Abstract
Cathepsin D inactivated aldolase at pH values between 4.2 and 5.2; the chloride, sulphate or iodide, but not citrate or acetate, salts of sodium or potassium accelerated the rate of inactivation. Cathepsin D cleaved numerous peptide bonds in the C-terminus of aldolase, but the major site of cleavage in this region was Leu354-Phe355. The most prominent peptide products of hydrolysis were Phe-Ile-Ser-Asn-His-Ala-Tyr and Phe-Ile-Ser-Asn-His. Up to 20 amino acids were removed from the C-terminus of aldolase, but no further degradation of native aldolase was observed. By contrast, extensive degradation of the 40 000-Mr subunit was observed after aldolase was denatured. The cathepsin D-inactivated aldolase cross-reacted with antibodies prepared against native aldolase and had the same thermodynamic stability as native aldolase, demonstrated by differential scanning calorimetry and fluorescence quenching of tryptophan residues. Furthermore, the cathepsin-modified and native forms of aldolase were both resistant to extensive proteolysis by other purified cellular proteinases and lysosomal extracts at pH values of 4.8-8.0.
Full text
PDF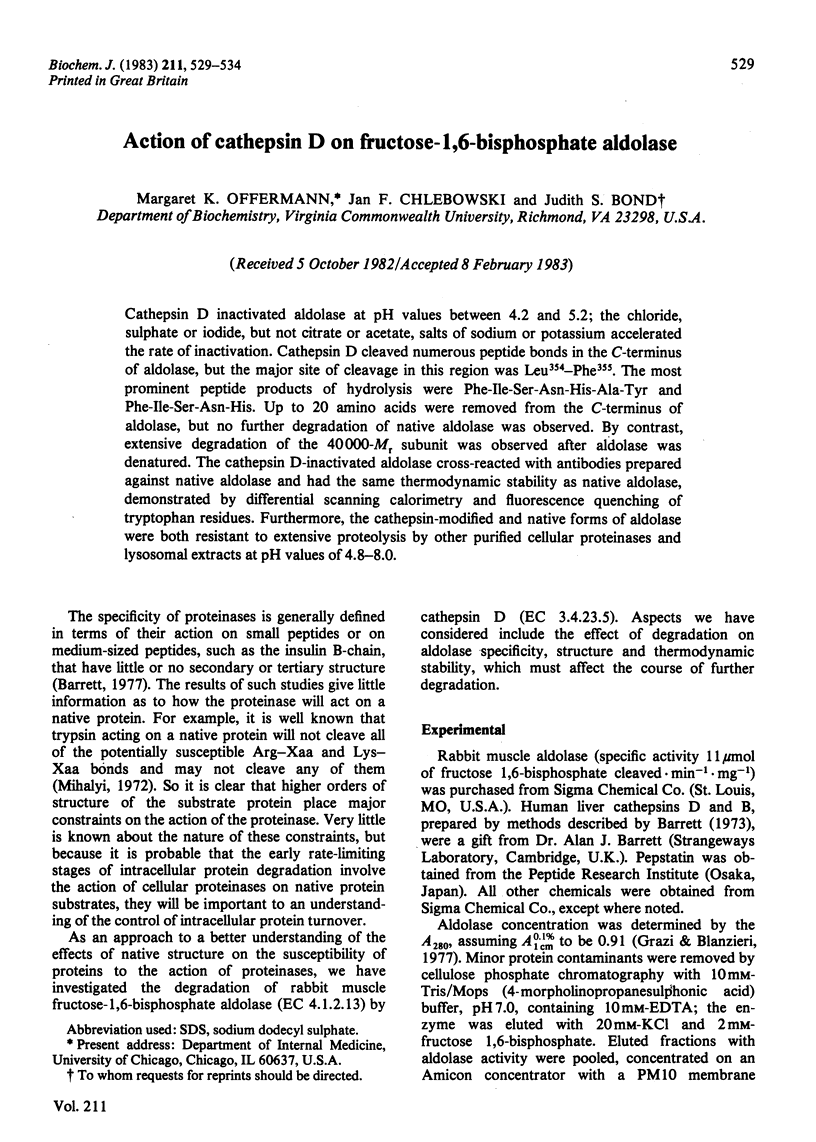
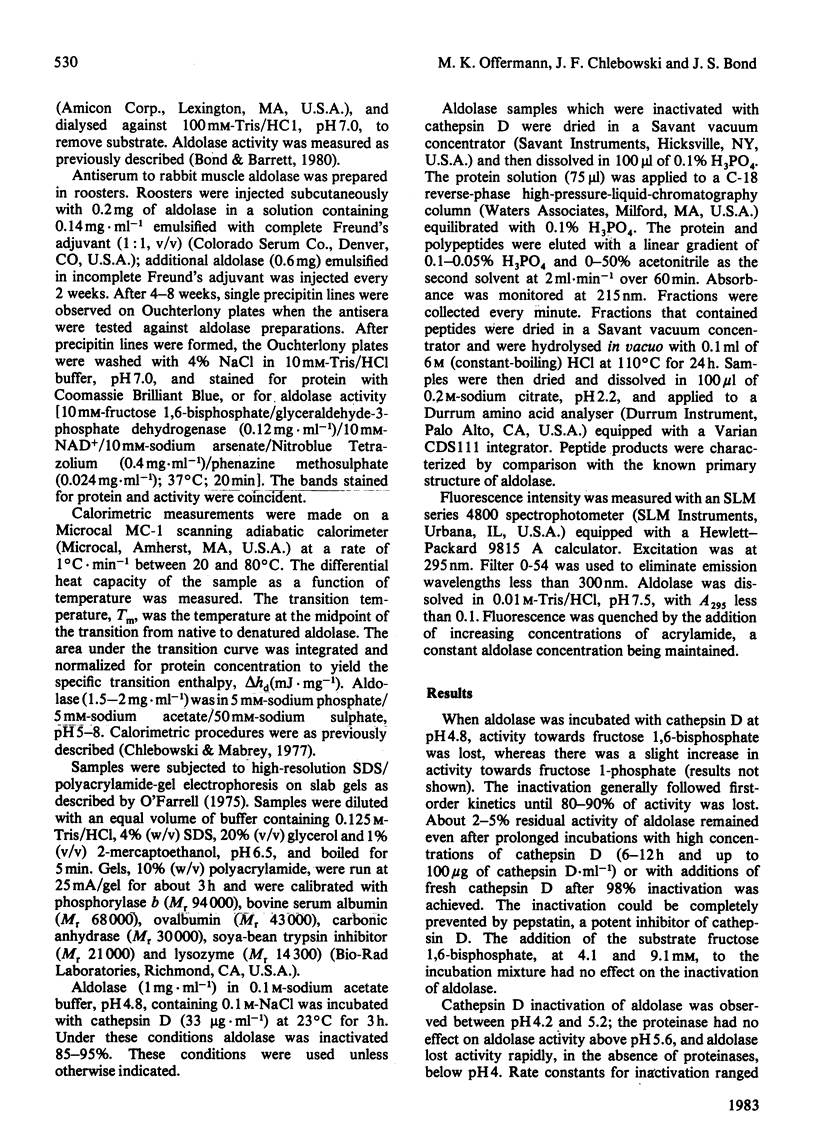
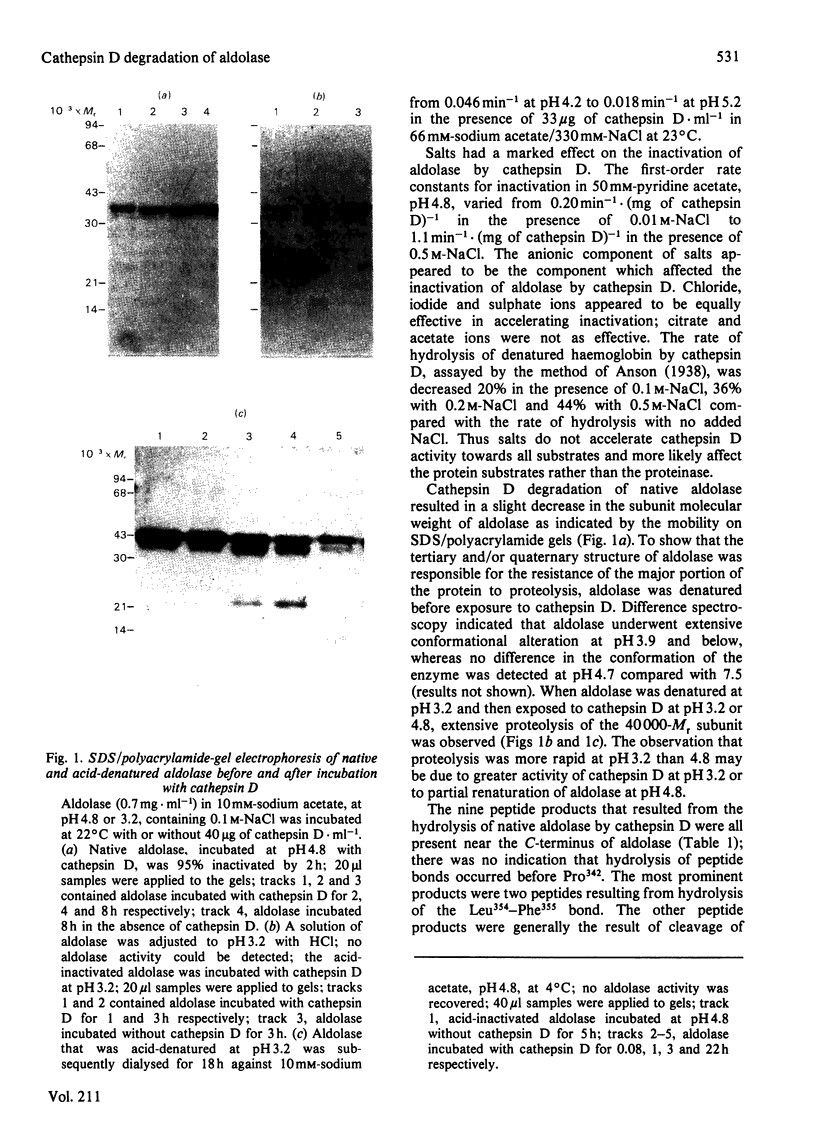
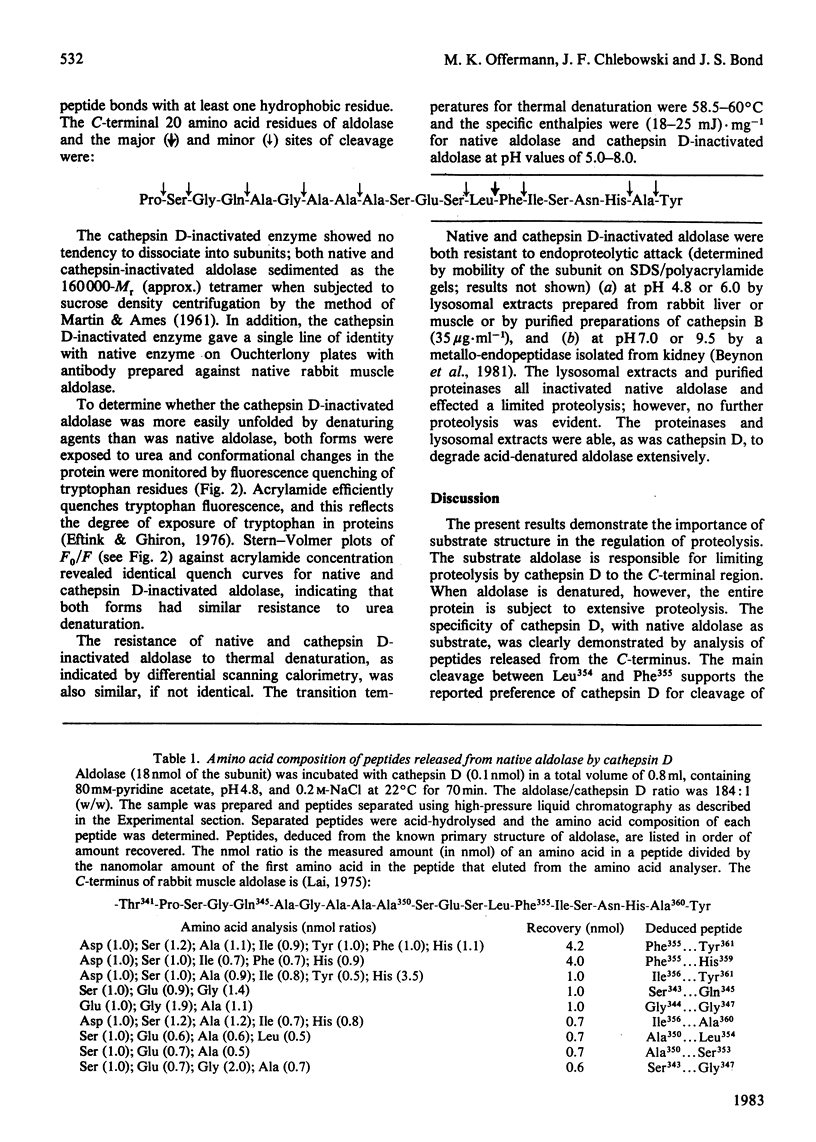
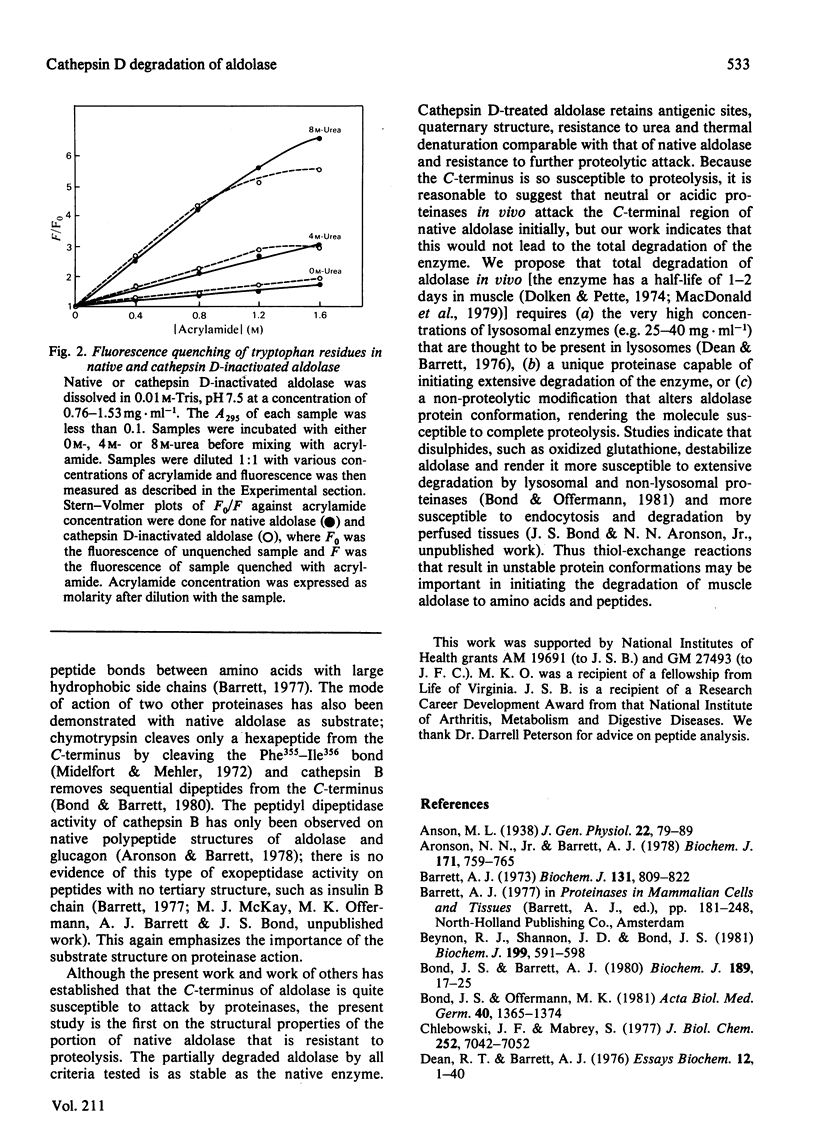
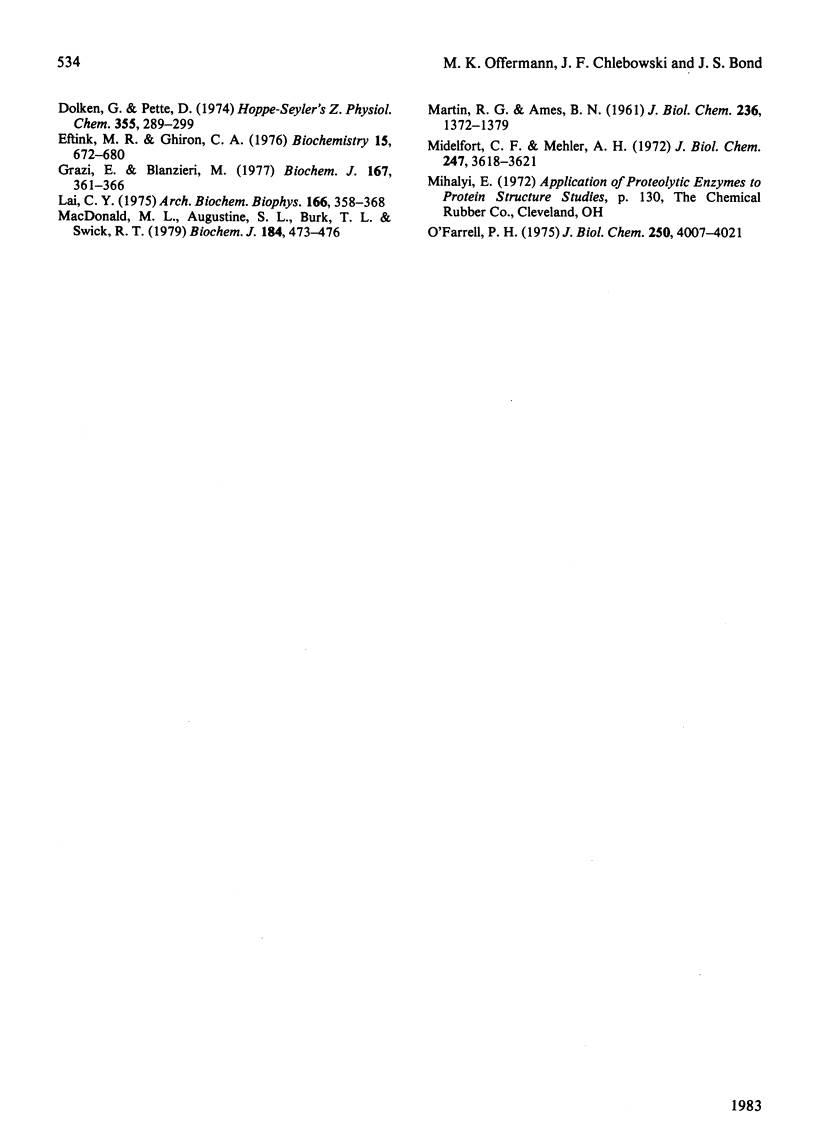
Images in this article
Selected References
These references are in PubMed. This may not be the complete list of references from this article.
- Aronson N. N., Jr, Barrett A. J. The specificity of cathepsin B. Hydrolysis of glucagon at the C-terminus by a peptidyldipeptidase mechanism. Biochem J. 1978 Jun 1;171(3):759–765. doi: 10.1042/bj1710759. [DOI] [PMC free article] [PubMed] [Google Scholar]
- Barrett A. J. Human cathepsin B1. Purification and some properties of the enzyme. Biochem J. 1973 Apr;131(4):809–822. doi: 10.1042/bj1310809. [DOI] [PMC free article] [PubMed] [Google Scholar]
- Beynon R. J., Shannon J. D., Bond J. S. Purification and characterization of a metallo-endoproteinase from mouse kidney. Biochem J. 1981 Dec 1;199(3):591–598. doi: 10.1042/bj1990591. [DOI] [PMC free article] [PubMed] [Google Scholar]
- Bond J. S., Barrett A. J. Degradation of fructose-1,6-bisphosphate aldolase by cathepsin B. Biochem J. 1980 Jul 1;189(1):17–25. doi: 10.1042/bj1890017. [DOI] [PMC free article] [PubMed] [Google Scholar]
- Bond J. S., Offermann M. K. Initial events in the degradation of soluble cellular enzymes: factors affecting the stability and proteolytic susceptibility of fructose-1,6-bisphosphate aldolase. Acta Biol Med Ger. 1981;40(10-11):1365–1374. [PubMed] [Google Scholar]
- Chlebowski J. F., Mabrey S. Differential scanning calorimetry of apo-, apophosphoryl, and metalloalkaline phosphatases. J Biol Chem. 1977 Oct 25;252(20):7042–7052. [PubMed] [Google Scholar]
- Dean R. T., Barrett A. J. Lysosomes. Essays Biochem. 1976;12:1–40. [PubMed] [Google Scholar]
- Dölken G., Pette D. Turnover of several glycolytic enzymes in rabbit heart, soleus muscle and liver. Hoppe Seylers Z Physiol Chem. 1974 Mar;355(3):289–299. doi: 10.1515/bchm2.1974.355.1.289. [DOI] [PubMed] [Google Scholar]
- Eftink M. R., Ghiron C. A. Exposure of tryptophanyl residues in proteins. Quantitative determination by fluorescence quenching studies. Biochemistry. 1976 Feb 10;15(3):672–680. doi: 10.1021/bi00648a035. [DOI] [PubMed] [Google Scholar]
- Grazi E., Blanzieri M. Fructose 1,6-bisphosphate aldolase from rabbit muscle. The isomerization of the enzyme-dihydroxyacetone phosphate complex. Biochem J. 1977 Nov 1;167(2):361–366. doi: 10.1042/bj1670361. [DOI] [PMC free article] [PubMed] [Google Scholar]
- Lai C. Y. Studies on the structure of rabbit muscle aldolase. Determination of the primary structure of the COOH-terminal BrCN peptide; the complete sequence of the subunit polypeptide chain. Arch Biochem Biophys. 1975 Jan;166(1):358–368. doi: 10.1016/0003-9861(75)90398-7. [DOI] [PubMed] [Google Scholar]
- MARTIN R. G., AMES B. N. A method for determining the sedimentation behavior of enzymes: application to protein mixtures. J Biol Chem. 1961 May;236:1372–1379. [PubMed] [Google Scholar]
- MacDonald M. L., Augustine S. L., Burk T. L., Swick R. W. A comparison of methods for the measurement of protein turnover in vivo. Biochem J. 1979 Nov 15;184(2):473–476. doi: 10.1042/bj1840473. [DOI] [PMC free article] [PubMed] [Google Scholar]
- Midelfort C. F., Mehler A. H. A chymotrypsin-catalyzed modification of rabbit muscle aldolase. J Biol Chem. 1972 Jun 10;247(11):3618–3621. [PubMed] [Google Scholar]
- O'Farrell P. H. High resolution two-dimensional electrophoresis of proteins. J Biol Chem. 1975 May 25;250(10):4007–4021. [PMC free article] [PubMed] [Google Scholar]