Abstract
1. Neurones enzymatically dissociated from the rat dorsal lateral geniculate nucleus (LGN) were identified as GABAergic local circuit interneurones and geniculocortical relay cells, based upon quantitative analysis of soma profiles, immunohistochemical detection of GABA or glutamic acid decarboxylase, and basic electrogenic behaviour. 2. During whole-cell current-clamp recording, isolated LGN neurones generated firing patterns resembling those in intact tissue, with the most striking difference relating to the presence in relay cells of a Ca2+ action potential with a low threshold of activation, capable of triggering fast spikes, and the absence of a regenerative Ca2+ response with a low threshold of activation in local circuit cells. 3. Whole-cell voltage-clamp experiments demonstrated that both classes of LGN neurones possess at least two voltage-dependent membrane currents which operate in a range of membrane potentials negative to the threshold for generation of Na(+)-K(+)-mediated spikes: the T-type Ca2+ current (IT) and an A-type K+ current (IA). Taking into account the differences in membrane surface area, the average size of IT was similar in the two types of neurones, and interneurones possessed a slightly larger A-conductance. 4. In local circuit neurones, the ranges of steady-state inactivation and activation of IT and IA were largely overlapping (VH = 81.1 vs. -82.8 mV), both currents activated at around -70 mV, and they rapidly increased in amplitude with further depolarization. In relay cells, the inactivation curve of IT was negatively shifted along the voltage axis by about 20 mV compared with that of IA (Vh = -86.1 vs. -69.2 mV), and the activation threshold for IT (at -80 mV) was 20 mV more negative than that for IA. In interneurones, the activation range of IT was shifted to values more positive than that in relay cells (Vh = -54.9 vs. -64.5 mV), whereas the activation range of IA was more negative (Vh = -25.2 vs. -14.5 mV). 5. Under whole-cell voltage-clamp conditions that allowed the combined activation of Ca2+ and K+ currents, depolarizing voltage steps from -110 mV evoked inward currents resembling IT in relay cells and small outward currents indicative of IA in local circuit neurones. After blockade of IA with 4-aminopyridine (4-AP), the same pulse protocol produced IT in both types of neurones. Under current clamp, 4-AP unmasked a regenerative membrane depolarization with a low threshold of activation capable of triggering fast spikes in local circuit neurones.(ABSTRACT TRUNCATED AT 400 WORDS)
Full text
PDF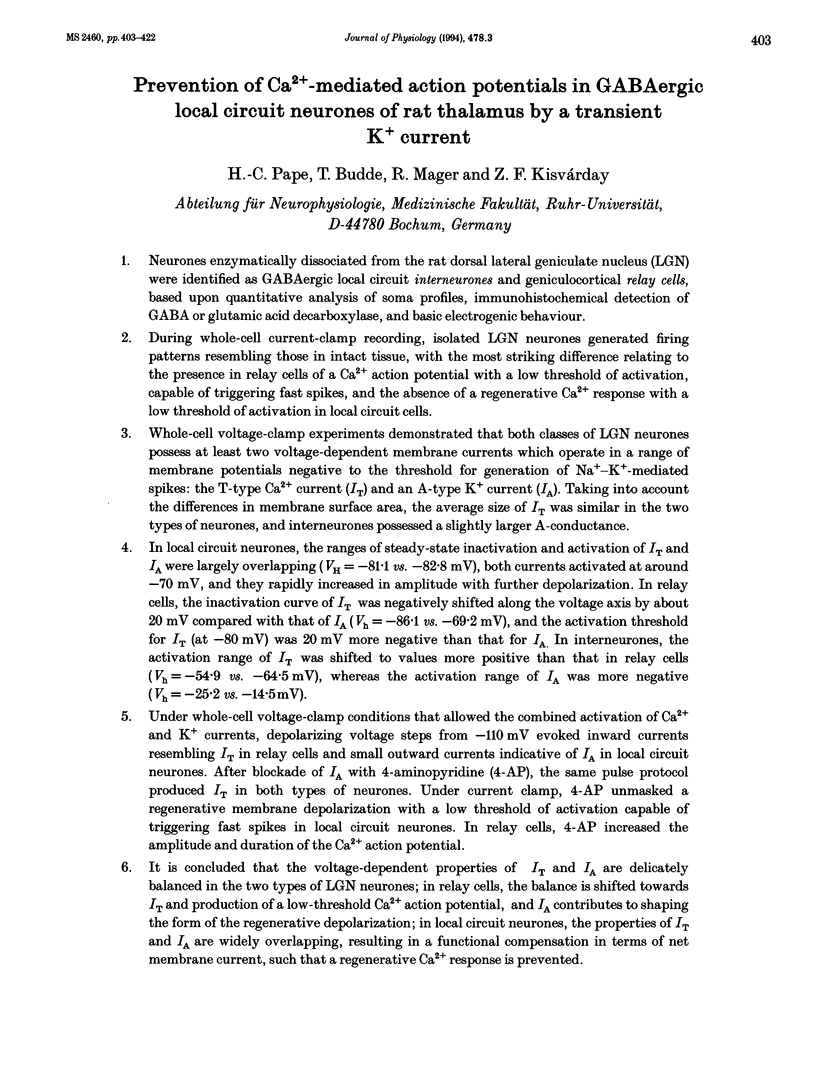
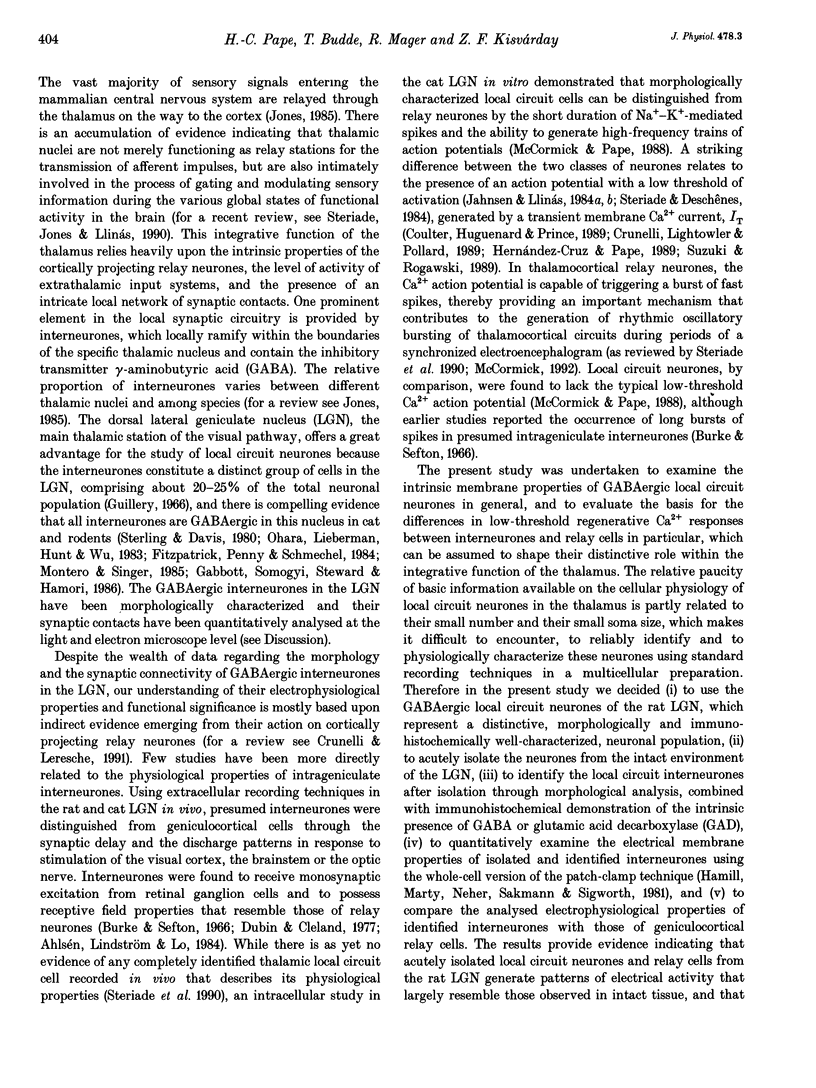
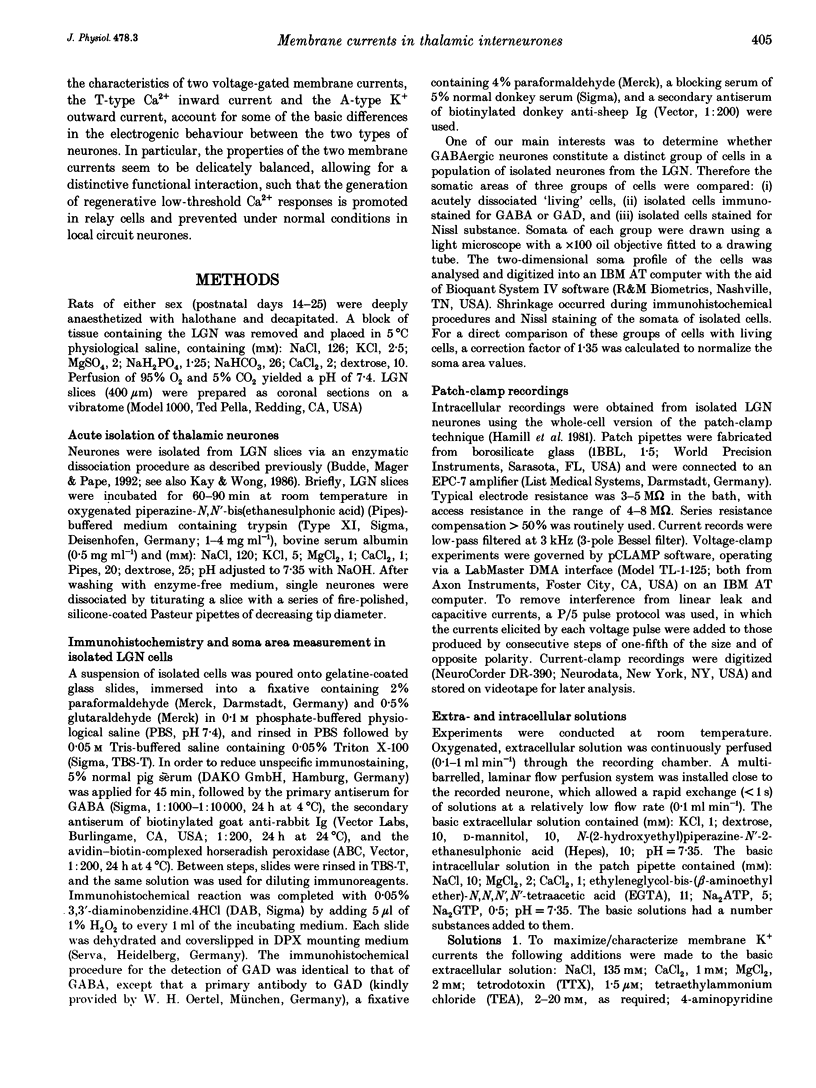
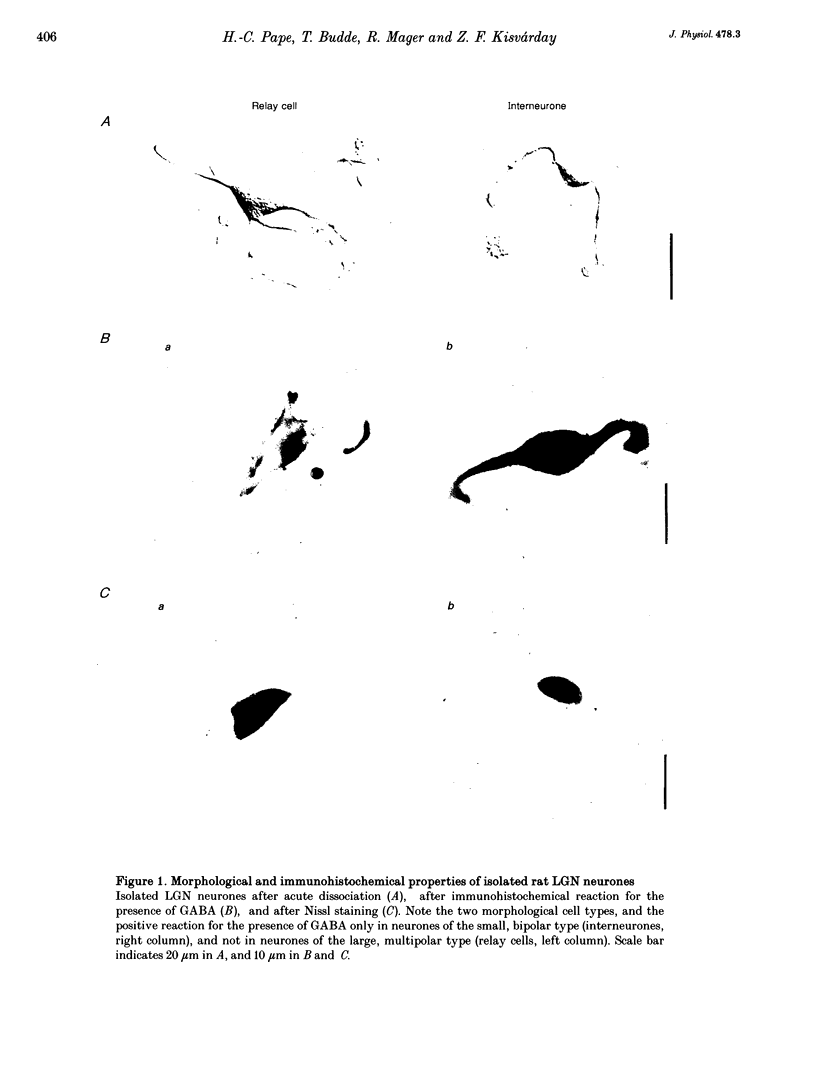
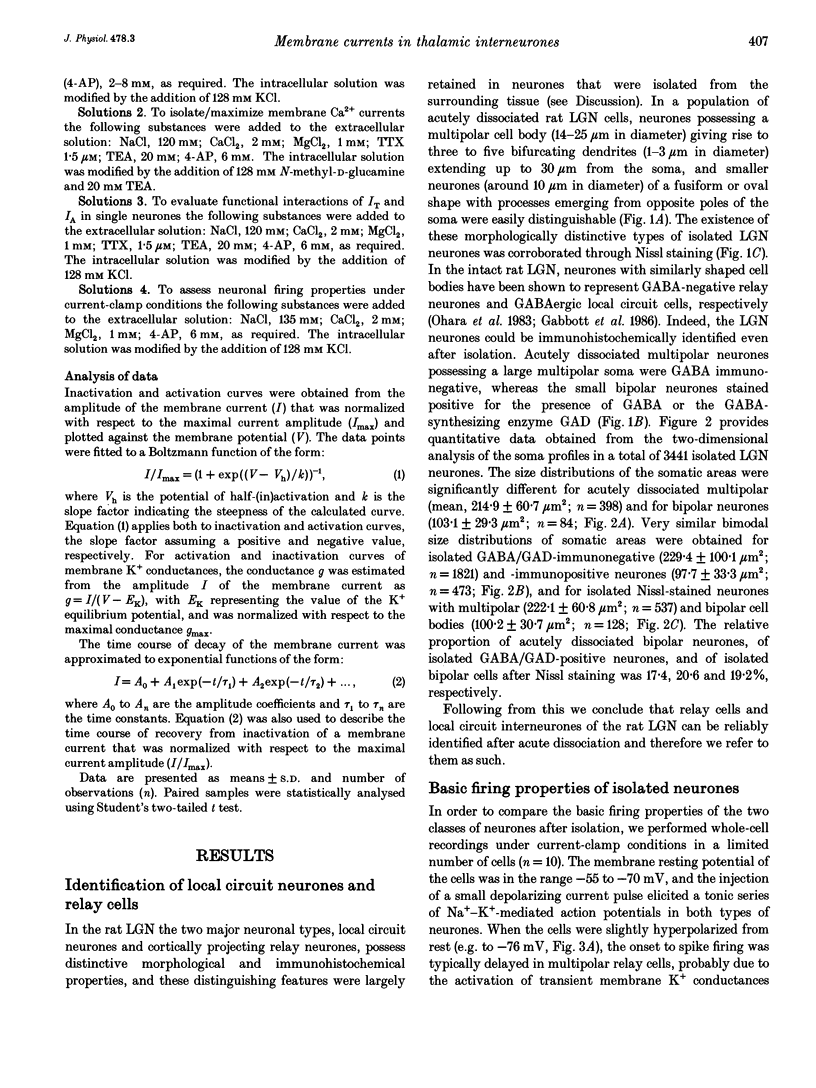
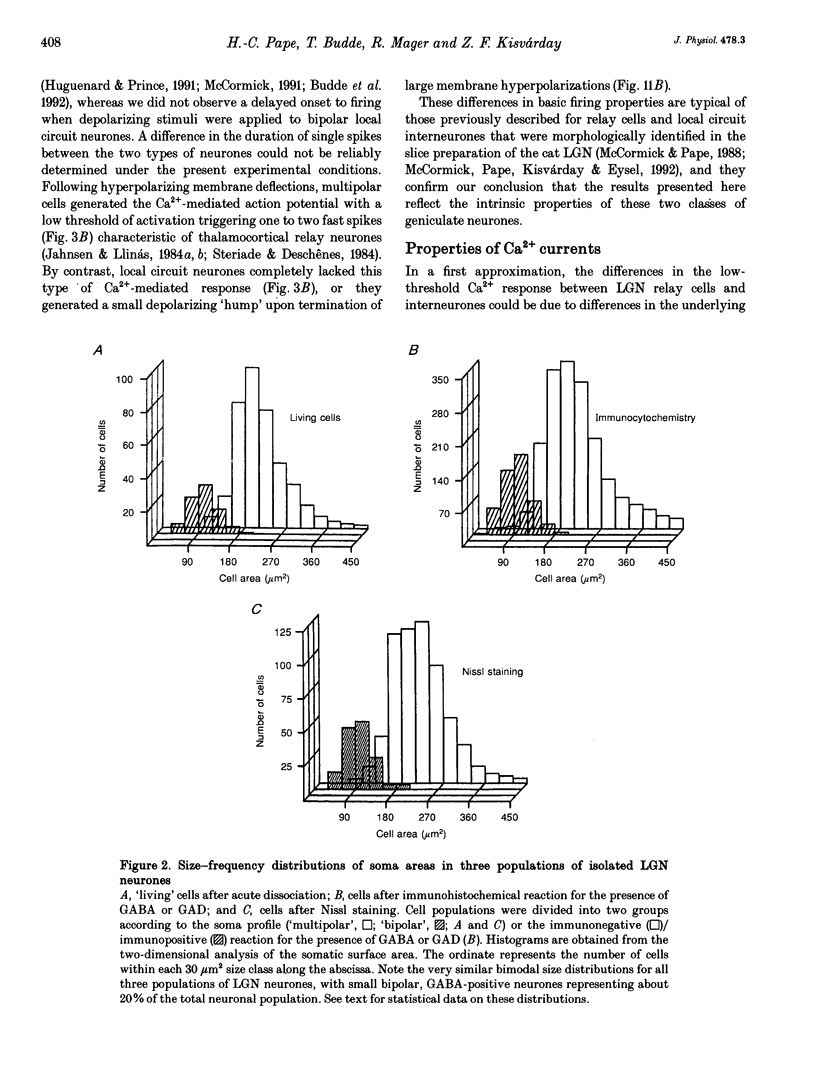
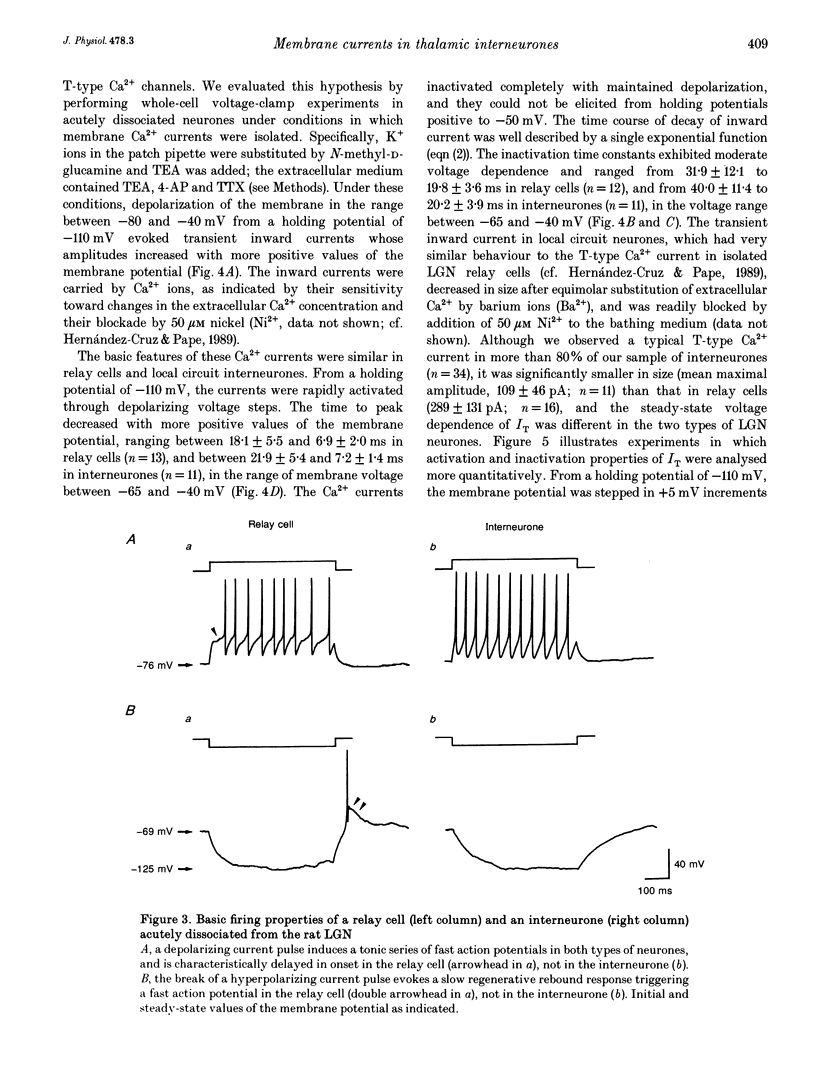
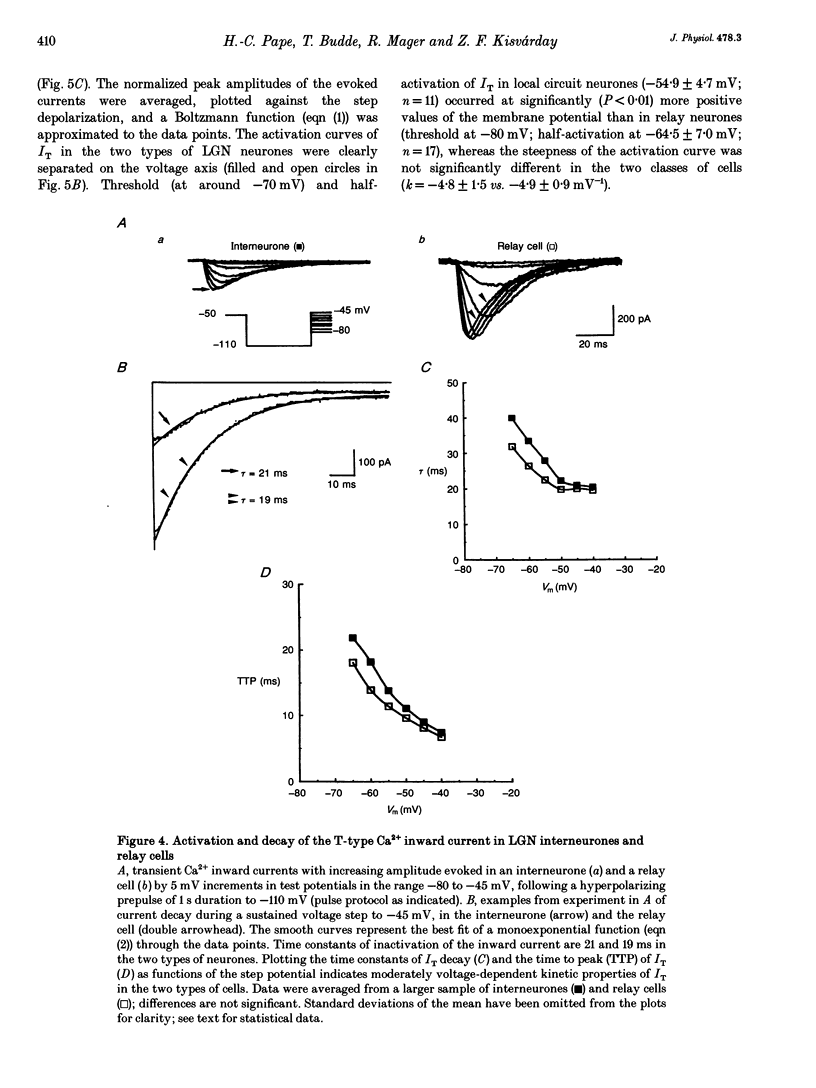
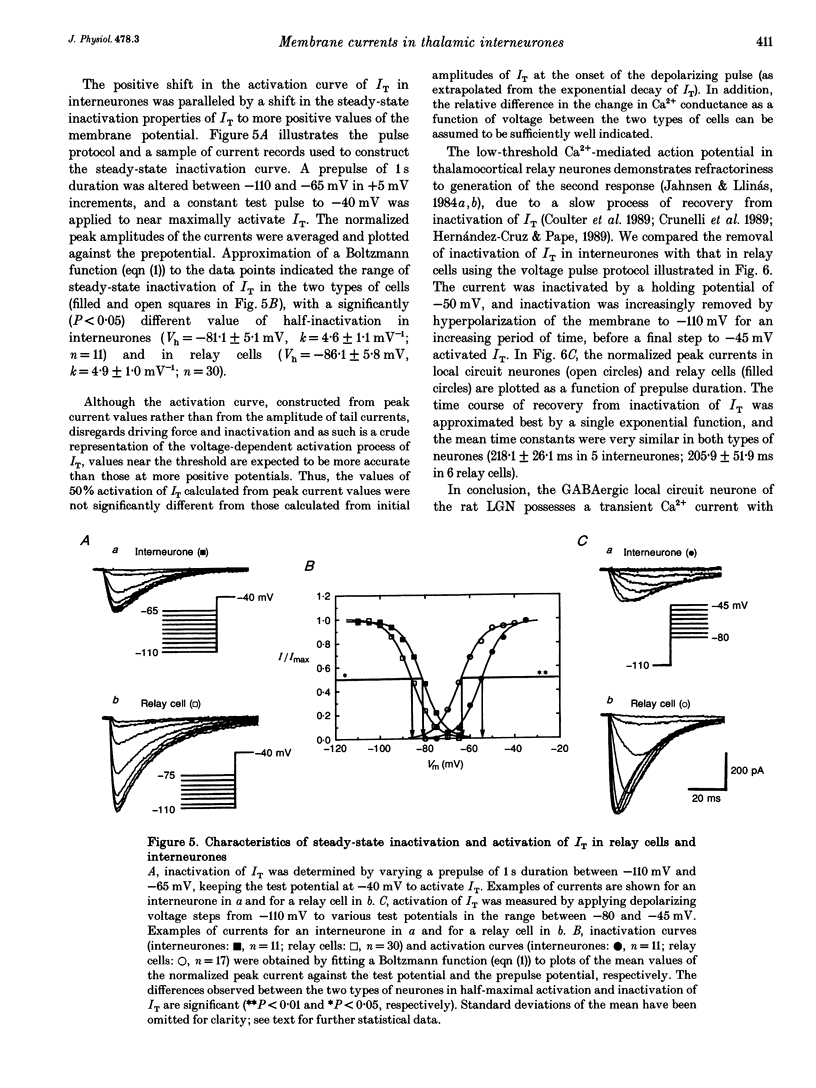
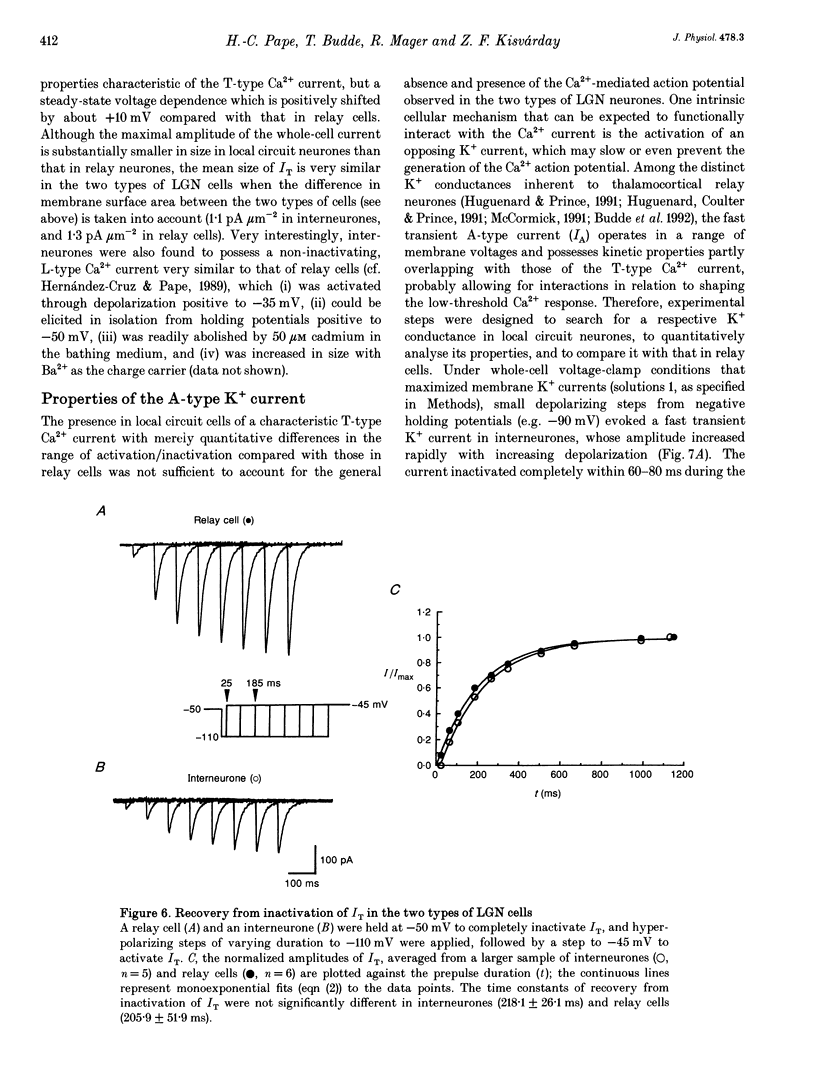
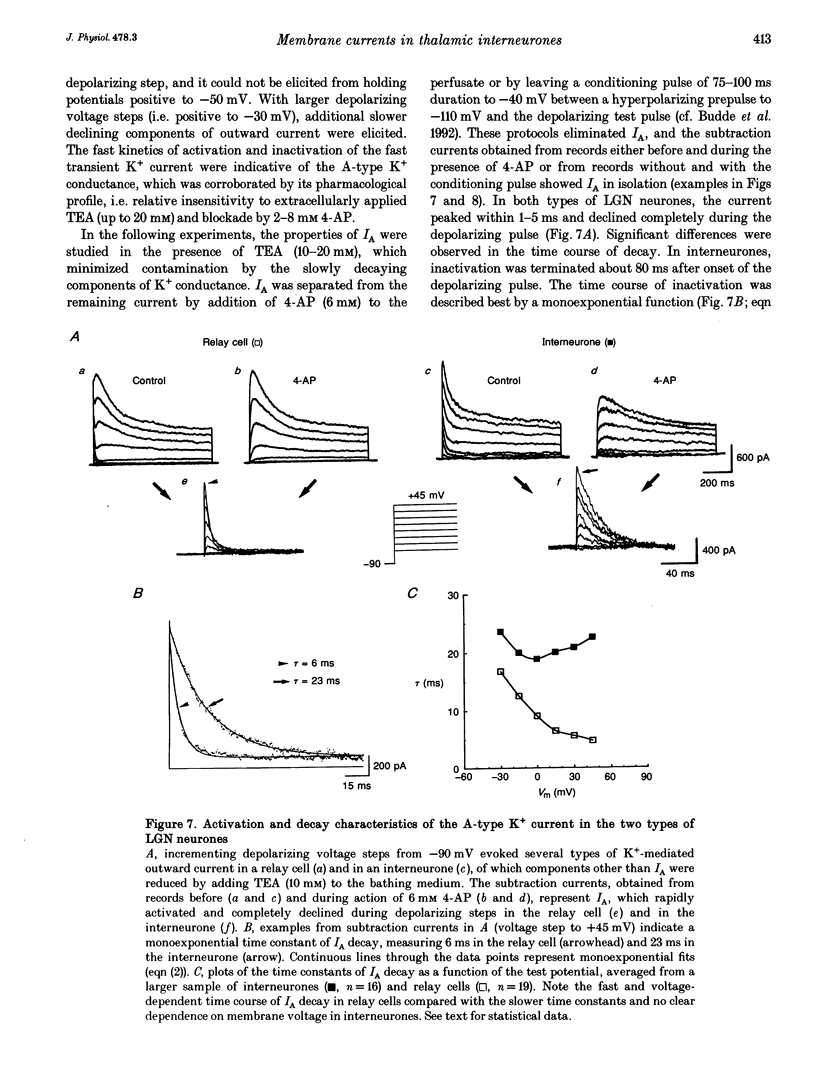
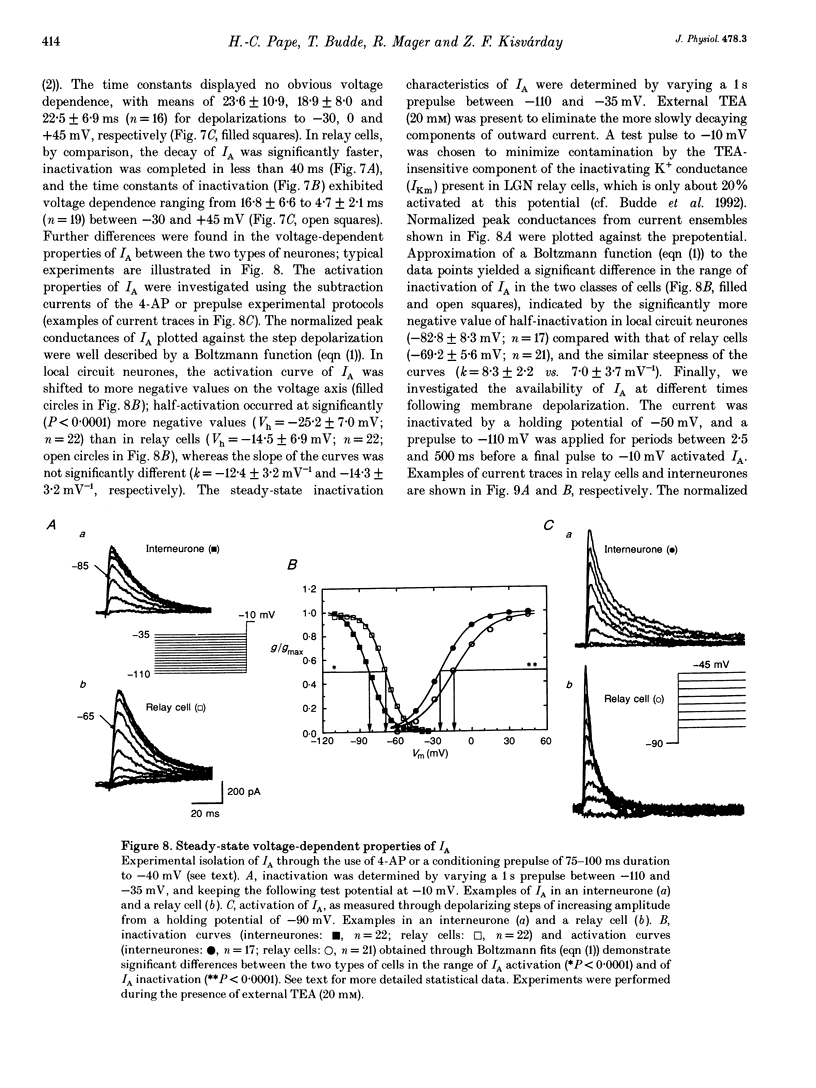
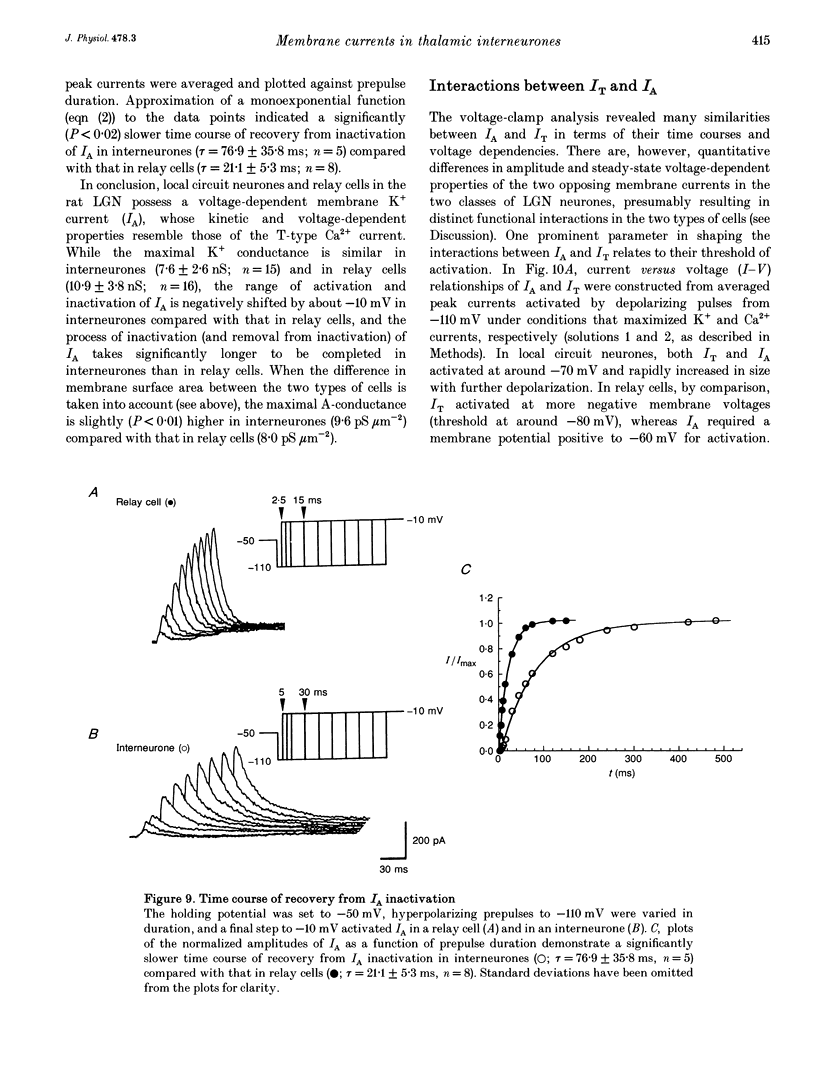
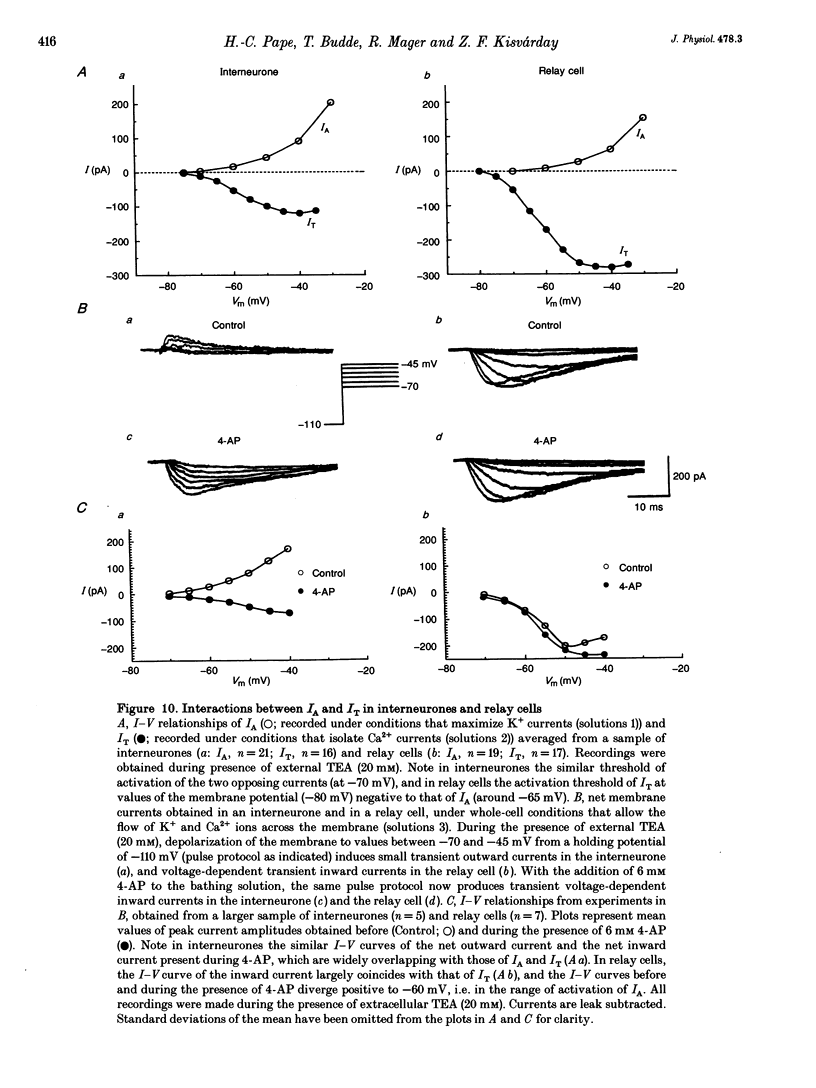
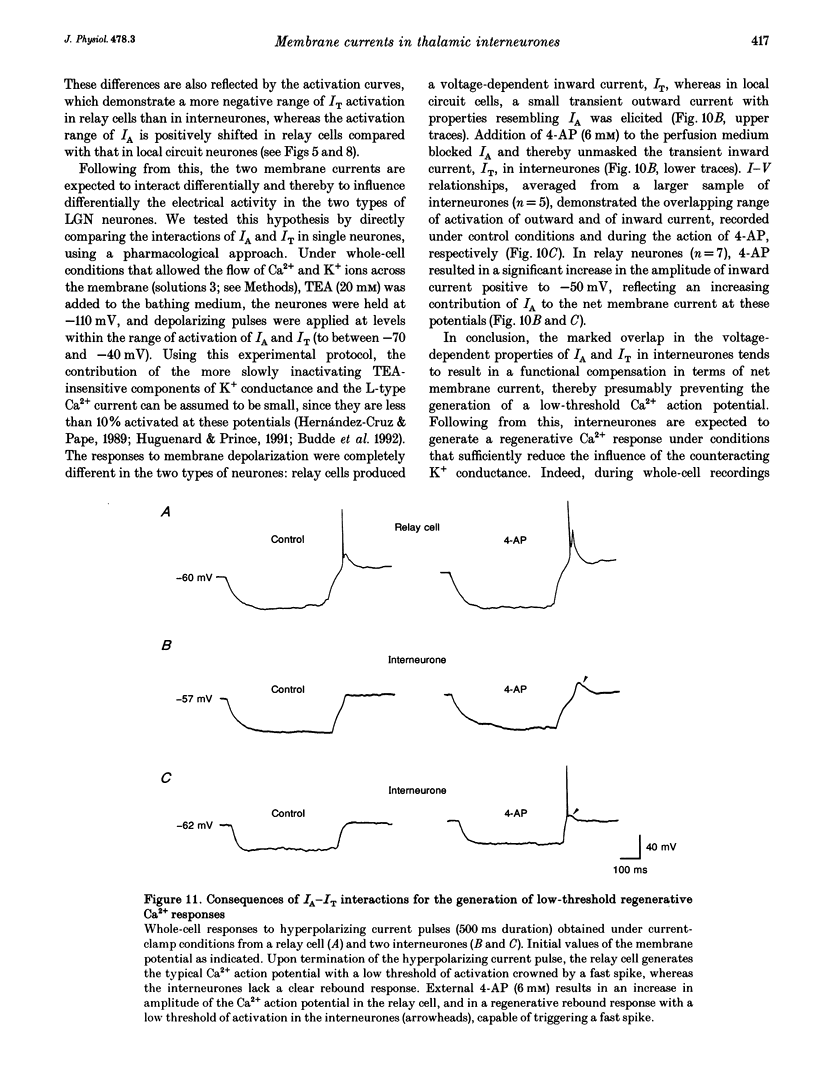
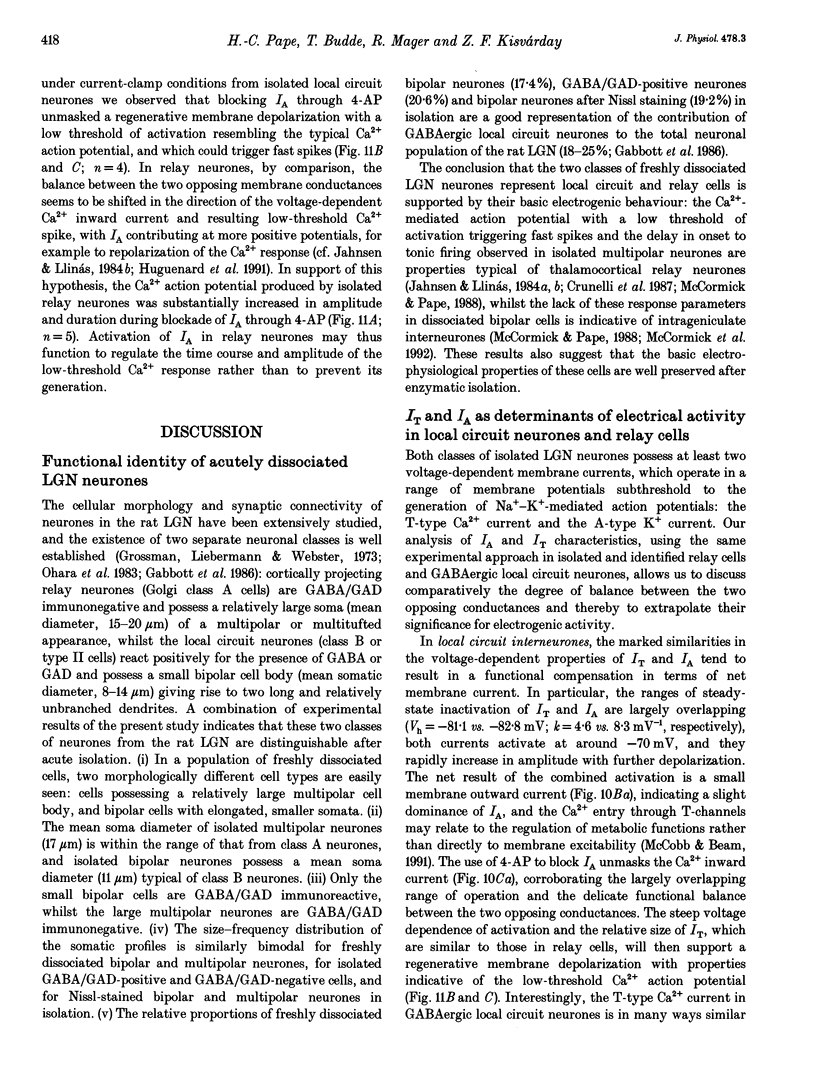
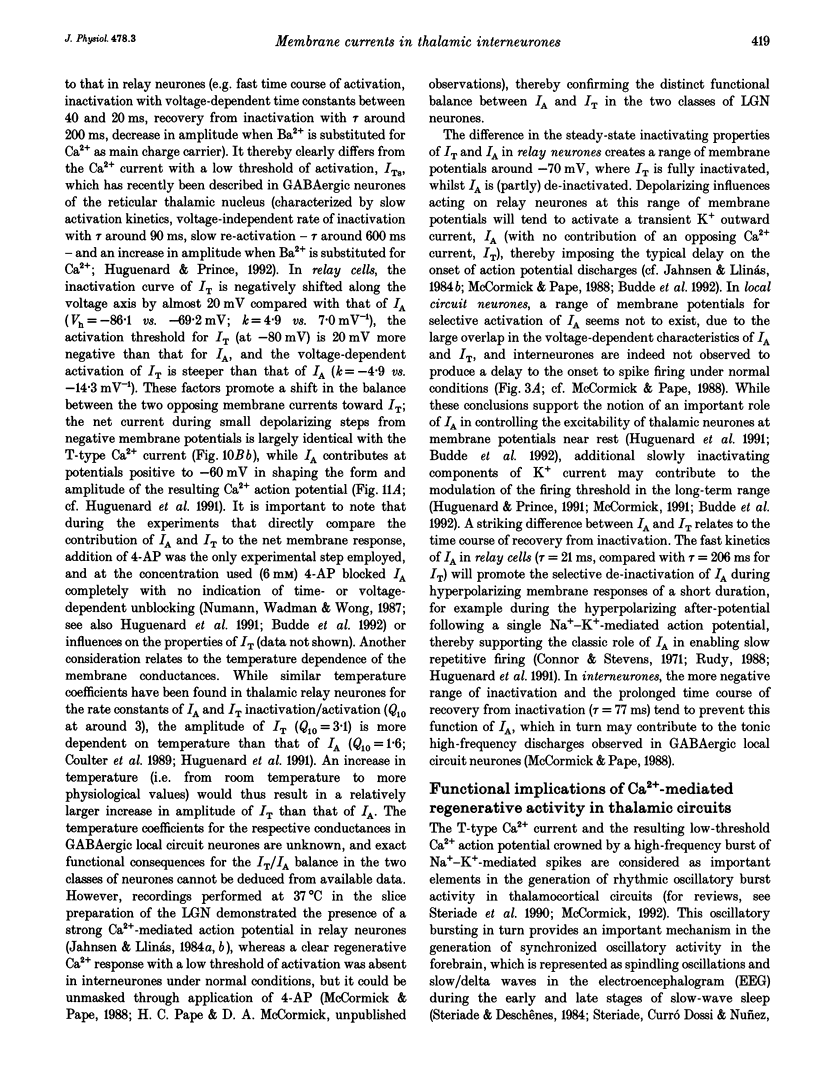
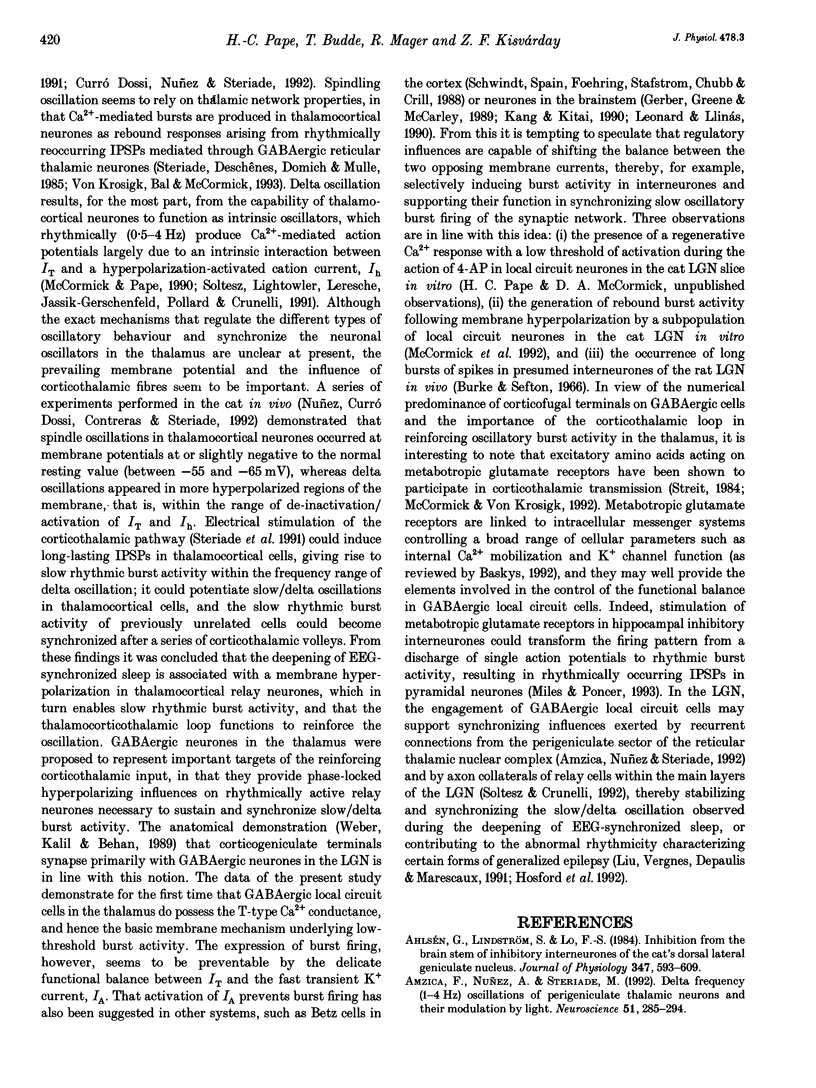
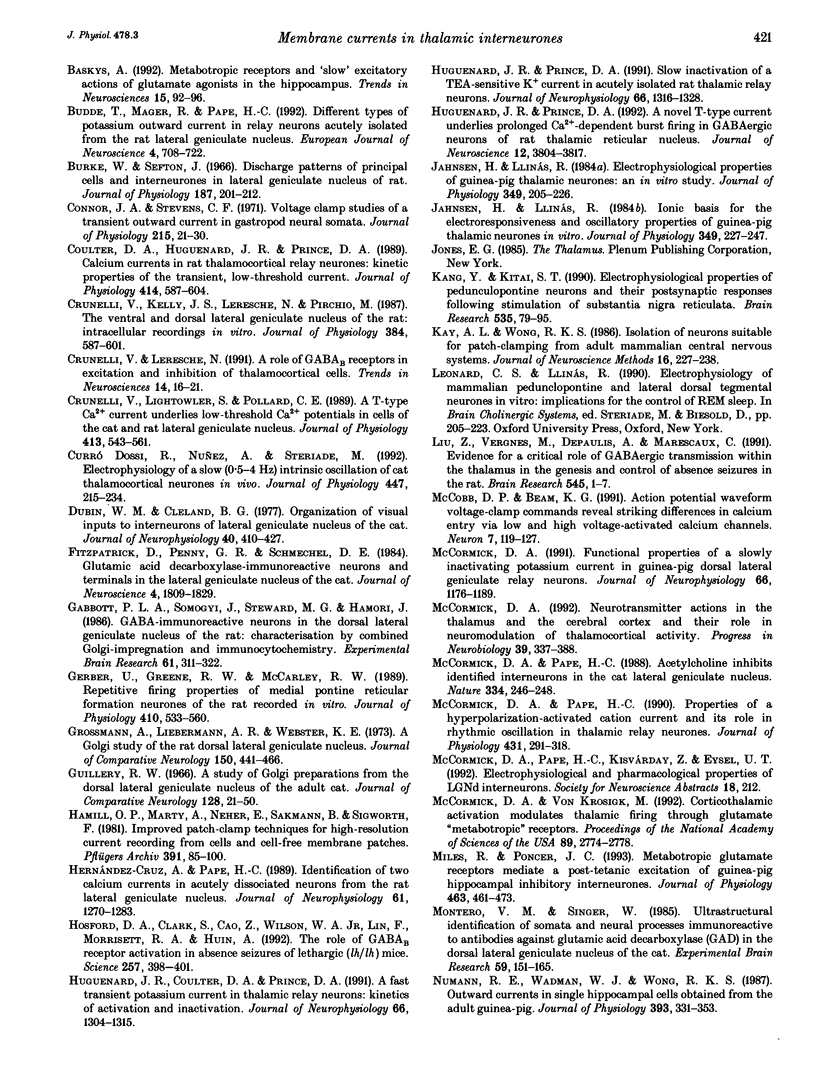
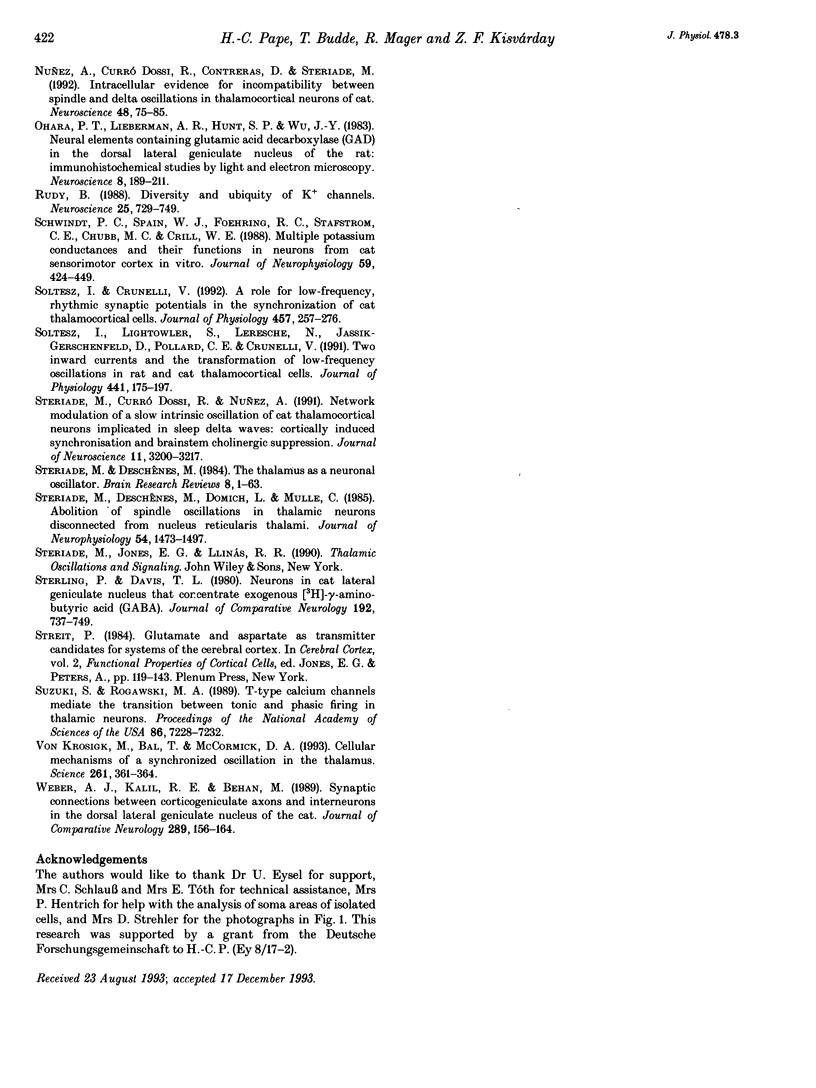
Images in this article
Selected References
These references are in PubMed. This may not be the complete list of references from this article.
- Ahlsén G., Lindström S., Lo F. S. Inhibition from the brain stem of inhibitory interneurones of the cat's dorsal lateral geniculate nucleus. J Physiol. 1984 Feb;347:593–609. doi: 10.1113/jphysiol.1984.sp015085. [DOI] [PMC free article] [PubMed] [Google Scholar]
- Amzica F., Nuñez A., Steriade M. Delta frequency (1-4 Hz) oscillations of perigeniculate thalamic neurons and their modulation by light. Neuroscience. 1992 Nov;51(2):285–294. doi: 10.1016/0306-4522(92)90315-s. [DOI] [PubMed] [Google Scholar]
- Baskys A. Metabotropic receptors and 'slow' excitatory actions of glutamate agonists in the hippocampus. Trends Neurosci. 1992 Mar;15(3):92–96. doi: 10.1016/0166-2236(92)90018-4. [DOI] [PubMed] [Google Scholar]
- Budde Thomas, Mager Ralph, Pape Hans-Christian. Different Types of Potassium Outward Current in Relay Neurons Acutely Isolated from the Rat Lateral Geniculate Nucleus. Eur J Neurosci. 1992;4(8):708–722. doi: 10.1111/j.1460-9568.1992.tb00180.x. [DOI] [PubMed] [Google Scholar]
- Burke W., Jervie Sefton A. Discharge patterns of principal cells and interneurones in lateral geniculate nucleus of rat. J Physiol. 1966 Nov;187(1):201–212. doi: 10.1113/jphysiol.1966.sp008083. [DOI] [PMC free article] [PubMed] [Google Scholar]
- Connor J. A., Stevens C. F. Voltage clamp studies of a transient outward membrane current in gastropod neural somata. J Physiol. 1971 Feb;213(1):21–30. doi: 10.1113/jphysiol.1971.sp009365. [DOI] [PMC free article] [PubMed] [Google Scholar]
- Coulter D. A., Huguenard J. R., Prince D. A. Calcium currents in rat thalamocortical relay neurones: kinetic properties of the transient, low-threshold current. J Physiol. 1989 Jul;414:587–604. doi: 10.1113/jphysiol.1989.sp017705. [DOI] [PMC free article] [PubMed] [Google Scholar]
- Crunelli V., Kelly J. S., Leresche N., Pirchio M. The ventral and dorsal lateral geniculate nucleus of the rat: intracellular recordings in vitro. J Physiol. 1987 Mar;384:587–601. doi: 10.1113/jphysiol.1987.sp016471. [DOI] [PMC free article] [PubMed] [Google Scholar]
- Crunelli V., Leresche N. A role for GABAB receptors in excitation and inhibition of thalamocortical cells. Trends Neurosci. 1991 Jan;14(1):16–21. doi: 10.1016/0166-2236(91)90178-w. [DOI] [PubMed] [Google Scholar]
- Crunelli V., Lightowler S., Pollard C. E. A T-type Ca2+ current underlies low-threshold Ca2+ potentials in cells of the cat and rat lateral geniculate nucleus. J Physiol. 1989 Jun;413:543–561. doi: 10.1113/jphysiol.1989.sp017668. [DOI] [PMC free article] [PubMed] [Google Scholar]
- Dossi R. C., Nuñez A., Steriade M. Electrophysiology of a slow (0.5-4 Hz) intrinsic oscillation of cat thalamocortical neurones in vivo. J Physiol. 1992 Feb;447:215–234. doi: 10.1113/jphysiol.1992.sp018999. [DOI] [PMC free article] [PubMed] [Google Scholar]
- Dubin M. W., Cleland B. G. Organization of visual inputs to interneurons of lateral geniculate nucleus of the cat. J Neurophysiol. 1977 Mar;40(2):410–427. doi: 10.1152/jn.1977.40.2.410. [DOI] [PubMed] [Google Scholar]
- Fitzpatrick D., Penny G. R., Schmechel D. E. Glutamic acid decarboxylase-immunoreactive neurons and terminals in the lateral geniculate nucleus of the cat. J Neurosci. 1984 Jul;4(7):1809–1829. doi: 10.1523/JNEUROSCI.04-07-01809.1984. [DOI] [PMC free article] [PubMed] [Google Scholar]
- Gabbott P. L., Somogyi J., Stewart M. G., Hámori J. GABA-immunoreactive neurons in the dorsal lateral geniculate nucleus of the rat: characterisation by combined Golgi-impregnation and immunocytochemistry. Exp Brain Res. 1986;61(2):311–322. doi: 10.1007/BF00239521. [DOI] [PubMed] [Google Scholar]
- Gerber U., Greene R. W., McCarley R. W. Repetitive firing properties of medial pontine reticular formation neurones of the rat recorded in vitro. J Physiol. 1989 Mar;410:533–560. doi: 10.1113/jphysiol.1989.sp017548. [DOI] [PMC free article] [PubMed] [Google Scholar]
- Grossman A., Lieberman A. R., Webster K. E. A Golgi study of the rat dorsal lateral geniculate nucleus. J Comp Neurol. 1973 Aug;150(4):441–466. doi: 10.1002/cne.901500404. [DOI] [PubMed] [Google Scholar]
- Guillery R. W. A study of Golgi preparations from the dorsal lateral geniculate nucleus of the adult cat. J Comp Neurol. 1966 Sep;128(1):21–50. doi: 10.1002/cne.901280104. [DOI] [PubMed] [Google Scholar]
- Hamill O. P., Marty A., Neher E., Sakmann B., Sigworth F. J. Improved patch-clamp techniques for high-resolution current recording from cells and cell-free membrane patches. Pflugers Arch. 1981 Aug;391(2):85–100. doi: 10.1007/BF00656997. [DOI] [PubMed] [Google Scholar]
- Hernández-Cruz A., Pape H. C. Identification of two calcium currents in acutely dissociated neurons from the rat lateral geniculate nucleus. J Neurophysiol. 1989 Jun;61(6):1270–1283. doi: 10.1152/jn.1989.61.6.1270. [DOI] [PubMed] [Google Scholar]
- Hosford D. A., Clark S., Cao Z., Wilson W. A., Jr, Lin F. H., Morrisett R. A., Huin A. The role of GABAB receptor activation in absence seizures of lethargic (lh/lh) mice. Science. 1992 Jul 17;257(5068):398–401. doi: 10.1126/science.1321503. [DOI] [PubMed] [Google Scholar]
- Huguenard J. R., Coulter D. A., Prince D. A. A fast transient potassium current in thalamic relay neurons: kinetics of activation and inactivation. J Neurophysiol. 1991 Oct;66(4):1304–1315. doi: 10.1152/jn.1991.66.4.1304. [DOI] [PubMed] [Google Scholar]
- Huguenard J. R., Prince D. A. A novel T-type current underlies prolonged Ca(2+)-dependent burst firing in GABAergic neurons of rat thalamic reticular nucleus. J Neurosci. 1992 Oct;12(10):3804–3817. doi: 10.1523/JNEUROSCI.12-10-03804.1992. [DOI] [PMC free article] [PubMed] [Google Scholar]
- Huguenard J. R., Prince D. A. Slow inactivation of a TEA-sensitive K current in acutely isolated rat thalamic relay neurons. J Neurophysiol. 1991 Oct;66(4):1316–1328. doi: 10.1152/jn.1991.66.4.1316. [DOI] [PubMed] [Google Scholar]
- Jahnsen H., Llinás R. Electrophysiological properties of guinea-pig thalamic neurones: an in vitro study. J Physiol. 1984 Apr;349:205–226. doi: 10.1113/jphysiol.1984.sp015153. [DOI] [PMC free article] [PubMed] [Google Scholar]
- Jahnsen H., Llinás R. Ionic basis for the electro-responsiveness and oscillatory properties of guinea-pig thalamic neurones in vitro. J Physiol. 1984 Apr;349:227–247. doi: 10.1113/jphysiol.1984.sp015154. [DOI] [PMC free article] [PubMed] [Google Scholar]
- Kang Y., Kitai S. T. Electrophysiological properties of pedunculopontine neurons and their postsynaptic responses following stimulation of substantia nigra reticulata. Brain Res. 1990 Dec 3;535(1):79–95. doi: 10.1016/0006-8993(90)91826-3. [DOI] [PubMed] [Google Scholar]
- Kay A. R., Wong R. K. Isolation of neurons suitable for patch-clamping from adult mammalian central nervous systems. J Neurosci Methods. 1986 May;16(3):227–238. doi: 10.1016/0165-0270(86)90040-3. [DOI] [PubMed] [Google Scholar]
- Liu Z., Vergnes M., Depaulis A., Marescaux C. Evidence for a critical role of GABAergic transmission within the thalamus in the genesis and control of absence seizures in the rat. Brain Res. 1991 Apr 5;545(1-2):1–7. doi: 10.1016/0006-8993(91)91262-y. [DOI] [PubMed] [Google Scholar]
- McCobb D. P., Beam K. G. Action potential waveform voltage-clamp commands reveal striking differences in calcium entry via low and high voltage-activated calcium channels. Neuron. 1991 Jul;7(1):119–127. doi: 10.1016/0896-6273(91)90080-j. [DOI] [PubMed] [Google Scholar]
- McCormick D. A. Functional properties of a slowly inactivating potassium current in guinea pig dorsal lateral geniculate relay neurons. J Neurophysiol. 1991 Oct;66(4):1176–1189. doi: 10.1152/jn.1991.66.4.1176. [DOI] [PubMed] [Google Scholar]
- McCormick D. A. Neurotransmitter actions in the thalamus and cerebral cortex and their role in neuromodulation of thalamocortical activity. Prog Neurobiol. 1992 Oct;39(4):337–388. doi: 10.1016/0301-0082(92)90012-4. [DOI] [PubMed] [Google Scholar]
- McCormick D. A., Pape H. C. Acetylcholine inhibits identified interneurons in the cat lateral geniculate nucleus. Nature. 1988 Jul 21;334(6179):246–248. doi: 10.1038/334246a0. [DOI] [PubMed] [Google Scholar]
- McCormick D. A., Pape H. C. Properties of a hyperpolarization-activated cation current and its role in rhythmic oscillation in thalamic relay neurones. J Physiol. 1990 Dec;431:291–318. doi: 10.1113/jphysiol.1990.sp018331. [DOI] [PMC free article] [PubMed] [Google Scholar]
- McCormick D. A., von Krosigk M. Corticothalamic activation modulates thalamic firing through glutamate "metabotropic" receptors. Proc Natl Acad Sci U S A. 1992 Apr 1;89(7):2774–2778. doi: 10.1073/pnas.89.7.2774. [DOI] [PMC free article] [PubMed] [Google Scholar]
- Miles R., Poncer J. C. Metabotropic glutamate receptors mediate a post-tetanic excitation of guinea-pig hippocampal inhibitory neurones. J Physiol. 1993 Apr;463:461–473. doi: 10.1113/jphysiol.1993.sp019605. [DOI] [PMC free article] [PubMed] [Google Scholar]
- Montero V. M., Singer W. Ultrastructural identification of somata and neural processes immunoreactive to antibodies against glutamic acid decarboxylase (GAD) in the dorsal lateral geniculate nucleus of the cat. Exp Brain Res. 1985;59(1):151–165. doi: 10.1007/BF00237675. [DOI] [PubMed] [Google Scholar]
- Numann R. E., Wadman W. J., Wong R. K. Outward currents of single hippocampal cells obtained from the adult guinea-pig. J Physiol. 1987 Dec;393:331–353. doi: 10.1113/jphysiol.1987.sp016826. [DOI] [PMC free article] [PubMed] [Google Scholar]
- Nuñez A., Curró Dossi R., Contreras D., Steriade M. Intracellular evidence for incompatibility between spindle and delta oscillations in thalamocortical neurons of cat. Neuroscience. 1992;48(1):75–85. doi: 10.1016/0306-4522(92)90339-4. [DOI] [PubMed] [Google Scholar]
- Ohara P. T., Lieberman A. R., Hunt S. P., Wu J. Y. Neural elements containing glutamic acid decarboxylase (GAD) in the dorsal lateral geniculate nucleus of the rat; immunohistochemical studies by light and electron microscopy. Neuroscience. 1983;8(2):189–211. doi: 10.1016/0306-4522(83)90060-x. [DOI] [PubMed] [Google Scholar]
- Rudy B. Diversity and ubiquity of K channels. Neuroscience. 1988 Jun;25(3):729–749. doi: 10.1016/0306-4522(88)90033-4. [DOI] [PubMed] [Google Scholar]
- Schwindt P. C., Spain W. J., Foehring R. C., Stafstrom C. E., Chubb M. C., Crill W. E. Multiple potassium conductances and their functions in neurons from cat sensorimotor cortex in vitro. J Neurophysiol. 1988 Feb;59(2):424–449. doi: 10.1152/jn.1988.59.2.424. [DOI] [PubMed] [Google Scholar]
- Soltesz I., Crunelli V. A role for low-frequency, rhythmic synaptic potentials in the synchronization of cat thalamocortical cells. J Physiol. 1992 Nov;457:257–276. doi: 10.1113/jphysiol.1992.sp019377. [DOI] [PMC free article] [PubMed] [Google Scholar]
- Soltesz I., Lightowler S., Leresche N., Jassik-Gerschenfeld D., Pollard C. E., Crunelli V. Two inward currents and the transformation of low-frequency oscillations of rat and cat thalamocortical cells. J Physiol. 1991 Sep;441:175–197. doi: 10.1113/jphysiol.1991.sp018745. [DOI] [PMC free article] [PubMed] [Google Scholar]
- Steriade M., Deschenes M. The thalamus as a neuronal oscillator. Brain Res. 1984 Nov;320(1):1–63. doi: 10.1016/0165-0173(84)90017-1. [DOI] [PubMed] [Google Scholar]
- Steriade M., Deschênes M., Domich L., Mulle C. Abolition of spindle oscillations in thalamic neurons disconnected from nucleus reticularis thalami. J Neurophysiol. 1985 Dec;54(6):1473–1497. doi: 10.1152/jn.1985.54.6.1473. [DOI] [PubMed] [Google Scholar]
- Steriade M., Dossi R. C., Nuñez A. Network modulation of a slow intrinsic oscillation of cat thalamocortical neurons implicated in sleep delta waves: cortically induced synchronization and brainstem cholinergic suppression. J Neurosci. 1991 Oct;11(10):3200–3217. doi: 10.1523/JNEUROSCI.11-10-03200.1991. [DOI] [PMC free article] [PubMed] [Google Scholar]
- Sterling P., Davis T. L. Neurons in cat lateral geniculate nucleus that concentrate exogenous [3H]-gamma-aminobutyric acid (GABA). J Comp Neurol. 1980 Aug 15;192(4):737–749. doi: 10.1002/cne.901920408. [DOI] [PubMed] [Google Scholar]
- Suzuki S., Rogawski M. A. T-type calcium channels mediate the transition between tonic and phasic firing in thalamic neurons. Proc Natl Acad Sci U S A. 1989 Sep;86(18):7228–7232. doi: 10.1073/pnas.86.18.7228. [DOI] [PMC free article] [PubMed] [Google Scholar]
- Weber A. J., Kalil R. E., Behan M. Synaptic connections between corticogeniculate axons and interneurons in the dorsal lateral geniculate nucleus of the cat. J Comp Neurol. 1989 Nov 1;289(1):156–164. doi: 10.1002/cne.902890113. [DOI] [PubMed] [Google Scholar]
- von Krosigk M., Bal T., McCormick D. A. Cellular mechanisms of a synchronized oscillation in the thalamus. Science. 1993 Jul 16;261(5119):361–364. doi: 10.1126/science.8392750. [DOI] [PubMed] [Google Scholar]