 Sep;234:2272–2280. [PubMed] [Google Scholar]
- WAKIL S. J., GREEN D. E., MII S., MAHLER H. R. Studies on the fatty acid oxidizing system of animal tissues. VI. beta-Hydroxyacyl coenzyme A dehydrogenase. J Biol Chem. 1954 Apr;207(2):631–638. [PubMed] [Google Scholar]
- Whitfield C. D., Mayhew S. G. Purification and properties of electron-transferring flavoprotein from Peptostreptococcus elsdenii. J Biol Chem. 1974 May 10;249(9):2801–2810. [PubMed] [Google Scholar]
- Williamson G., Engel P. C. A convenient and rapid method for the complete removal of CoA from butyryl-CoA dehydrogenase. Biochim Biophys Acta. 1982 Sep 7;706(2):245–248. doi: 10.1016/0167-4838(82)90493-9. [DOI] [PubMed] [Google Scholar]
- Williamson G., Engel P. C., Nishina Y., Shiga K. A resonance Raman study on the nature of charge-transfer interactions in butyryl CoA dehydrogenase. FEBS Lett. 1982 Feb 8;138(1):29–32. doi: 10.1016/0014-5793(82)80387-6. [DOI] [PubMed] [Google Scholar]